8 Structural Brain Imaging in Epilepsy
Epilepsy is a common problem, with a prevalence of 0.4 to 1% of the population. Epilepsies and epileptic syndromes are classified into focal and generalized epilepsies. Focal epilepsies account for 40 to 60% of all newly diagnosed cases. Up to 30% of these patients develop intractable epilepsy, despite medical therapy.1,2 In some patients, surgical treatment may lead to cessation or significant improvement in seizure control. The role of structural imaging is to identify the pathological substrate responsible for the epilepsy and to demonstrate the relation of lesion to eloquent areas of the brain. Any epilepsy that is not a benign, idiopathic form of epilepsy requires neuroimaging, including symptomatic focal epilepsy, specific epileptic syndromes pointing to structural brain abnormalities such as Ohtahara or West syndromes, status epilepticus, the so-called catastrophic epilepsies, and progressive deterioration.
Computed tomography (CT) scan is not the first line investigation for epilepsy, but may supplement magnetic resonance imaging (MRI) in the detection of focal area of calcification, such as with tuberous sclerosis or Sturge-Weber syndrome. The main imaging modality for detecting the pathological substrate responsible for epilepsy is MRI. In children with newly diagnosed epilepsy, MRI detected an abnormality in 62/388 (16%).3 In patients with intractable epilepsy, MRI detected an abnormality in 82 to 86% of cases.4,5 MRI has excellent soft tissue contrast, better spatial resolution, multiplanar capability, and higher sensitivity than CT and is therefore the imaging modality of choice. With advances in techniques, previously undetectable subtle structural abnormalities can now be demonstrated by MRI. It is, however, crucial to correlate MRI-identified substrate with clinical and electrophysiological data to avoid false localization. MRI also has prognostic implications: failure to detect a lesion on MRI leads to a worse surgical outcome compared with when a lesion is identified.6–8
MRI Techniques
The sensitivity of MRI for detecting abnormalities depends on the MRI techniques used, the pathological substrate, and the experience of the interpreting physician. Visual assessment of MRIs should be done by experts in epilepsy imaging, with knowledge of the clinical semiology and electroen-cephalography (EEG) findings. An optimal MRI technique for assessing the pathological substrate should include a variety of imaging sequences, including T1-weighted imaging (WI), T2WI, proton density, and fluid attenuation inversion recovery (FLAIR) sequences. These need to be acquired in at least two orthogonal planes covering the whole brain, using the minimum slice thickness. In patients with temporal lobe epilepsy, the coronal plane should be perpendicular to the long axis of the hippocampus to optimize visualization of the hippocampus and mesial temporal lobe structures. For extratemporal epilepsy, the bicommissural plane (i.e., parallel to the anterior commissure-posterior commissure plane) is the standard method used. A three-dimensional T1 volume sequence with slice thickness of 1.5 mm or less should be included because this sequence provides excellent gray/white matter contrast and can be reformatted into any orthogonal or nonorthogonal planes. The three-dimensional volume T1WI can also be subjected to additional postprocessing without the penalty of additional imaging time. Gadolinium does not improve the sensitivity of MRI in patients with epilepsy and should be used only to characterize selective intracerebral lesions, such as tumors. A systematic approach should be used to evaluate MRIs to optimize detection of subtle lesions and dual pathologies.
MRI Spectroscopy
MRI spectroscopy may be used to better characterize a lesion (mostly between tumors, or tumor vs. dysplasia according to the spectral profile), or, by showing a decreased N-acetylaspartate (NAA), to locate the epileptogenic area. However, the changes may reflect both the structural abnormalities and the metabolic alterations related to the seizures, making the interpretation of the results difficult.9–11
Diffusion Imaging
Diffusion imaging has shown striking abnormalities during status epilepticus, reflecting cytotoxic edema, both locally and remotely in the ipsilateral pulvinar12,13; ipsilateral hippocampus13; and contralateral cerebellum (diaschisis-like response)12 as well as in the corpus callosum.12 This brain response to repeated or prolonged seizures may be useful in locating a focus. In addition to conventional MRI, diffusion tensor imaging (DTI) better depicts the structure of the white matter. Although it is not currently used for diagnosis, it may help in understanding the abnormalities of white matter associated with cortical malformations.14 It also helps in preparing the surgical approach to a lesion15 ( Fig. 8.1B ). Perfusion imaging in the setting of epilepsy is still in an experimental stage.
