8 Neural Element Injury
8.1 Mechanisms of Neural Element Injury
External influences can cause a cell to become dysfunctional, or to die, by one or a combination of three mechanisms: (1) cell disruption, (2) cell distortion, and (3) metabolic derangements. Disruption of the cell usually results in its death. Cell distortion and metabolic derangements can cause temporary dysfunction or death of the cell. Cell disruption can result from the initial (primary) injury or can be secondary to the exaggeration of cell distortion that can result from delayed central nervous system tissue shifts, such as those related to edema or hematoma formation (ongoing primary injury). Cell disruption (death) can also be caused by metabolic derangements, such as extracellular osmotic shifts; self-destructive processes that can follow the primary injury; and apoptosis. 1 This is termed secondary injury. Thus, cell distortion and metabolic derangements can lead to cell disruption. 2 , 3 Although cell death results in spinal cord atrophy, care must be taken to consider the size of the uninjured normal spinal cord at baseline as a comparison. 4
The surgical decompression of a mass lesion (bone, disc, tumor, hematoma, etc.) can relieve distortion of the cell and can also relieve metabolic derangements. Augmentation of tissue perfusion pressure, alone, can result in an improved metabolic milieu.
The spine surgeon can do nothing to affect the primary insult (injury), other than to participate in consumer safety and injury prevention programs. Conversely, the secondary injury response to neural injury can be interrupted, at least in part, by pharmacologic interventions. 5 It is not the purpose of this chapter to delve into the neurochemistry and neuropharmacology of neural injury. Suffice it to say that the possibility of minimizing neural injury by pharmacologic means should not be underestimated. Such, however, is not the focus of this chapter or this book.
As mentioned previously, an ongoing primary injury with an obligatory ongoing secondary injury response may frequently follow a neural insult. This ongoing primary injury may be the result of such factors as persistent extrinsic impingement on neural elements and ischemia. Complex biocellular and biomechanical events may contribute to neurologic impairment. 2 , 3 , 6 – 10 These may be closely interrelated. Therefore, the biomechanics of the spine and spine pathology, as well as the biomechanics of neural decompression, fusion, and instrumentation, play a role in the prevention of an ongoing primary injury. The timing of surgery may or may not play a role in the propagation of the ongoing primary injury process. 11 , 12
Four fundamental mechanisms of injury are related to persistent neural element distortion: (1) extrinsic neural element compression, (2) simple distraction, (3) tethering of the neural elements over extrinsic masses in the sagittal plane (“sagittal bowstring” effect), and (4) tethering of the neural elements over extrinsic masses in the coronal plane (“coronal bowstring” effect) (Fig. 8.1). Each must be considered and accounted for before surgical intervention, both so that the neural elements are adequately decompressed by the operative intervention and so that operative intervention itself does not cause neural element distortion.

8.1.1 Extrinsic Neural Element Compression
Spinal cord compression is the most common cause of neurologic dysfunction associated with degenerative disease and trauma. In the case of degenerative spine diseases, compression often results from an annular constriction of the neural elements. This constriction is a result of a combination of factors, such as a ventral osteophyte, dorsolateral facet, and hypertrophic dorsal ligament flavum. Compression is often related to impingement onto the neural elements by an extrinsic mass located only on one side of the neural elements, usually ventral.
The spinal cord consists predominantly of long tracts with relatively little gray matter. In this respect, it differs substantially from brain tissue. Because of differences in blood supply, sensitivity to injury, myelination, and the surrounding bony and soft tissue elements, brain injuries and spinal cord injuries are very different with regard both to theory and to management. Nevertheless, neurons in general can withstand significant external pressure and remain functional. With compressive lesions, therefore, the cause of neurologic dysfunction is often not clear. This perhaps is no more evident than in cases of syringomyelia. 13
As mentioned previously, distortion and metabolic derangements are not well tolerated by the cell. Although the mechanism of injury of a compressive lesion may appear to be pure compression, distortion and ischemia may play significant roles. Degenerative spine diseases, such as cervical spondylolysis, may appear to be causing only compression of the spinal cord via annular constriction. 14 In reality, however, the distortion of neural elements, combined with their exposure to repetitive movement and distortion (trauma), can result in injury dosage accumulation. 15 – 21 This may, in fact, result in objective evidence of clinical neural injury.
A compressive lesion can cause asymmetric deformation of the spinal cord (Fig. 8.2), resulting not only in increased tissue pressure but also in distortion (focal) of the neurons. This causes neuronal dysfunction over and above that resulting from compression alone. Chronic compression may respond clinically to a decompression operation, as does acute compression. 22 , 23 This has significant clinical implications. Although nonoperative management for chronic compression has been recommended, 24 surgical decompression is often recommended as an alternative. Finally, decompression, particularly early decompression, may be associated with neurologic deterioration. 25 Therefore, the timing of surgery and surgical treatment in general are, at best, controversial. 22 , 25 – 27

