Summary
This chapter covers the clinical applications of radiotherapy in skull base tumors. We discuss the role of adjuvant radiotherapy after surgery, definitive radiotherapy when surgery is not recommended, skull base re-irradiation, and palliative radiotherapy. We provide a comprehensive review of clinical outcomes and complications after skull base radiotherapy for benign and malignant tumors. Studies evaluating modern conformal radiotherapy approaches are highlighted. Benign tumors covered in this chapter include pituitary adenoma, acoustic schwannoma, meningioma, craniopharyngioma, and paraganglioma. Malignant tumors covered here include skull base chordoma and chondrosarcoma, nasopharyngeal carcinoma, and sinonasal malignancies. Tables comparing outcomes after conventional fractionation, hypofractionation, and stereotactic radiosurgery are provided. The optimal clinical management and appropriate dose and fractionation for a specific tumor biology and tumor location are discussed.
9 Clinical Applications of Radiotherapy for Skull Base Tumors
9.1 Introduction
Tumors of the skull base can be categorized by location and/or histologic type. Anatomically, skull base tumors may arise from the following:
neurovascular structures and meninges of the base of the brain (e.g., schwannoma, meningioma, pituitary adenoma, craniopharyngioma, paraganglioma);
the base of skull itself (e.g., chordoma, chondrosarcoma, osteosarcoma, plasmacytoma); and
head and neck structures below the skull base (e.g., nasopharyngeal carcinoma [NPC], paranasal sinus tumor, perineural spread of head and neck tumors).
In addition to a tumor’s anatomic location, its subtype often dictates the role and sequence of radiotherapy. Carcinoma arising from the nasopharynx is treated using radiation as the primary modality due to the morbidity associated with resection of tumors arising from this location, whereas carcinoma arising from the paranasal sinus often requires a combination of surgery and radiation therapy to optimize local disease control. Clival chordoma requires very high radiation doses for tumor control and is well suited for proton therapy. High-grade malignant tumors that have a high propensity for nodal recurrence, such as squamous cell carcinomas, have dictated the use of elective nodal irradiation (ENI) fields, whereas benign tumors typically have sharp margins of demarcation from the surrounding normal structures and are amenable to highly conformal local radiation with a steep radiation dose gradient beyond the target.
This chapter covers the clinical radiotherapy applications for skull base tumors. The management of skull base tumors using radiotherapy requires an understanding of both tumor radiobiology and modern treatment planning, delivery, and monitoring techniques; these aspects are discussed in Chapter 8 of this book.
9.2 Normal Tissue Constraints of Critical Organs of the Skull Base
As mentioned in the previous chapter, most skull base tumors are well suited for both fractionated radiotherapy and stereotactic radiosurgery (SRS). Patients who are irradiated for skull base tumors are at risk of developing radiation-induced complications as a result of injury to the normal brain parenchyma and critical structures. Table 9.1 lists the commonly quoted radiation dose constraints for conventionally fractionated (1.8–2.0 Gy per fraction) radiotherapy (FRT), single fraction SRS, and multisession hypofractionated stereotactic radiotherapy (FSRT). Critical structures at risk include the brain parenchyma, brainstem, spinal cord, optic pathway, and inner ear/cochlea. Significant effort should be made to keep the radiation doses to these critical structures below the constraints during radiation treatment planning so as to minimize the risk of radiation-induced complications.
9.3 Pituitary Adenoma
Pituitary adenoma is a broad term for benign primary tumors arising from pituitary glandular tissue. They can be divided into nonsecretory and secretory types. Depending on the type of secretory cells from which they arise, secretory pituitary adenomas can be subdivided into growth hormone (GH)–secreting, prolactin-secreting, adrenocorticotrophic hormone (ACTH)–secreting, gonadotropin-secreting, and thyroid stimulating hormone–secreting tumors. The goals of treatment of pituitary adenomas are tumor control and normalization of the hypersecreted hormone for secretory tumors. Except for prolactinomas, surgical resection is usually recommended because such an approach can decompress the optic apparatus, if it is compressed by the tumor, and can rapidly normalize the hypersecreted hormone in a secretory tumor.1 When complete surgical resection is not achievable, postoperative radiation therapy is often necessary to stop the growth of the residual tumor and normalize the hypersecreted hormone. Radiation therapy is also indicated for recurrent pituitary adenomas.