Structural Image Analyses
Structural image analyses describe various computer-assisted methods devised to improve the rate of detection of subtle brain abnormalities in epilepsy. Most use segmentation methods and postprocessing algorithms aimed at quantifying the volume of various compartments of the brain (such as gray and white matter) or specific lobes or structures (such as the hippocampus) or to evaluate the thickness of the cortex or blurring of the cortical–subcortical junction. Unfortunately, these methods are not fully automated, are time-consuming, and are difficult to use in clinical practice.
Multimodal integration of the anatomical data from MRI, functional data from interictal positron emission tomography (PET) or ictal/interictal single photon emission computed tomography (SPECT), and electrophysiological data from cortical or stereo-EEG or from magnetoencephalography (MEG) may also help in identifying the epileptogenic focus with its underlying structural abnormalities.
Epileptogenic Substrates
Although the range of pathological substrates responsible for intractable partial epilepsy in children is similar to that of adults, malformations of cortical development (MCD) and developmental tumors are more commonly detected in surgical specimens of pediatric epilepsy patients. MCD constitute 10 to 50% of pediatric epilepsy cases being evaluated for surgery and 4 to 25% of adult cases of intractable epilepsy.16 By contrast, hippocampal sclerosis is less common in pediatric patients compared with adults. In a series of 126 children undergoing temporal lobectomy for intractable epilepsy, the reported prevalence of hippocampal sclerosis was 13%.6 However, in another cohort of the 109 children, a higher prevalence (45%) of hippocampal sclerosis was reported.17 By contrast, hippocampal sclerosis is the most common epileptogenic substrate in adults with intractable epilepsy and accounts for 50 to 70% of cases.
Epilepsy-Associated Tumors
Epilepsy-associated developmental tumors may account for up to two thirds of the surgical pathological substrate.6,17–19 These tumors originate in and develop from the cortex and, therefore, clinically present with seizures. They are slow-growing tumors, with well-defined margin, without associated edema or necrosis. Complete removal results in good seizure control or renders the patients seizure free. These tumors may developmentally be related to focal cortical dysplasia (FCD).20 They may all derive from the same precursor cells and may have originated from the dysplastic tissue.20,21 FCD may be present in the cortex adjacent to the developmental tumors. The epilepsy-associated tumors include ganglioglioma and its variants, dysembryoplastic neuroepithelial tumor (DNET), pleomorphic xanthoastrocytoma (PXA), and low grade astrocytoma. More glioneuronal tumors have recently been described, but they are uncommon.22

Gangliogliomas
Gangliogliomas are slightly more common in male patients and are 10 times more common in children than in adults.20 They are macroscopically observed to be up to eight times larger in children than in adults.23 They are associated with chronic epilepsy in 85% of cases, and are located mostly in the temporomesial (50%) or temporolateral (29%) location.24 Gangliogliomas and lowgrade astrocytomas were found to be the most common tumors in a temporal lobectomy series.6 Gangliogliomas may present as a solid mass in 43%, a cyst in 5%, and a mixed lesion in 52% of patients25 ( Fig. 8.2A,B ). They involve the cortex, usually broaden the gyri, and may cause remodeling of the adjacent bone. On CT, gangliogliomas may present as hypo- (38%), iso- (15%), hyperat-tenuating (15%), or mixed masses (32%). Calcification may be seen in approximately 30 to 50% of cases. On MRI, the tumor may appear hypo- or isointense to gray matter on T1WI and hyperintense on T2WI. Some may demonstrate intrinsic high T1 signal.25 Enhancement after gadolinium administration is common in up to 60% of cases. It can be nodular, ringlike, or solid. Leptomeningeal involvement may rarely be seen.25 Gangliogliomas histologically consist of two cellular populations: one neuronal and one glial. The neuronal component does not expand, and the glial component may evolve to become anaplastic tumor or glioblastoma multiforme in approximately 6% of cases.25 Patients with ganglioglioma had the best outcome compared with other types of epilepsy associated tumors (92% Engel class I and II).6,18,24
Gangliocytoma
Gangliocytoma is uncommon and affects older children and young adults. It involves the cortex and consists of neurons without glial tissue. It usually has solid and cystic components. On MRI, gangliocytoma demonstrates low T1 and high T2 signals and enhances on the postgadolinium scans.
