Macroscopically, the cerebrum is made up of the cerebral cortex, the subcortical white matter, and the basal ganglia, which were discussed in Chapter 8. The gross structure of the cerebrum can be understood best with reference to its embryological development. Its most impressive feature is the immense expansion of the cortex, which causes folding (gyration) of the brain surface. The individual cortical areas are connected to each other, and to deeper brain structures, by the numerous fiber pathways that make up the subcortical white matter.
Histologically, most of the cerebral cortex possesses a six-layered cellular architecture. This basic histological pattern undergoes characteristic variations from one location in the cortex to another, giving rise to numerous, cytoarchitecturally distinct cortical areas. The early neuroanatomists proposed that the specific cellular structure of each area corresponded to the specific task that it carried out. It has, indeed, been possible to assign a single, concrete function to a number of areas, the so-called primary cortical fields. Yet the greater part of the cerebral cortex consists of association areas, whose function apparently consists of higher-level processing of information derived from, or traveling to, the primary fields. Higher cortical functions such as language, in particular, cannot be localized to a single cortical area but depend instead on a complex interaction of multiple areas.
Our ability to localize brain functions with currently available methods remains limited. Moreover, the cerebral cortex has a capacity for functional and structural plasticity.
Development
The cerebrum or endbrain (telencephalon) develops from the paired telencephalic vesicles at the front end of the neural tube, the so-called prosencephalon. The enormous growth of the telencephalic vesicles makes the endbrain envelop the brainstem like a cloak (pallium) and leads to the development of the lateral ventricles, with their characteristic anatomical subdivisions, from outpouchings of the fluid-filled lumen of the neural tube. The semicircular extension that characterizes the growth of the telencephalon (Fig. 9.1) and the lateral ventricles can also be seen in the developing fiber projections, in the fornices, and in the corpus callosum, the great fibrous connection between the two hemispheres. A few more details of telencephalic development will be presented here as a useful aid to the understanding of cerebral anatomy. For further details, the reader is referred to standard textbooks of neuroanatomy and embryology.
Fig. 9.1 Ontogenetic development of the cerebral cortex. Lateral view of an earlier and a later developmental stage of the telencephalon. The telencephalic vesicle expands massively (arrows) in the form of an arch, resulting in simultaneous, archlike expansion of the cerebral cortex (yellow), ventricle (blue), and basal ganglion (orange).
Evolution of the endbrain. In the telencephalon, as elsewhere in the central nervous system, the neural tube consists of two parts, ventral and dorsal. The ventral part gives rise to the septal region medially and the basal ganglion laterally. The basal ganglion, in turn, gives rise to the caudate (“tailed”) nucleus, putamen, claustrum, and amygdala. The cortex derived from the dorsal part becomes differentiated over the course of phylogenetic development into the more laterally lying paleocortex, the oldest portion of cerebral cortex, and the more medially lying and phylogenetically younger archicortex.
The spatial arrangement of paleocortex and archicortex is preserved unchanged in amphibians. In reptiles, however, the neocortex arises in a lateral position between the paleocortex and the archicortex. The neocortex takes on an enormous size in higher organisms, pushing the paleocortex and archicortex far apart. In humans, the paleocortex is ultimately displaced to the base of the brain, where it makes up various components of the phylogenetically ancient olfactory system (olfactory bulb, tract, and trigone, anterior perforated substance, and lateral olfactory stria, see Olfactory System (CN I), Chapter 4). Meanwhile, the archicortex is displaced medially; the semicircular growth of the telencephalic vesicle pushes it into the inferior horn of the lateral ventricle, where it makes up the massive hippocampal formation. Only a thin layer of archicortex is found mediodorsally on the outer surface of the corpus callosum: this is the indusium griseum, with its medial and lateral longitudinal striae. By far the greatest part of the human cerebral cortex is of neocortical origin (
Fig. 9.2).
Fig. 9.2 Phylogenetic development of the cerebral cortex (coronal sections). The neocortex (yellow) arises between the archicortex (red) and paleocortex (blue). It expands markedly in higher organisms; in humans it displaces the paleocortex to the base of the brain (olfactory cortex, not shown) and the archicortex to a medial position overlying the corpus callosum (indusium griseum). The hippocampal formation (archicortex) in the floor of the inferior horn of the lateral ventricle reaches its basal position through the archlike expansion of the telencephalon (cf. Fig. 9.1).