Finally, ischemia related to decreases in tissue perfusion pressure can also cause neural dysfunction. The clinical relevance of this, however, is often not clear. The role of ischemia in neurologic dysfunction related to neural distortion is speculative, at best. Ischemic susceptibility most certain is affected by the presence of injury in watershed or angiosome territories. Such may warrant preoperative spinal angiography in selected cases when interruption of the radiculomedullary artery of Adamkiewicz is anticipated to be a possible consequence of surgery. 28
8.1.2 Simple Distraction
Simply distracting a neural element may result in electrophysiologic and metabolic dysfunction or cell death. 29 Distraction is associated with two fundamental potentially harmful effects: (1) neuronal distortion and (2) impediment of the blood supply (Fig. 8.3). This may also occur secondary to adhesions that form a cicatrix around neural elements, 13 which, in turn, can similarly disturb blood supply. Again, such mechanisms are truly speculative.

Cusick and colleagues and Breig have studied spinal cord distraction in detail. 30 – 33 Distraction alone may require the application of a considerable force to cause neural dysfunction. However, a combination of injury mechanisms, such as is often seen in cases of trauma, may exaggerate the neural injury. For example, with distraction of the spinal cord over an impinging mass (tethering), much less force is required to cause a given neuronal impairment than with simple distraction alone (Fig. 8.1c). Flexion, extension, and distraction affect the cross-sectional area of the spinal cord, as well. 34 , 35
8.1.3 “Sagittal Bowstring” Effect
An underestimated cause of neurologic dysfunction is tethering of the spinal cord over extrinsic structures. In the sagittal plane, this involves either ventral or dorsal structures. The cervical spinal cord elongates and is displaced significantly during flexion. 36 , 37 This may result in significant distortion. Most often, extrinsic masses located ventral to the spinal cord are implicated. The neurologic deficit in a patient with a focal kyphosis is related, in part, to spinal cord tethering in the sagittal plane (“sagittal bowstring” effect; Fig. 8.4a). 38 This explains why some patients may be neurologically worsened after dorsal decompression procedures (Fig. 8.4b). Morgan and colleagues documented this clinically in patients with posttraumatic ventral mass lesions. 39 The neurologic dysfunction in these cases may be related to vascular compromise in the spinal cord, as is probably the case with simple distraction. Care must be taken in patients with ventral compression secondary to spondylolysis and other pathologies to consider the relationship of the ventral compression. Dorsal operations may not be effective when the sagittal bowstring effect is present. 40 Fusion alone, in fact, has been suggested as a factor leading to improved neurologic outcomes. 41 , 42 This suggests that dynamic factors (i.e., repetitive trauma to the spinal cord) must be involved in the cervical myelopathy process. In other words, a stabilization–fusion procedure “stops the trauma.” This is illustrated by a case of myelopathy in a patient without compression on neutral magnetic resonance (MR) imaging but with compression on flexion MR imaging. Following surgery, the patient’s myelopathy began to improve immediately (Fig. 8.5a–d), despite the fact that his preoperative neutral MR imaging demonstrated no compression. In many respects, surgery functioned by “stopping the trauma.” In this case, a dorsal decompression procedure was employed to decompress ventrally. It was clear on preoperative flexion–extension radiographs that each segment moved (was not fused). Hence, a dorsal release–relaxation procedure via multilevel facet osteotomy was employed to gain the extension necessary to establish an acceptable lordotic posture. Although this patient improved, such repetitive and other chronic distortion processes can result in irreversible injuries. 43