9.3.1 Growth Hormone–Secreting Adenoma
There is abundant literature on the use of FRT to treat pituitary adenoma. The typical doses used range from 45 to 54 Gy in conventional fractionation. For nonfunctioning pituitary adenoma, the reported 10-year progression-free survival rate after FRT ranges from 80 to 98%.2 , 3 , 4 , 5 , 6 , 7 For secretory tumors, apart from control of tumor growth, normalization of hypersecreted hormones is one of the main goals of treatment. For GH-secreting tumors, FRT is efficacious in the normalization of GH. However, GH levels tend to fall slowly after treatment and may take years to normalize. Typically, by 10 years, approximately 70 to 90% will have normalized GH levels.3 , 4 , 8
Multiple studies have evaluated the efficacy of GH normalization rates after SRS and FRT. These studies have yielded no clear significant differences by radiotherapy technique and may be primarily dependent on preradiotherapy GH and insulin-like growth factor (IGF-I) levels.9 Because of the metabolic effects and cosmetic deformities associated with acromegaly, medical therapy may be given to patients before their GH levels normalize. Colleagues from St. Bartholomew’s Hospital reported one of the world’s largest series of patients who had acromegaly from pituitary adenoma treated using radiotherapy. GH level decreased to < 2.5 ng/mL in 22%, 60%, and 77% of the 884 patients treated with radiotherapy for acromegaly at 2, 10, and 20 years, respectively.10 Notably, 63% of patients had a normal IGF-I level by 10 years. Another recent study showed similar findings.11
9.3.2 PRL-Secreting Adenoma
When evaluating treatment outcomes, it is important to distinguish between pituitary stalk effect and prolactin hypersecretion. The serum prolactin level is usually lower in the case of stalk effect, which is defined as the loss of hypothalamic inhibition due to compression of the pituitary stalk. Conventional fractionated radiation therapy is usually offered to patients who cannot tolerate bromocriptine or similar medical therapy or to those who develop disease progression during medical therapy. Normalization of prolactin levels occurs in 50 to 70% of patients after fractionated radiation therapy.12 , 13 , 14 , 15 , 16 , 17 , 18
9.3.3 Adrenocorticotrophic Hormone–Secreting Adenoma
Patients who have ACTH-secreting tumors present with Cushing’s disease when the tumor is still small and thus usually have microadenomas. Because these tumors are typically very small, they might not be detected on a regular brain MRI. A dynamic MRI or bilateral selective venous sampling of ACTH from the inferior petrosal sinuses can be performed to establish a diagnosis. The metabolic effects of Cushing’s disease are very crippling and, if long-standing, can be fatal. Accordingly, the goal of treatment is rapid normalization of the ACTH, and surgical resection is usually the recommended initial treatment. Fractionated radiation therapy is recommended in patients who have residual tumor after surgery and in patients who are medically inoperable. Remission occurs in approximately 50 to 80% of patients after fractionated radiation therapy.19 , 20 , 21
9.3.4 Stereotactic Radiosurgery for Pituitary Adenoma
SRS has been used in the treatment of pituitary adenomas. Because the optic apparatus is in close to the pituitary gland, a 3- to 5-mm gap between tumor and organ is typically needed to respect the tolerance of the optic chiasm. Abundant data in the literature demonstrate that SRS is a safe and efficacious procedure for the treatment of pituitary adenomas. However, if one anticipates that to deliver an adequate dose to the tumor or target volume, the maximum dose to the optic apparatus cannot be limited to 8 to 10 Gy in a single fraction, then FRT or FSRT in three to five fractions should be recommended instead. SRS is very effective at controlling tumor growth, yielding a tumor control rate of 92 to 100%.22 The tumor control rate in patients who have endocrine-inactive pituitary tumors treated to lower marginal doses of 14 to 16 Gy is similar to that of patients who have secretory pituitary tumors treated to marginal doses of 14 to 34 Gy. When judging normalization of hormone level, however, inconsistencies in the endpoints used in various studies render interpretation of results difficult. A decrease in hormone hypersecretion can occur within a few months of SRS, but complete normalization can take up to 8 years. Data in the literature suggest that a radiation dose response is involved in normalization of hormone levels. It has also been suggested that the use of antisecretory medications at the time of SRS has a negative impact on the efficacy of the procedure. For endocrine-inactive tumors, a dose of 14 to 16 Gy is usually used; for secretory tumors, a higher dose should be considered.
