7 Properties of Bone Cements and Vertebral Fill Materials: Implications for Clinical Use in Image-Guided Therapy and Vertebral Augmentation
Summary
Bone cement or polymethyl methacrylate (PMMA) is the primary stabilizing material used for treating painful vertebral compression fractures (VCFs). The PMMAs available are acrylic polymers comprised of different components that can be manipulated to maximize its utility in minimally invasive spine interventions. In addition to PMMA, there is also another cement type comprised of self-hardening calcium phosphate cements (CPCs) that have been used to treat VCFs. Unlike PMMA that hardens by a polymerization process converting methylmethacrylate (MMA) monomers to PMMA, CPCs dissolve with water into a paste that hardens at body temperature into hydroxyapatite (HA). The advantage of CPCs are that they are biocompatible and are resorbed over time but the disadvantages compared to PMMA is that they are difficult to inject, are weaker than PMMA, and are often too brittle to be used in high stress areas. Fill materials also include a glass ceramic tri-resin polymer that is hydrophilic, has lower exothermic temperatures, and mimics the mechanical properties of bone. While the hydrophilic properties result in a greater amount of interdigitation into the bone and less material injected, it tends to extravasate more easily than the hydrophobic cements. Cement properties can be tailored to the appropriate use by varying the cement ingredients and can have other added components such as antibiotics and other radiopacifying agents. The future of bone cements is likely with the addition of bioactive materials such as bioactive glass, bone morphogenic protein, platelet rich plasma, stem cells, and various growth factors to promote the osteogenic activity of the native bone and to improve the strength of the cement and its degree of bony incorporation.
7.1 Introduction
Advances in image-guided therapy for vertebral fractures and other bone-related disorders have made acrylic bone cement an integral part of the interventional tool kit. This chapter focuses on the chemistry of bone cement polymerization and the properties of components in PMMA based polymers, the most commonly used bone cements in interventional procedures such as percutaneous vertebroplasty (PVP) or vertebral augmentation. The effects of altering the concentration of the components such as MMA monomers, PMMA beads, benzoyl peroxide (BPO) activator, N,N-dimethyl-p-toluidine (DMPT) initiator, and radiopacifiers on the setting time, viscosity, polymerization temperature, and compressive strength of the cement are also considered. This information allows us to manipulate bone cement characteristics for specific applications and maximize the clinical potential of image-guided interventions. Most commercial cement kits used by radiologists were originally designed for joint replacement surgery, not vertebral fracture therapy. Novel applications such as VP require modifications of standard bone–cement mixtures—adjustments that can be made only by understanding the underlying chemical and material properties of these compounds. Our discussion will focus on the theoretical and practical aspects of polymerization chemistry, the basis for most bone cement solidification.
7.2 Bone Cement Types
There are two main types of bone cements: polymers and self-hardening CPCs. Both types are fillers that stabilize joint implants or fractures, but neither is adhesive. Polymers are most commonly used because of their proven track record of safety and efficacy. They are based on the polymerization of MMA monomers to polymethyl methacrylate (PMMA), a compound better known by the name Plexiglas. Unlike polymers, the first CPCs were developed in the late 1980s to remineralize early dental carries and were approved by the Food and Drug Administration for repairing cranial defects in 1996 as cavity void fillers.
The CPCs are potentially an ideal filling material for VP and balloon kyphoplasty (BKP) applications. CPCs function by a completely different chemical mechanism based on the solubility of reactants and products. Despite the large number of calcium phosphate (CaP) combinations in different CPC systems, the setting chemistry is similar and involves dissolution and reprecipitation. Based on the nature of the end hydration product, which depends on the pH of the cement paste, Ca PO4 cements can be divided into two categories: apatite (HA) or calcium-deficient hydroxyapatite [CDHA], formed at pH > 4.2) and brushite (dicalcium phosphate dehydrate [DCPD]). Due to the short setting time, low mechanical strength, and inferior injection characteristics, brushite cements only have limited clinical applications. When tetracalcium phosphate and dicalcium phosphate are mixed with water into a paste, HA (the major mineral found in normal teeth) is rapidly formed and precipitates out of the solution, forming a hard mass at body temperature. 1 , 2 HA is a biocompatible material believed to be gradually replaced in vivo by new bone with no loss of volume. 3 , 4 On the downside, the cement is difficult to inject against high backpressure, and the bone resorption properties may actually cause long-term weakening in patients with osteoporosis. Additionally, it may be weaker than polymer cement 5 and too brittle to be used for load-bearing applications. Because there are no long-term data for CPC technology, this review will focus on PMMA bone cements.
The use of HA and CaP bone cements in an osteoporotic patient without the addition of anabolic parathyroid (PTH) analog medication is probably not a sound concept. The addition of systemic PTH allows bone activation and anabolic bone stimulation to integrate these cements in a way that the inert elderly osteoporotic cannot achieve otherwise.