Inside-out stratification of the cerebral cortex. The neurons of the cerebral cortex, as of all parts of the central nervous system, are initially formed in the ventricular zone, i.e., near the fluid-filled lumen (ventricle) of the neural tube. The cells formed earliest make up the so-called preplate, which is later subdivided into the marginal zone and the subplate. The cortical plate proper, consisting of six cellular layers, develops between these two structures. The cortical neurons that are formed first occupy the deeper layers (layers 5 and 6), and those formed later migrate upward into the more superficial layers (“inside-out” arrangement). Thus, the later neurons must travel past their precursor cells to get to the subpial cortical layers (Fig. 9.3), passing from the ventricular zone to the cortical plate along radial glial fibers. The six cortical layers are numbered topographically from superficial to deep (the traditional system, as used in this book). Recent studies have shown that normal neuronal migration, leading to the characteristic inside-out cortical layering, depends crucially on the participation of the Cajal–Retzius cells of the marginal zone. These cells secrete a protein called reelin, which apparently directs neuronal migration along the radial glial fibers (
Fig. 9.3). Abnormalities of neuron formation, migration, or separation from the radial glial fibers are collectively called neuronal migration disorders.
Fig. 9.3 Inside-out lamination of the cerebral cortex. The earliest neurons form the so-called preplate, which soon divides into the marginal zone (M), with its Cajal–Retzius cells (CR), and the “subplate” with its subplate neurons (S). The neurons of the cortical plate are deposited in the intervening space (N1–N3). Early cortical neurons (N1) migrate upward, from the vicinity of the ventricle (V), along radial glial fibers (RF) to the marginal zone of the cerebral cortex, where it is thought that they are stopped by reelin (brown), a protein of the extracellular matrix produced by the Cajal–Retzius cells. As the cortex thickens, later cortical neurons (N2, N3) must travel longer distances to reach the reelin-containing marginal zone. As a result, the neurons that are laid down earliest form the deeper layers of the cerebral cortex, while those that are laid down later form its more superficial layers. P, brain surface with pia mater.
Gross Anatomy and Subdivision of the Cerebrum
The cerebral longitudinal fissure (interhemispheric fissure) separates the two hemispheres down to the corpus callosum. Each hemisphere possesses lateral, medial, and basal surfaces; the transitional area between the (dorso)lateral and medial surfaces is called the parasagittal region. Each hemisphere is also divided into four lobes, namely, the frontal, parietal, occipital, and temporal lobes (Fig. 9.4,
Fig. 9.5,
Fig. 9.6). The insula is sometimes counted as a fifth lobe. The massive enlargement of the mammalian neopallium (= neocortex) achieves its greatest extent in humans, enveloping the phylogenetically older cortical regions in neocortex. Thus, most of the structures derived from the paleocortex and archicortex cannot be seen on the external surface of the brain (the olfactory bulb and tract, olfactory area, paraterminal gyrus, fasciolar gyrus, indusium griseum, dentate gyrus, and hippocampal formation).
Gyri and Sulci
The massive enlargement of the neocortex causes folding of the brain surface into convolutions (gyri) separated by grooves (sulci, fissures). Only about one-third of the cerebral cortex is visible on the external surface, while two-thirds are hidden in the sulci (Fig. 9.7,
Fig. 9.8, and
Fig. 9.9).
Only a few sulci have a relatively unchanging anatomical position. The lateral sulcus (sylvian fissure) separates the temporal lobe from the frontal and parietal lobes. Unlike other named sulci, the lateral sulcus does not merely form the border between two adjacent gyri. It extends deep under the surface of the brain, widening out into a broad, flat space containing CSF, the sylvian cistern, which is not visible from the outside. The sylvian cistern is usually very narrow, almost a virtual space, except in markedly atrophic brains. Its medial wall is the insula (island of Reil; cf. Fig. 9.10 and
Fig. 9.11), sometimes called the buried or central lobe of the brain. The lateral wall of the sylvian cistern is called the operculum (“lid”), because it covers the cistern like a lid; it consists of buried portions of the three lobes of the brain lying around it, which are called the temporal, frontal, and parietal opercula (
Fig. 9.7). A buried portion of the superior temporal gyrus contains the transverse gyri of Heschl (primary auditory cortex,
Fig. 9.10).
