7 Types of Hydrocephalus

7.1 Introduction
Hydrocephalus is one of the oldest neurologic pathologies recorded in human history, with multiple descriptions by Galen and Hippocrates.1 Despite the passage of more than two millennia from these early accounts, hydrocephalus remains a common, enigmatic, and challenging entity to treat. Most modern surgical management strategies for obstructive and nonobstructive hydrocephalus have followed evolving cerebrospinal fluid (CSF) shunting strategies. However, recent advances in our understanding of hydrodynamic dysfunctions underlying hydrocephalus have spurred intervention strategies that focus on restoring normal physiologic CSF circulation rather than on external drainage. Neuroendoscopy, a vital tool in the minimally invasive paradigm, affords maximum access to the ventricular system. This chapter briefly describes the different types of hydrocephalus, their pathophysiologic and hydrodynamic bases, and the implications of endoscopic intervention.
7.2 Pathophysiology
7.2.1 Obstructive Hydrocephalus
The traditional definition of obstructive hydrocephalus stems from work in 1914 by Dandy and Blackfan.2 It refers to obstruction of bulk CSF flow, leading to dilatation and isolation of the proximal ventricular system from the subarachnoid space. Obstructive hydrocephalus includes forms of hydrocephalus in which a physical obstruction within the ventricular system, or its outlet from the brain, impedes CSF flow. The level of the obstruction can vary, and it can occur over the entire span of the ventricular system, from the foramen of Monro to the third ventricle, the cerebral aqueduct, the fourth ventricle, and its outlets. Notable common etiologies of obstructive hydrocephalus include congenital or acquired stenosis of the foramen of Monro or the aqueduct, obstruction by parasites causing conditions such as neurocysticercosis, or tumors and arachnoid cysts that cause compression due to direct mass effect (Fig. 7.1, Video 7.1 and Video 7.2).

7.2.2 Communicating Hydrocephalus
Communicating hydrocephalus, also known as non-obstructive hydrocephalus, has been described in terms of CSF malabsorption resulting from a diverse list of possible etiologies. This type of hydrocephalus encompasses all cases in which the flow of CSF is obstructed at a point distal to the outlet of CSF from the brain (i.e., the foramina of Luschka and Magendie). This can occur within the subarachnoid space or at the level of the arachnoid granulations. Common causes of communicating hydrocephalus include infections, intracranial hemorrhage, and trauma that lead to scarring and adhesions within the subarachnoid space. Equally common are idiopathic and congenital cases—usually presenting in the pediatric population—and normal pressure hydrocephalus, which occurs in the aging adult. However, the differentiation between obstructive and communicating hydrocephalus is somewhat arbitrary. All forms of hydrocephalus involve an obstructive element, either physical within the ventricular system (classic obstructive hydrocephalus) or functional within the arachnoid villi or subarachnoid space (communicating hydrocephalus).
A new model of CSF hydrodynamics has emerged within the past 15 years that pinpoints decreased brain compliance as a unifying pathophysiology for communicating hydrocephalus, regardless of its etiology.3, 4 Multiple studies have examined pulse pressure transmission and CSF flow states in patients with chronic communicating hydrocephalus, and brain compliance is found to be nearly one order of magnitude lower in these patients.5,6,7 This diminished state of brain compliance increases the pulse pressure transmitted from the arterial system to the brain, consequently raising transmitted pulse pressure through the brain and ventricles. This compresses cerebral vessels and further hinders circulatory absorption of CSF (Fig. 7.2). Therefore, the central pathophysiology underpinning communicating hydrocephalus may be alterations in the ventricular and parenchymal compliance, distorting pulse, and perfusion-dependent circulation of CSF.

7.2.3 Extraventricular-Intracisternal Obstructive Hydrocephalus
The type of hydrocephalus known as extraventricular-intracisternal obstructive hydrocephalus results from obstruction of the basal cisterns. The basal subarachnoid spaces play an important role in the circulation of CSF, and they are also vulnerable to insult.7,8,9 Subarachnoid hemorrhage, trauma, and infection (e.g., meningitis or arachnoiditis) can cause basal subarachnoid inflammation, adhesions, and scars that reduce intracranial compliance by different mechanisms.7,10 These adhesions can also cause obstruction of the basal cisterns. Experimental models have shown that adhesions of the basal subarachnoid spaces have a stronger effect than an intraventricular obstruction on the development of hydrocephalus.11 Additional studies have indicated that any process that interferes with the expansion of the arteries in the basal subarachnoid space may cause hydrocephalus.7,8,12
7.2.4 Multiloculated Hydrocephalus
Neoepithelial loculations that develop in patients with hydrocephalus typically occur after infection or hemorrhagic events and result in multicompartmentalized CSF spaces. In 1973, Schultz and Leeds13 described the etiology of these septations as originating from glial tufts that protruded through eroded areas of ependyma after infectious meningitis (Fig. 7.3 a,b). The inciting and perpetuating offender appears to be a continuous inflammatory response to debris created after the initial insult, as well as continued disruption of the membranes that arise during interventions or shunt overdrainage.14 Other causative and continuous biological mechanisms of multiloculated hydrocephalus remain unknown.