Cortoss is a glass ceramic combeite tri-resin polymer, an injectable, synthetic, nonresorbable biomaterial that mimics the mechanical properties of cortical bone. 1 Cortoss has been clinically proven to match the safety and effectiveness of PMMA for VP and clinical trials with Cortoss report a low incidence of adjacent fractures, 2 lower exothermic temperatures, and less monomer release than PMMA. The safety and efficacy of Cortoss has been demonstrated in three U.S. clinical investigations and multiple European studies. 6 , 7 In patients with a first-time fracture at one level, there was a 43% reduction in adjacent-level fractures in the patient population that used Cortoss. 6 Compared to PMMA, Cortoss is more hydrophilic, which enables it to coat and augment the internal structure of the vertebral body. This interdigitating characteristic resulted in a 30% reduction in material injected when compared to PMMA in a controlled study. Despite the optimal characteristics allowing the interdigitation of Cortoss within the cancellous vertebral body bone, the hydrophilic properties of the fill material make it prone to extravasation outside the vertebral body.
7.2.1 Polymer Chemistry
Before the specifics of bone cement are discussed, we will briefly review polymer chemistry. Polymers are large molecules composed of individual repeating units (monomers). Proteins are classic examples of biologic polymers composed of smaller amino acid units.
Polymerization can occur by two different mechanisms: (1) in condensation reactions, a functional group on the monomer reacts with a functional group on the growing end of the polymer, lengthening the chain and releasing an extraneous molecule (▶Fig. 7.1) 8 and (2) in addition reactions, the polymer chain grows by reacting directly with the double bond of a monomer and no extraneous molecules are created (▶Fig. 7.2).


Polymerization begins by the addition mechanism in which a monomer becomes unstable by reacting with an initiator, a volatile molecule that is most commonly a radical (molecules that contain a single unpaired electron). Radicals bond with monomers, forming monomer radicals that can attack the double bond of the next monomer by the mechanism shown in ▶Fig. 7.2, propagating the polymer chain.
Because radicals are so transient, initiators are often added in the form of an unreactive peroxide form that is stable in solution. Radicals are formed when heat or light cleaves the peroxide molecule. For applications in which high temperatures are not practical (such as the use of bone cement in vivo), peroxide is cleaved by adding a chemical activator such as N, N-dimethyl-p-toluidine (DMPT; ▶Fig. 7.3). 9

7.2.2 PMMA-Based Bone Cements
Bone cements polymerize by radical-initiated addition reactions. Most commercially available cements have two separate components (▶Table 7.1): a powder containing prepolymerized beads of PMMA (or PMMA/styrene copolymer) and a liquid containing MMA monomer. The BPO initiator is incorporated into the powder and the chemical activator (i.e., DMPT) is incorporated into the liquid, so peroxide cleavage and polymerization begin only when the two are mixed. To prevent spontaneous polymerization during storage, the easily oxidized molecule hydroquinone is also added to the liquid. The DMPT cleaves BPO initiator at room temperature, forming a phenyl radical (▶Fig. 7.3) that attacks the double bond of the MMA monomer by the mechanism shown in ▶Fig. 7.2. The growing polymer chains encapsulate the PMMA beads within a solid matrix.
Powder |
|
Liquid |
|
Abbreviations: DMPT, N,N-dimethyl-p-toluidine; MMA, methylmethacrylate; PMMA, polymethyl methacrylate. |
Cement is made radiopaque by adding barium sulfate or zirconium dioxide compounds to the powder (▶Table 7.2).
7.3 Cement Properties
To design cements with predictable intraoperative and postoperative behavior, we must understand how cement formulation affects the polymerization and material properties of the solid. Like any heterogeneous mixture, the characteristics of the whole differ markedly from those of its components. For example, one cannot calculate a priori the porosity and strength of building mortar based solely on the properties of its various pebbles and finely ground minerals. These individual particles form a complex intermolecular network whose characteristics can be identified only empirically. The effect of each component on the setting time, polymerization temperature, and material properties of bone cement is detailed in the following sections.
7.3.1 Liquid-to-Powder Ratio
Polymerization temperature and setting time can be studied by altering liquid-to-powder (L/P) ratios. Clinically, these are key properties to control. Predictable setting time is critical intraoperatively to prevent solidification before completion of the procedure. High polymerization temperatures are known to cause tissue necrosis and hinder the efficacy of joint prostheses. 1 In spite of adverse effects of cement heat in some situations, highly exothermic cements can be preferentially used in a patient with malignant disease and pathologic compression fractures to achieve local tumor kill. Exofix is a cement that polymerizes to 88°C and was designed specifically for this purpose. Early research showed that greater L/P ratios produce higher peak temperatures but increase setting time. One hypothesis was that, at high L/P ratios, an abundance of monomers react exothermically, increasing the peak polymerization temperature. However, the high L/P ratio also decreases the relative concentration of the initiator (a component of the powder), so the monomers are activated slowly, increasing setting time. 10 , 11 Clearly, studies that vary monomer-topolymer ratios independently of other cement components are needed (▶Table 7.3).