Among the other relatively invariant sulci, the central sulcus (rolandic fissure) defines the border between the frontal and parietal lobes. The precentral gyrus, which lies in front of the central sulcus and is therefore in the frontal lobe, contains the primary motor cortex; the postcentral gyrus, which lies behind it and is therefore in the parietal lobe, contains the primary somatosensory cortex. On the medial surface of the hemisphere, the parieto-occipital sulcus forms the border between the parietal and occipital lobes. Its inferior end joins the anterior end of the calcarine sulcus, which lies entirely in the occipital lobe and runs backward toward the occipital pole. Most of the primary visual cortex is located in the depths of this sulcus, and the remainder in the gyri on either side of it. Finally, the cingulate sulcus separates the neocortex from the mesocortex of the cingulate gyrus.
The borders of the occipital lobe are incompletely defined by the parieto-occipital sulcus and the preoccipital notch (Fig. 9.7 and
Fig. 9.8).
The portion of the lateral surface of the frontal lobe that lies anter ior to the precentral gyrus is divided into the superior, middle, and inferior frontal gyri. For the names and locations of all gyri mentioned in this section, and a few others, as well as the names of the sulci that lie between them, see Fig. 9.7,
Fig. 9.8, and
Fig. 9.9. The anatomy of many of the gyri and sulci varies greatly from one individual to another, and even between the two hemispheres of the same individual.
Histological Organization of the Cerebral Cortex
The folded surface of the brain is made up of the gray matter of the cerebral cortex, which is gray because of the very high density of neurons within it. The cortex varies in thickness from 1.5 mm (visual cortex) to 4.5 to 5 mm (precentral gyrus); it is generally thicker on the crown of a gyrus than in the depths of the neighboring sulci.
Laminar Architecture
The laminar structure of the cerebral cortex is visible to the naked eye in only a few cortical areas, most clearly in the visual cortex, where an anatomical section perpendicular to the brain surface reveals the white stripe of Gennari (or of Vicq d’Azyr) within the cortical gray matter. Microscopic examination of most cortical areas reveals the basic six-layered structure that typifies the cerebral cortex (neocortex), as described by Brodmann. Cortical areas possessing this structure are called isocortex (after O. Vogt), as opposed to the phylogenetically older allocortex, which, in turn, is divided into the paleocortex and the archicortex. The paleocortex includes the olfactory area, while the archicortex includes the fasciolar gyrus, hippocampus, dentate gyrus, and parahippocampal gyrus.
The internal structure of the six-layered isocortex is depicted in Fig. 9.12. In an anatomical section perpendicular to the brain surface, the following layers can be distinguished, from outside to inside (i.e., from the pial surface to the subcortical white matter).
1. Molecular layer (zonal layer). This layer is relatively poor in cells. In addition to the distal dendritic trees (apical tuft) of lower-lying pyramidal cells and the axons that make synaptic contact with them, this layer contains mostly small neurons (Cajal–Retzius cells), whose dendrites run tangentially within the layer. The Cajal–Retzius cells play an essential role in the development of the cortical laminar pattern. Some of them degenerate once this development is complete.
2. External granular layer. This layer contains many granule cells (“nonpyramidal cells”) and a few pyramidal cells whose dendrites branch out both within the external granular layer and upward into the molecular layer. The nonpyramidal cells are mostly GABAergic inhibitory neurons, while the pyramidal cells are excitatory and use glutamate as their neurotransmitter.
3. External pyramidal layer. As its name implies, this layer contains many pyramidal cells, which, however, are smaller than those of the deeper cortical layers. These cells are oriented with their bases toward the subcortical white matter. The axon of each pyramidal cell arises from the cell base and travels down into the white matter. The axon already receives a myelin sheath within the external pyramidal layer. It may function as a projection fiber or, more commonly, as an association (see Association Fibers) or commissural fiber (see
Commissural Fibers). A dendrite emerging from the apex of the pyramidal cell travels upward into the external granular and molecular layers, where it divides into its terminal branches (apical tuft).
4. Internal granular layer. Like the external granular layer, this layer contains many nonpyramidal cells. These granule cells mainly receive afferent input from thalamic neurons by way of the thalamocortical projection. The fibers lying in the external pyramidal layer are mostly radially oriented, but those of the internal granular layer are overwhelmingly tangential, forming the external band of Baillarger.
5. Internal pyramidal layer. This layer contains medium-sized and large pyramidal cells. The largest cells of this layer (Betz cells) are found only in the region of the precentral gyrus. The especially thickly myelinated neurites of these cells form the corticonuclear and corticospinal tracts. This layer also contains many tangentially oriented fibers (internal band of Baillarger).
6. Multiform layer. This layer of polymorph cells is subdivided into an inner, less dense layer containing smaller cells, and an outer layer containing larger cells.
