8 Diagnostic Imaging of Sporadic Vestibular Schwannoma and Other CPA Lesions
8.1 Imaging Technique
Imaging of the internal auditory canal (IAC), cerebellopontine angle (CPA), and labyrinthine structures is most often performed as a screening study to exclude vestibular schwannoma (VS) in the patient presenting with asymmetric sensorineural hearing loss (SNHL) or as part of a “watch and wait” strategy in following up patients with previously diagnosed schwannomas. Magnetic resonance (MR) has replaced computed tomography (CT) as the primary imaging modality of choice. Diagnostic examinations can be obtained at either 3T or 1.5T. The higher field strength available at 3T offers the ability to achieve higher resolution images at shorter acquisition times. Disadvantages of 3T including increased susceptibility artifact at air–bone interfaces and increased banding artifact with use of 3D gradient echo (GRE) sequences (Fast Imaging Employing Steady-state Acquisition [FIESTA], Constructive Interference in the Steady State [CISS]) should be considered when deciding between these two commonly available MR platforms. The current preference at our institution is to scan at 3T using imaging sequences that eliminate or significantly reduce higher field strength artifacts (e.g., replacing the more commonly employed 3D GRE sequence with 3D fast spin echo [FSE] sequence). Improved image resolution can also be maximized with the use of multichannel coils, such as a 32- or 64-channel head coil depending on the available 3T platform.
Selection of imaging sequences is critical in achieving maximal image resolution. At the author’s center, the current protocol utilizes higher resolution submillimeter 3D acquisitions with isotropic voxel sizes (Table 8‑1). These sequences permit multiplanar reconstructions (Fig. 8‑1 A–F), replacing the previously employed 3- to 4-mm 2D sequences that required separate planes of acquisition.

T2-weighted MR cisternography performed at small fields of view (FOV) to include 3D FSE T2 or 3D GRE (or T2*) acquisitions have become the standard of care when imaging the IAC. At 3T, 3D FSE offers distinct advantages over 3D GRE. Specifically, 3D FSE produces less susceptibility artifact at the skull base compared to 3D GRE and has the added advantage of eliminating problems with banding artifact inherent to 3D GRE imagings. Literatur (Fig. 8‑2 ). Image blurring, a consequence of the long-echo trains employed with high-resolution 3D FSE techniques, can be minimized by employing variable flip angle acquisitions.s. Literatur Our protocol also includes a 3D fluid-attenuated inversion recovery (FLAIR) sequence to assess for any change in labyrinthine fluid signal not detectible on the T2 cisternogram sequences (Fig. 8‑1 D).

To date, utilization of T1-weighted 3D sequences when imaging the IAC has not been as widely accepted compared to their 2D counterparts but are gaining in popularity because of the superior image resolution and multiplanar reformatting capability available with 3D acquisitions. 3D T1-weighted images can be produced using FSE or GRE sequencing. T1-weighted 3D GRE images differ slightly in appearance from T1-weighted 3D FSE in that flow-related enhancement is noted within the major vascular structures (i.e., adjacent internal carotid arteries) on 3D GRE (Fig. 8‑3 A). 3D T1 FSE also provides better resolution of the fluid-filled labyrinthine structures against the background of dense labyrinthine bone than 3D T1 GRE (Fig. 8‑3 B).

All 3D sequences, if acquired at isometric voxel size, allow for multiplanar reconstructions obviating the need for additional planes of acquisition. 3D sequences are more sensitive to motion artifact due to longer imaging times when compared to 2D sequences and therefore the patient’s ability to remain still during image acquisition needs to be taken into account when protocoling the study.
The use of contrast in the imaging evaluation of VS has been considered standard practice and continues to be part of the routine examination in most centers. We routinely employ fat saturation on the postcontrast sequences to increase the conspicuity of pathologic contrast enhancement, particularly following fat graft placement, and eliminate the possibility of confusing enhancement with hyperintense marrow fat around the porus acusticus and within the petrous apex. Because of the low diagnostic yield of adding contrast to the screening exam, some authors have advocated the use of noncontrast MR examinations, in an effort to reduce scan time and cost.s. Literatur , s. Literatur Recent studies have demonstrated that certain gadolinium chelate products are deposited in body tissues.s. Literatur , s. Literatur , s. Literatur As a result of these studies and those demonstrating the low diagnostic yield of adding contrast to the examination, we may anticipate a future demand for noncontrast MR evaluations at least until more pharmacologically stable contrast agents become available. MR angiography and MR venography can be added to the imaging protocol to exclude vascular lesions of the IAC and CPA.
8.2 Anatomic Variants
Variations in the anatomy of the temporal bone may have important implications for avoiding surgical complications during VS resection. Most of these variants are best delineated with high-resolution multidetector or cone beam CT. Vascular variants would include anterior sigmoid sinus, high jugular bulb, unusual emissary veins of the temporal bone, and aberrant or lateralized internal carotid artery (Fig. 8‑4 ). An anterior sigmoid sinus can be recognized when this structure is anteriorly displaced into the mastoid cavity, thereby restricting transmastoid access even with extensive decompression and placing it at risk for injury.s. Literatur Variable pneumatization of the petrous portion of the temporal bone to include the margins of the IAC may predispose to postoperative cerebrospinal fluid (CSF) leaks if care is not taken to recognize these variants preoperatively and carefully seal off these air cells during surgery. Retained secretion within these air cells may produce the appearance of enhancing “pseudolesions” on MR and, if necessary, may be confirmed with temporal bone CT (Fig. 8‑5 ).