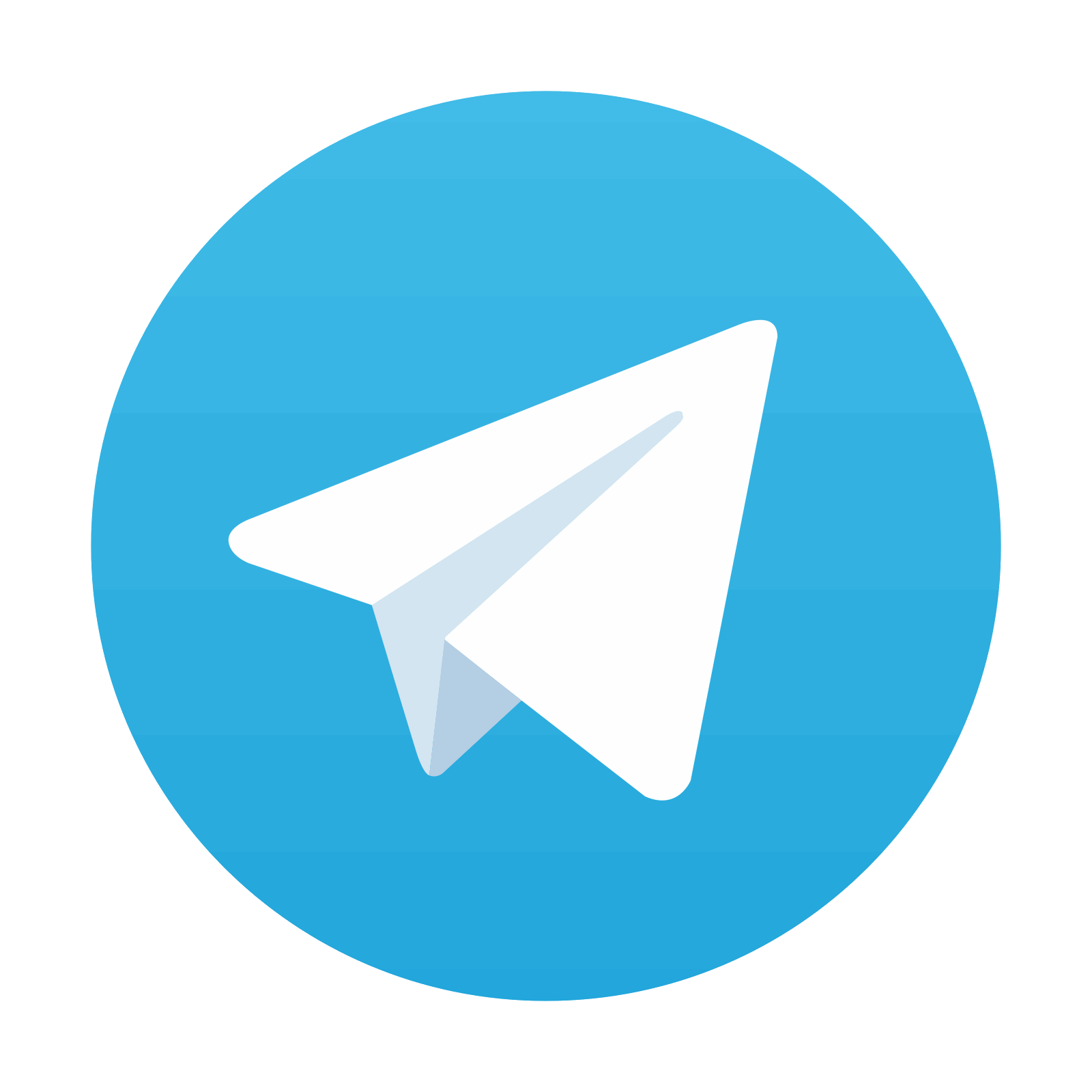
Stay updated, free articles. Join our Telegram channel

Full access? Get Clinical Tree
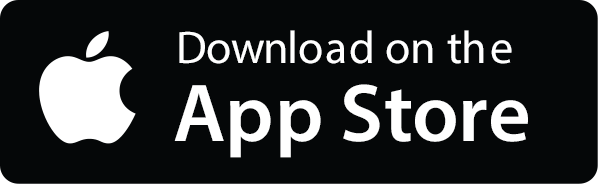
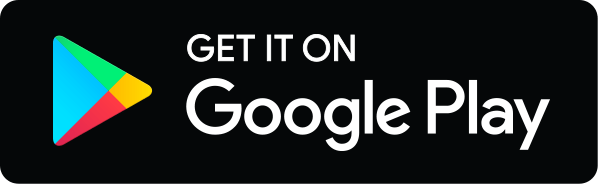