Types of Neurons in the Cerebral Cortex
The cerebral cortex thus contains two major types of neurons: the excitatory projection neurons (pyramidal cells) and the other nonpyramidal cells (granule cells or interneurons), which are more commonly inhibitory and tend to make local rather than long-distance connections. But this dichotomy is oversimplified. The interneurons, for example, come in a number of subtypes, such as basket cells, chandelier cells (axo-axonal cells), and double bouquet cells. Furthermore, the pyramidal cells also participate in local regulatory circuits (recurrent inhibition: backward-running local collaterals of the pyramidal cells activate GABAergic inhibitory interneurons, which, in turn, inhibit the pyramidal cells).
The pyramidal cells of the fifth cortical layer give rise to the projection pathways (Fig. 9.13), which travel through the subcortical white matter and the internal capsule to the thalamus, striatum, brainstem nuclei, and spinal cord. The association and commissural fibers traveling to other ipsilateral and contralateral cortical areas, respectively, are derived from the pyramidal cells of the third cortical layer (numbered 4 in
Fig. 9.13). The granule cells of the second and fourth cortical layers, as well as the pyramidal cells, receive projection fibers from the thalamus (1), as well as association and commissural fibers from other cortical areas (2).
Variations of the Laminar Pattern
The six-layered laminar pattern just described is called the homotypical pattern. In some cortical areas, however, the full pattern of six layers is barely discernible; these areas are called heterotypical.
In the receptive cortical fields, such as the visual, auditory, and somatosensory cortices, the density of granule cells is increased, while that of pyramidal cells is decreased (“granulization”; “granular cortex”). In the motor cortical fields, on the other hand, there are relatively more pyramidal cells (“pyramidalization”; “agranular cortex”).
Cytoarchitectural cortical fields. As we have seen, cortical areas vary not only in thickness but also in histological structure. The heterogeneous distribution of various types of neurons across cortical areas, and the resulting variations in the cortical laminar pattern, led the neuroanatomists Brodmann, O. Vogt, and von Economo to subdivide the cerebral cortex into a large number of cytoarchitectural fields. Brodmann’s cytoarchitectural map of the cerebral cortex, which is somewhat simpler than von Economo’s, is now in general use as a system for naming cortical areas. Agranular cortex is found in Brodmann areas 4 and 6 (primary and secondary motor cortical fields); the inner granular layer of these areas is rich in pyramidal cell components. Granular cortex (koniocortex), on the other hand, is found in Brodmann areas 3, 1, 2, 41, and especially 17, the striate cortex (primary receptive cortical areas). As shown in Fig. 9.14, the cytoarchitectural fields do not coincide with the gyral pattern of the brain surface. They partly overlap with one another and vary across individuals in their shape and extent.
Fig. 9.14 Cytoarchitectural fields of the human cerebral cortex. (a) Lateral view of left hemisphere. (b) Medial view of right hemisphere. The cortical fields are numbered. (Reproduced with permission from Brodmann, from Bargmann W. Histologie und Mikroskopische Anatomie des Menschen. 6th ed. Stuttgart: Thieme; 1967.)
It is possible to subdivide the cerebral cortex histologically, not only according to cytoarchitectural criteria but also on the basis of local variations in myelinated fibers, glial cells, or blood vessels (i.e., according to its myeloarchitecture, glioarchitecture, or angioarchitecture). More recent brain maps have also exploited variations in neurotransmitters, neurotransmitter-related enzymes, neuropeptides, and calcium-binding proteins, as revealed by immunohistochemical studies using specific antibodies against these substances.
Plasticity of cortical architecture. The microscopic structure of the cerebral cortex is not strictly genetically determined, nor is it immutable. Much current research concerns the question of how environmental influences, by activating specific groups of neurons, can decisively affect the structural differentiation of cortical areas over the course of ontogenetic development. A further question is whether, and by what mechanisms, long-lasting changes in neuronal activity in the mature brain (e.g., through perturbations of the external environment or loss of a sensory organ) can actually bring about changes in the microarchitecture of the cortex, including a changed anatomy of synaptic connections.
Many studies of this kind have been performed on the visual system, because the environmental conditions affecting it (visual stimuli) are relatively easy to manipulate. It has been found that certain “elementary components” of visual stimuli, including their color, orientation, and localization on the retina, are processed separately by distinct groups of neurons, which are distributed over the visual cortex in small, interspersed areas. These specialized cortical areas take on different characteristic shapes, depending on the elementary aspect of visual processing with which they are concerned: color is processed in so-called blobs, while the spatial localization and orientation of the stimulus are dealt with by ocular dominance and orientation columns. Experimental manipulation of a given type of elementary stimulus, for a sufficiently long period of time, can be shown to produce morphological changes in the corresponding processing units.