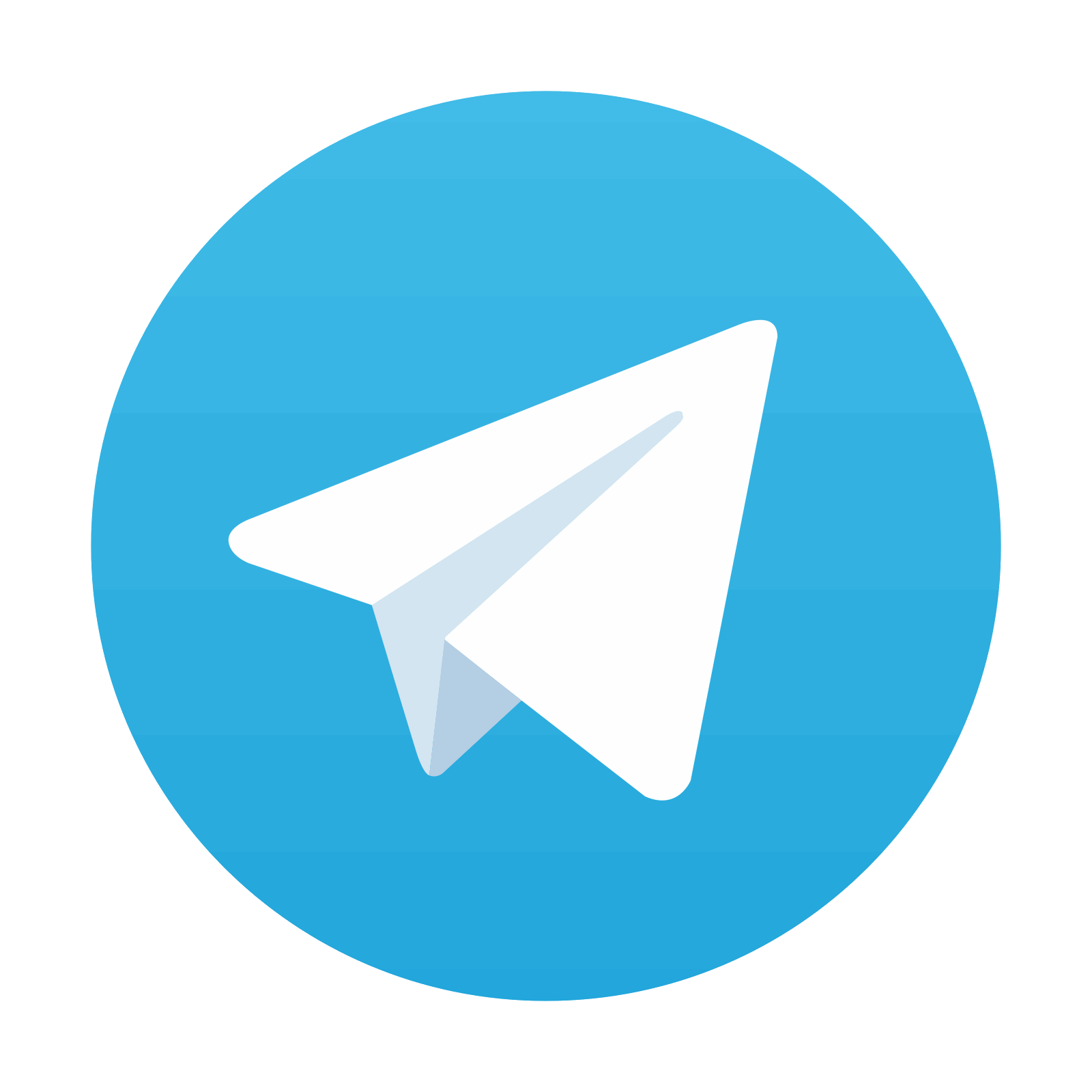
Stay updated, free articles. Join our Telegram channel

Full access? Get Clinical Tree
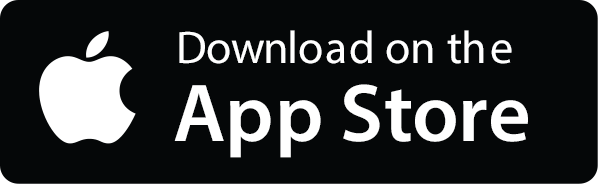
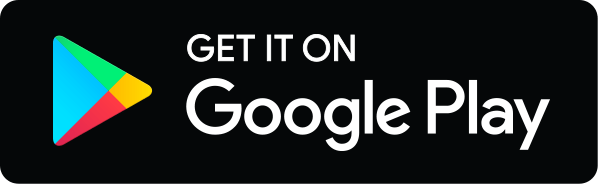