The neurologic ineffectiveness of operative procedures in which dorsal distraction is used for thoracic and lumbar spine trauma has been clearly documented by Dickson and colleagues. 44 They demonstrated, in patients who underwent dorsal distraction fixation (Harrington distraction rods) combined with dorsal fusion without decompression, that the patients so treated enjoyed no greater neurologic recovery than those treated without surgery. However, other reports have documented the “anatomical efficacy” of dorsal distraction procedures 45 – 50 and nonoperative management. 51
Retropulsed bone and disc fragments can be reduced if the posterior longitudinal ligament is intact (Fig. 8.6a). The attempted reduction of ventral spinal masses by this dorsal distraction technique is termed ligamentotaxis (annulotaxis). The rationale for this treatment option is the assumption that, in addition to the presence of an intact posterior longitudinal ligament, the bone and disc fragments are mobile (i.e., they can be relocated) and that the anterior longitudinal ligament does not impede spinal distraction (Fig. 8.6b). One must keep the following in mind: (1) This is unlikely because most injuries are compression injuries, with relative preservation of the anterior longitudinal ligament; (2) the anterior longitudinal ligament is much stronger than the posterior longitudinal ligament and the posterior longitudinal ligament is often disrupted by retropulsed bone and disc fragments, thus rendering it ineffective regarding the ligamentotaxis maneuver; and finally, (3) the spinal cord may be tethered over nonreduced bone and disc fragments during the ligamentous maneuver (see Chapter 1).

Others have shown that ventral decompression operations are effective in improving neurologic function. 52 – 55 Both ventral decompression and dorsal distraction operations, when combined with instrumentation and fusion, effectively restore spinal stability and, in many cases, restore normal spinal canal dimensions. What then, is the cause of the discrepancy between the neurologic outcomes of the two types of procedures? First, most obviously, the normal spinal canal dimensions may not have been completely restored. Even small ventral masses may have clinical significance in the face of spinal cord distraction. Second, the act of reducing retropulsed bone and disc fragments may, by its nature, cause significant tethering during the act of reduction. It seems likely, therefore, that the reason why Dickson and colleagues recognized no neurologic advantage in their dorsal distraction procedures was the collective effect of a number of potential sequelae of the biomechanics of the distraction process.
Of course, some patients may experience neurologic improvement because of effective decompression of the spinal cord with minimal distraction-related distortion or tethering. In others, neurologic improvement may be impeded by tethering of the spinal cord over an incompletely reduced mass (see Fig. 8.6). The adverse nature of this type of outcome may not be immediately obvious on neurologic examination. It may manifest, however, in an early plateauing of neurologic recovery. Finally, the patient’s condition may be worsened by this treatment regimen. The cumulative import of these various neurologic outcomes may indeed be, as Dickson and colleagues observed, that surgery offers no neurologic advantage over nonoperative management. 44
During the operative decision-making process, the surgeon must take care not to misinterpret axially oriented imaging studies, such as computed tomography (CT). Axial images alone can be very misleading with regard to sagittal neural element and extrinsic mass relationships. This is particularly so if spinal deformation in the sagittal plane is present, and if thick axial CT cuts are used (Fig. 8.7).

It is obvious from the foregoing that anatomical restoration of the spinal canal dimensions is not the only important consideration in a spinal decompression and stabilization operation. The surgeon must also consider the mechanism by which restoration of the spinal canal dimensions is to be achieved, as well as the mechanism for achieving a nonpathologic relationship between the neural elements and the spinal elements, so that the neurologic outcome can be optimized. In general, the ultimate goal in surgery for spinal decompression and stabilization is to obtain and maintain a nonpathologic relationship between the bone and soft tissues of the spine and the neural elements. The restoration of normal spinal alignment is not absolutely necessary in all cases.
8.1.4 “Coronal Bowstring” Effect
The spinal cord can be tethered in the coronal plane as well as in the sagittal plane. 38 Coronal plane tethering (“coronal bowstring” effect) is caused by tethering of the spinal cord ventrally by the lateral extensions of the spinal cord proper—that is, by nerve roots or the dentate ligaments (Fig. 8.8a). If coronal bowstring is present, a laminectomy may be ineffective in relieving spinal cord distortion (Fig. 8.8b). 56 Thus, a ventral decompression procedure or a laminectomy, combined with an untethering procedure, is required to relieve the spinal cord distortion adequately. This may be achieved by anterior decompression of the spinal cord or by sectioning of the dentate ligament (Fig. 8.8c). 45 Kahn detailed the anatomical and biomechanical factors involved. 15 In theory, at least, these factors may have clinical roles. This is corroborated by the often-observed cervical spondylolysis-related flattening of the spinal cord that may persist following laminectomy.