Desmoplastic Infantile Ganglioglioma
Desmoplastic infantile ganglioglioma (DIG) is a rare, likely congenital tumor that develops in infants. It is usually huge and more often suprasylvian in location. The mass is partly cystic and partly solid. The solid portion incorporates the cortex and is diffusely attached to the dura; it is strongly desmoplastic, and this, together with the age of the patient, characterizes the tumor. In approximately half the cases, the infant presents with macrocephaly, neurological deficits, and seizures. The imaging features are relatively specific when observed in an infant: huge cystic and solid mass involving more often the frontal and parietal lobes, less commonly the temporal lobe, with cranial asymmetry and expansion of the vault. The solid portion, attached to the dura, is mildly hyperattenuating on CT and may be calcified.26 The cystic portion extends into the white matter. On MRIs, the solid portion is isointense to gray matter on T1WI and hyper-, iso-, or hypointense on T2WI, usually heterogeneously. Contrast-enhancement of the solid portion is intense and extends to the dura. The wall of the cystic component does not enhance.26 In spite of the spectacular appearance of the tumor, the prognosis is good.25,26

Dysembryoplastic Neuroepithelial Tumor
DNET was first described by Daumas-Duport et al in 1988.27 It affects the temporal and frontal lobes predominantly. DNET (14%) is less common in children compared with ganglioglioma (43%).18 The lesion may have a well-demarcated margin (50%), or the margins may be slightly blurred.28 The tumor may cause broadening of the gyri, effacement of the sulci, and distortion of the ventricles.28 The tumor may also result in remodeling of the overlying skull vault in 44% of cases.29 On CT, the tumor is hypoattenuating with cystic appearance.30 Calcification has been reported in 20 to 36% of cases.18,29 On MRI, the tumor is of low T1/high T2 signal, often with multicystic, multinodular changes and “bubbly” appearance,28 and it may have a thin rim of high signal on FLAIR sequence ( Fig. 8.3A ). The tumor appears wedge shaped and extends to the ventricle in 30% of cases. One third of cases show faint punctate or ring enhancement. Spontaneous hemorrhage has been reported in DNET.31 The tumor is usually stable over the years. However, a significant increase in size has been documented in a few cases.28,32 DNET has less favorable outcome compared with ganglioglioma, with 70% of patients with DNET having Engel class I and II surgical outcomes.6 Long-term follow-up of DNET has reported reduced seizure-free surgical outcome,33 which may be attributed to incomplete resection of the tumor or the presence of cortical dysplasia beyond the margins of the resected DNET.34 The cortical dysplasia is usually not visible on MRI. The differential diagnoses of DNET include ganglioglioma, low-grade glioma, and FCD. FCD does not enhance and usually does not have mass effect. Gangliogliomas usually have more mass effect. MRI spectroscopy is reported to be normal in DNET,35 whereas high choline and low NAA are observed in gliomas and gangliogliomas.
Another tumor has been described both as a “nonspecific DNET” and as a cortical oligodendroglioma (World Health Organization [WHO] grade II). It is an intracortical hemispheric tumor that presents with isolated epilepsy without neurological deficit or increased intracranial pressure. It has a DNET-like appearance on imaging: triangular cortical lesion with septa, low T1/high T2 signals, without surrounding edema or mass effect, and no enhancement.36 By contrast with the deep oligodendroglioma, this peripheral epilepsy-associated tumor has a good prognosis.36,37
Pleomorphic Xanthoastrocytoma
PXA is a rare tumor affecting children and young adults. Like the other epilepsy-associated tumors, PXA is slow growing, located in the cortex, and highly epileptogenic. It is supratentorial in 98% of cases; mostly temporal (49%) in location; and less commonly parietal, frontal, and occipital.38 The tumor is located in the cortex, has cystic and solid components, and may demonstrate continuity with the dura ( Fig. 8.4B ). On CT, the tumor is predominantly hypodense with mixed density nodule. On MRI, the tumor is hypo- to isointense to gray matter on T1WI and hyper- to isointense on T2WI, and the cystic portion is isointense to cerebrospinal fluid (CSF). Postgadolinium scans demonstrate enhancement of the nodular solid component as well as enhancement of the adjacent meninges (dural “tail”). The enhancing nodule often abuts the pial surface. Calcification is rare, and the tumor is well circumscribed without peritumoral edema.25,39 Hemorrhage has been reported. It contains predominantly glial component but may also contain neuronal elements.40,41 Like DNET, PXA is also associated with cortical dysplasia.40,42 The prognosis is generally good, but it may recur and malignant degeneration occurs in 20% of cases.38


Low-Grade (Fibrillary) Astrocytomas
Low-grade astrocytomas are usually ill-definedinfiltrative tumor located predominantly in the frontal or temporal lobes and invading the cortex. They do not contain ganglionic cells. CT demonstrates ill-defined homogeneous hypodense or isodense mass. MRI demonstrates a homogenous mass that is hypointense on T1 and hyperintense on T2 and may expand the adjacent cortex ( Fig. 8.5B ). The tumor usually does not show enhancement. Calcification and cysts are uncommon, and hemorrhage or surrounding edema is rare. The tumor has an inherent tendency for malignant progression to anaplastic astrocytoma.