Input-specific differentiation of cortical microstructures can be demonstrated in other areas as well. The cortical barrels of the rodent somatosensory cortex, composed of annular collections of cells, are a well-known example: each barrel represents a single whisker of the animal.
Thus, a large number of recent studies permit the following general conclusions: (1) certain cortical areas contain a topical representation of the sensory stimuli that they process; (2) this representation can undergo plastic change.
The diversity of histological structure among cortical fields immediately implies that they must have correspondingly diverse functions. For well over a hundred years, much research has focused on the assignment of function to different cortical fields. The knowledge that has been gained is of vital clinical importance. We will discuss functional localization in detail in the section after next, but first, as a necessary prerequisite, the fiber connections of the cerebral cortex will be presented.
Cerebral White Matter
Each hemisphere contains a large amount of subcortical white matter, which is composed of myelinated nerve fibers of varying thickness and neuroglia (mainly oligodendrocytes, the cells that form myelin sheaths).
The subcortical white matter is bounded by the cerebral cortex, the lateral ventricles, and the striatum. Its nerve fibers are of three types:
Projection Fibers
Projection fibers connect different parts of the central nervous system with each other over long distances.
Efferent fibers. Efferent fibers from the cerebral cortex traverse the subcortical white matter and then come together to form the internal capsule. As discussed in Chapter 3, these are the corticonuclear, corticospinal, and corticopontine fibers, as well as the fibers that link the cerebral cortex with the thalamus, striatum, reticular formation, substantia nigra, subthalamic nucleus, midbrain tectum, and red nucleus. The long efferent corticospinal fibers arise mainly in areas 4, 3, 1, and 2, and also in area 6, while fibers to other destinations, such as the corticopontine and corticothalamic fibers, arise from larger association areas of the cortex.
Afferent fibers. These travel from the thalamus to extensive areas of the cerebral cortex. These include fibers of all somatosensory modalities, which travel to areas 3, 1, 2, and 4, as well as other fibers carrying impulses from the cerebellum, globus pallidus, and mamillary body by way of the thalamus to the cerebral cortex. The thalamus is the last major relay station that sensory impulses must traverse before reaching their specific primary cortical areas and is therefore sometimes called the “gateway to consciousness.” Olfactory fibers are the only exception to this rule: they reach the cortex directly, without any thalamic relay.
Thalamocortical reciprocity. Most thalamocortical projections are reciprocal (i.e., there are fibers running in both directions). The cerebral cortex is thus presumed to modulate its own input by means of a feedback loop between the cortex and the thalamus. These massive thalamocortical and corticothalamic projections make up the large white matter tracts known as the anterior, superior, posterior, and inferior thalamic peduncles, which are usually collectively termed the corona radiata. The topical organization of the thalamic projections is their most important anatomical feature.
Association Fibers
The association fibers (Fig. 9.15 and
Fig. 9.16) make up most of the subcortical white matter. These fibers connect neighboring distant cortical areas of the same hemisphere with each other. The cerebral cortex is able to carry out its diverse associative and integrative functions only because all of its functionally important areas are tightly interconnected and neural impulses can travel easily from one cortical area to another. These extensive fiber connections between cortical areas may also be an important anatomical substrate for the partial recovery of function often seen in the aftermath of cortical injury (e.g., after trauma or stroke). Over time, as the individual practices the impaired activities, performance may improve because the corresponding neural impulses have been redirected along the remaining, intact pathways.
The superior longitudinal fasciculus runs dorsal to the insula and connects the frontal lobe with large parts of the parietal, occipital, and temporal lobes. An extension of it, the arcuate fasciculus, winds around the posterior end of the lateral sulcus (sylvian fissure) in the depths of the subcortical white matter. This fiber bundle connects the frontal and temporal language areas (of Broca and Wernicke) with each other. Lesions of the arcuate fasciculus produce conduction aphasia (see Table 9.1). The inferior longitudinal fasciculus connects the temporal lobe with the occipital lobe. The uncinate fasciculus travels around the anterior end of the lateral sulcus like a hook, connecting the orbital frontal gyri with the anterior portion of the temporal lobe.