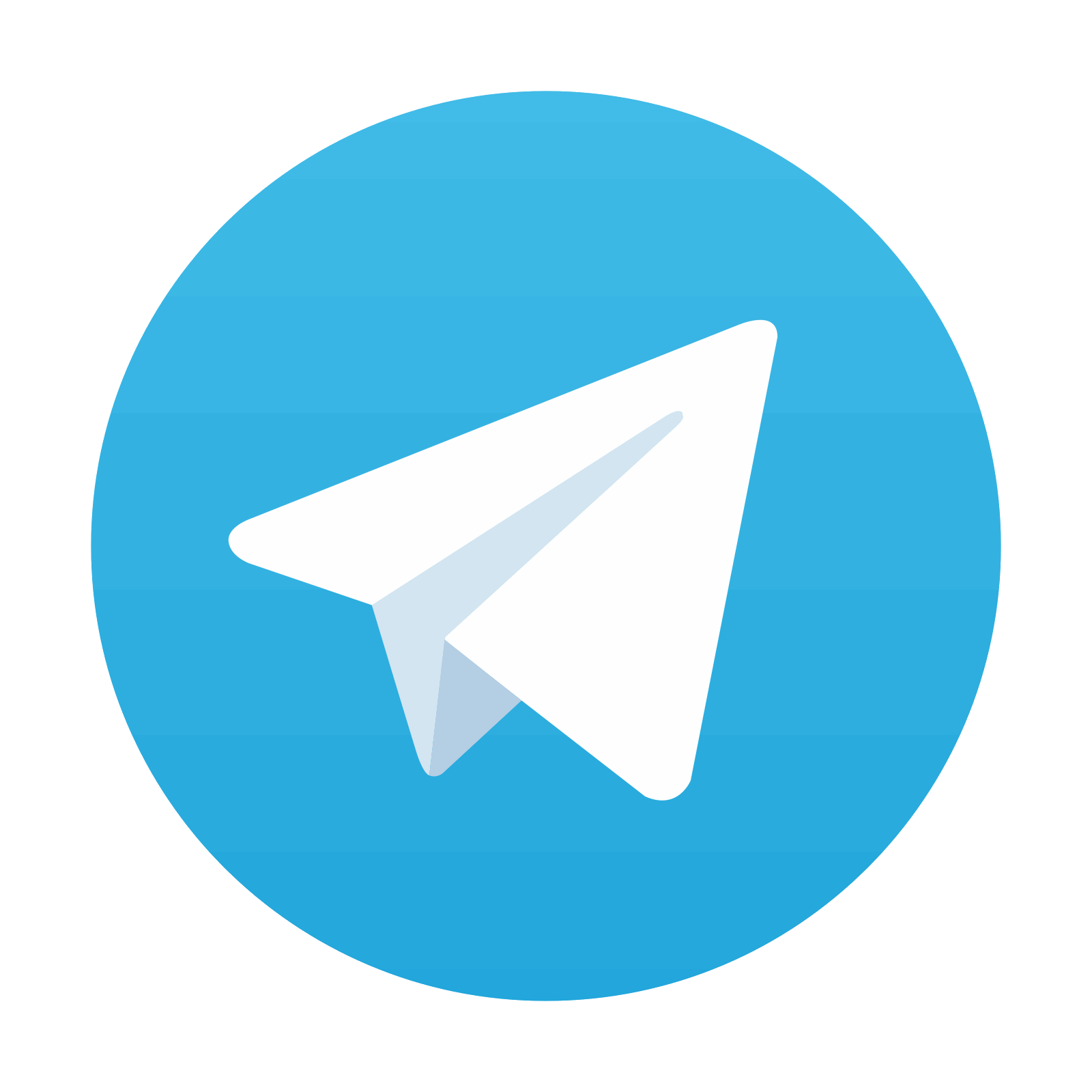
Stay updated, free articles. Join our Telegram channel

Full access? Get Clinical Tree
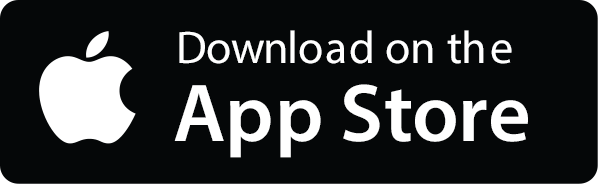
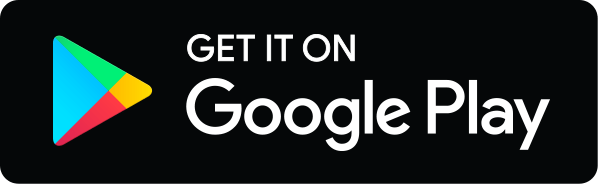