Malformations of Cortical Development
Of the different subtypes of malformations of cortical development, the lesions that more commonly undergo surgical management of epilepsy are FCD, hemimegalencephaly, and tuberous sclerosis.

Focal Cortical Dysplasia
FCD is intrinsically epileptogenic and is a frequent cause of epilepsy in children.43,44 Palmini et al.43 have shown selective occurrence of ictal or continuous epileptogenic discharges in FCD compared with other epileptic pathologies such as tumors or arteriovenous malformations. The mechanism of the epilepsy is still unclear. Several possibilities have been proposed, including abnormal firing from the dysplastic neurons rather than from the balloon cells,45 dysfunction of synaptic circuits with abnormal synchronization of the neuronal population, and abnormal organization of the inhibitory interneurons.46 FCD is identified in 18 to 40% of localization-related epilepsy.47–51 There has been a suggestion that FCD has been more frequently reported in the pediatric population than in adult series52: 18% of FCD in surgical specimens of a series of 216 adult patients with intractable epilepsy,49 versus 30 to 39% of FCD in pediatric patients.47,48
Classification and Origin of FCD
The term FCD was originally coined by Taylor et al53 to describe a specific cortical abnormality found in patients who presented with refractory partial epilepsy and who were cured by excision of the affected cortical area. The dysplastic cortex was characterized by disorganized “bizarre neurons” and giant dysmorphic “balloon cells.” Since then, the term FCD has been used extensively in the literature to refer to a wide range of derangements of the cortex, and various classifications have been proposed.50,54–58
The classification proposed by Palmini et al is based on neuropathological findings58:
Mild malformations of cortical development, previously known as microdysgenesis, consist of heterotopic or excess neurons in or outside layer I.
Type IA FCD consists of cortical dyslamination without giant or immature neurons, whereas type IB consists of cortical dyslamination with giant or immature neurons but no dysmorphic neurons.
Type IIA FCD consists of cortical dyslamination with dysmorphic neurons but no balloon cells, whereas type IIB consists of cortical dyslamination with balloon cells.
Both type IIA and IIB FCD have been considered to represent the most severe end of the histopathological spectrum of FCD,56,58 and type IA, type IB, and mild MCD have been considered as the milder end of the spectrum.