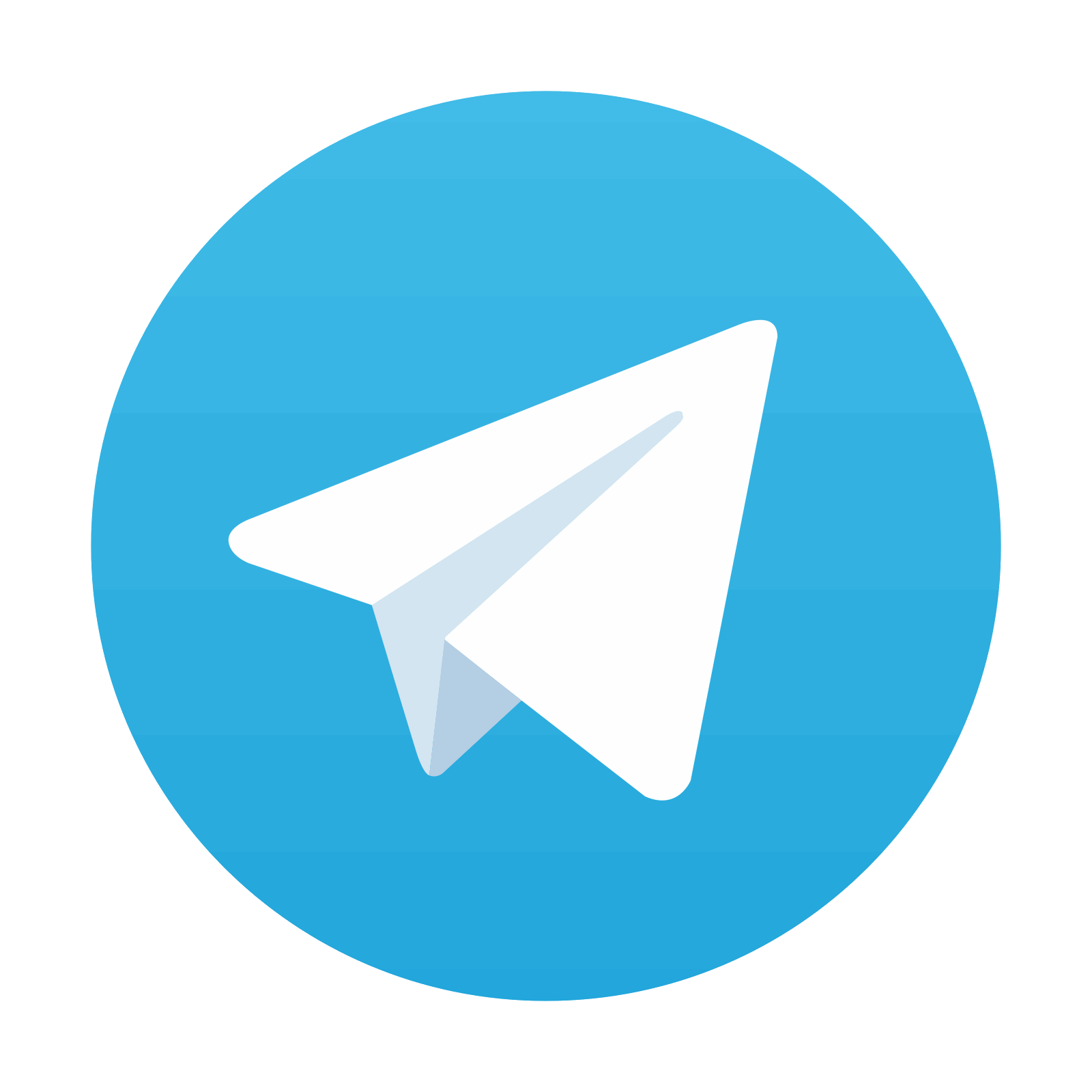
Stay updated, free articles. Join our Telegram channel

Full access? Get Clinical Tree
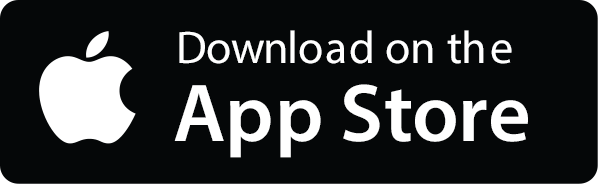
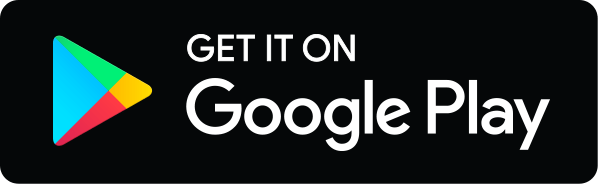