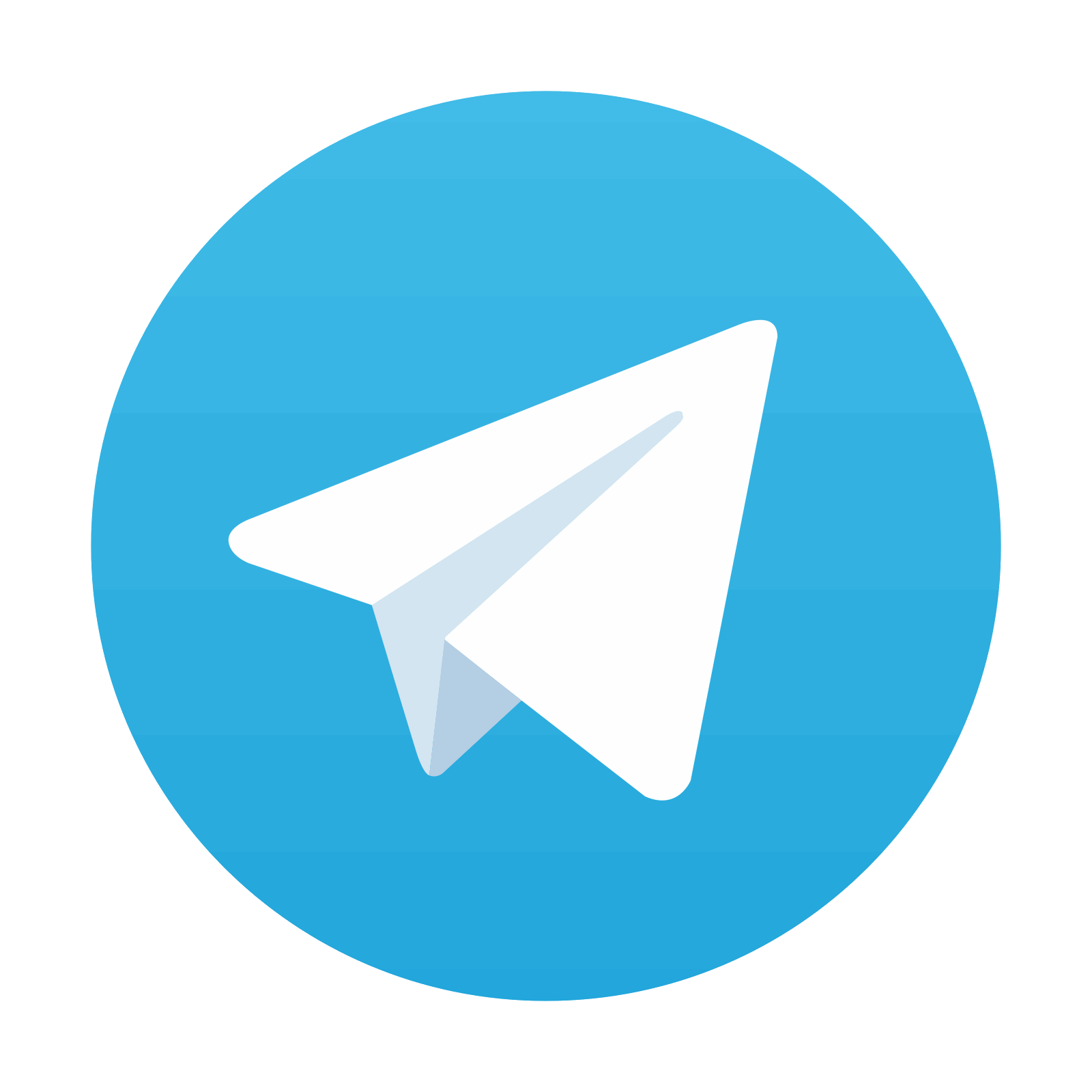
Stay updated, free articles. Join our Telegram channel

Full access? Get Clinical Tree
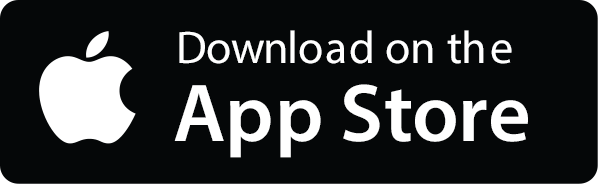
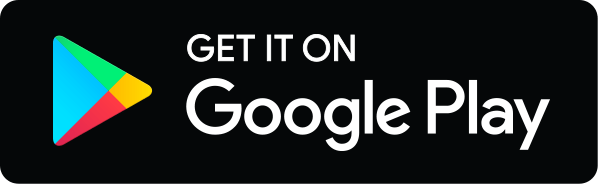