Other classifications have been based on the stage at which abnormal development occurred54: FCD with balloon cells has been classified as malformation because of abnormal neuronal proliferation or apoptosis, whereas FCD without balloon cells as well as microdysgenesis has been classified as malformation because of abnormal cortical organization (including late neuronal migration). Work by Englund et al59 suggested that cortical dysplasia with balloon cells was the result of very early disturbance of glialneuronal differentiation and therefore supported its inclusion in malformations of cellular proliferation or apoptosis. Colombo et al60 and Urbach et al61 found that Taylor’s FCD was more likely to demonstrate abnormal signal in the white matter that tapers from the cortex toward the ventricles (transmantle dysplasia).62 During development, cells migrate from the germinal matrix to the cortex along radial glial fibers; abnormal cellular proliferation may in turn affect cellular migration and cortical organization and potentially be responsible for this transmantle abnormal signal. This supports the suggestion that FCD with balloon cells occurs at an earlier stage of cortical development compared with FCD without balloon cells. By contrast, Andres et al63 found an increased number of neurons in layer I of the cortex and the white matter and abnormal morphology of neurons in Taylor’s FCD. They suggested that excessive neurogenesis of late-generated neurons and possible retention of radial glial and subplate neurons may account for the development of Taylor’s FCD, which would then indicate that Taylor’s FCD occurs in the later stage of cortical development.63
Type IA and IB FCD and mild malformations of cortical development are classified as malformations because of abnormal cortical organization.54 These lesions are thought to arise secondary to insults occurring later in the development of the cortex, during late gestation, and they may be acquired up to 2 years postnatally.64–68 Histological findings of dysplastic neurons with giant neurons have been seen after severe perinatal injury such as ventricular hemorrhage in the premature neonate,65 white matter hypoxicischemic injury in premature66 and term neonates,67 early perinatal closed head injury,64 and in nonaccidental injury.68
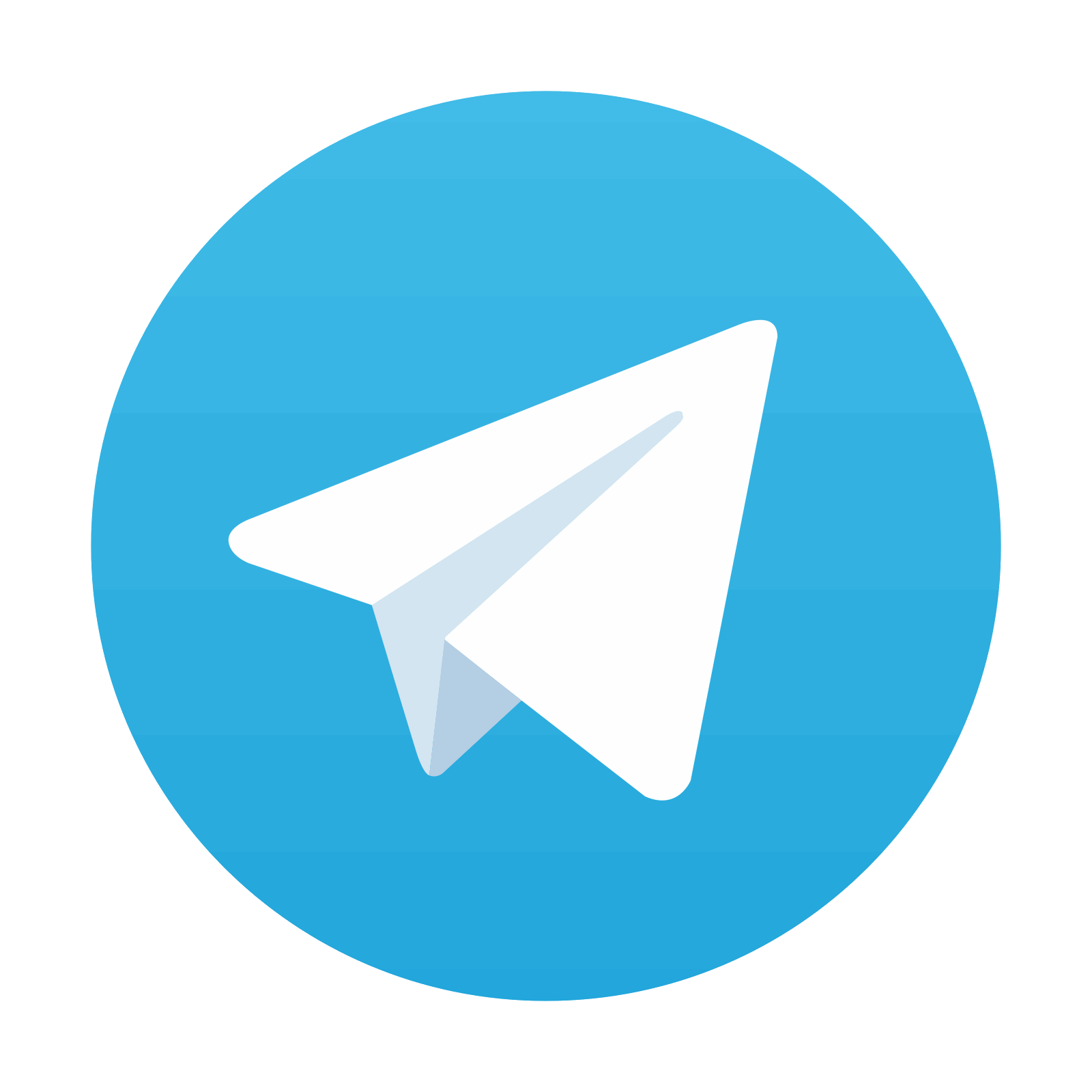
Stay updated, free articles. Join our Telegram channel

Full access? Get Clinical Tree
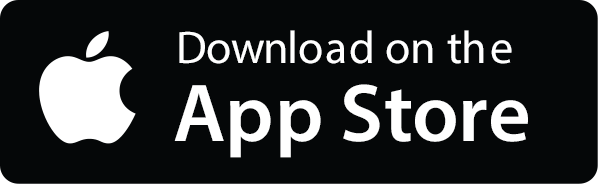
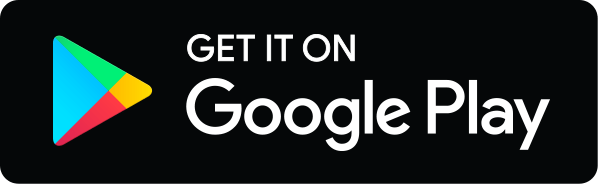