From the endocrinologist’s standpoint, an endocrinological cure of acromegaly is usually defined as a GH level of ≤ 1 ng/mL and a normal IGF-I. A comprehensive review examining the outcomes of SRS for the treatment of acromegaly showed that the rate of endocrine cure ranged from 20 to 82%.22 This wide variation might be a result of the different percentage of patients receiving antisecretory medications during SRS. For prolactinomas, SRS results in an endocrine cure rate of approximately 30% and a significant reduction of PRL levels in 29 to 100% of patients.22 The post-SRS stalk effect may cause PRL to be slightly elevated even when hypersecretion is well controlled.
For ACTH-secreting tumors, reported endocrine cure rates after SRS widely ranged from 10 to 100%.22 , 23 However, some of the studies did not specify the criteria for endocrine cure, and other studies used differing criteria. Among the studies having defined criteria of endocrine cure, the rates of endocrine cure ranged from 28 to 100%.23
9.3.5 Fractionated Stereotactic Radiotherapy
Recent data on the use of FSRT (typically delivered over three to five fractions) for the treatment of pituitary adenomas is promising. Tumor control rates ranged from 85 to 98%, and reported complication rates were low.1 Although no direct comparisons of radiotherapy modalities have been performed in a randomized clinical fashion, a recent meta-analysis of eight studies enrolling a total of 634 patients evaluated the efficacy and safety of FSRT (n = 361) and SRS (n = 273) for treatment of pituitary adenomas.24 Comparable tumor control rates ranged from 91 to 96%, comparable rates of radiation-induced optic neuropathy ranged from 0 to 2%, and comparable rates of endocrinologic deficits ranged from 1 to 22%. Independent of technique used, whether Gamma Knife (GK)-„, CyberKnife (CK)-„, or linear accelerator (LINAC)-based, tumors larger than 4 mL were associated with a higher rate of treatment complications (2% vs. 1–2% optic neuropathy; 22% vs. 1–7% pituitary dysfunction) and lower rates of tumor control (91% vs. 96–99%)24 when compared with those < 4 mL.
9.4 Acoustic Schwannoma
Acoustic schwannomas (vestibular schwannomas) represent the most common cerebellopontine angle tumor (~ 80%). There are four management options for acoustic neuromas: observation, microsurgery, SRS/FSRT, and FRT. Tumor control for all local therapeutic interventions is excellent and focuses on minimizing treatment-related complications, principally hearing preservation and cranial nerve (CN) preservation. In trying to identify the optimal management option, consideration is given to patient age, tumor size, growth rate, symptom burden, and the goals of hearing and CN preservation. Because acoustic schwannomas are slow-growing tumors, some physicians favor close observation for smaller tumors. However, it should be noted that tumor progression can result in permanent loss of hearing function and can increase the risk of complications associated with surgery or radiotherapy. Meta-analyses and prospective data evaluating the natural history of acoustic schwannomas in patients suggest that those having a tumor growth rate > 2.5 mm per year were more likely to lose hearing function.25 , 26
Comparisons have been made between microsurgery and SRS, demonstrating that SRS yields similar tumor control rates but lower incidence of CN deficits. These findings appear to hold as long-term data for SRS emerge.27 , 28 , 29 Acoustic schwannomas are ideal targets for SRS, and the literature contains an abundant clinical data on the use of SRS for acoustic schwannoma. GK is the SRS modality most commonly used to treat these tumors, but LINAC-based and CK modalities are used as well, with excellent conformality and accuracy. As of 2011, more than 18,000 patients who had acoustic neuroma had been treated using GK.30 In the past, more rudimentary CT-based planning was used, and plans were typically less conformal, reflecting the state of earlier computer algorithms. Modern treatment planning entails MRI-based planning and the use of a larger number of shots to improve the conformality around the tumor.31
9.4.1 Modern Stereotactic Radiosurgery Series for Acoustic Schwannoma
Table 9.2 summarizes the treatment outcomes of selected series for acoustic schwannoma. The prescribed dose for acoustic schwannoma has been lowered since the early 2000s. In earlier studies, marginal doses in the range of 16 to 20 Gy were used.31 , 32 , 33 Reported tumor control rates were excellent, but incidence of radiation-induced hearing loss and CN deficits (mainly trigeminal and facial) was substantial. This prompted investigation of a lower prescribed dose. Modern series using a dose of 12 to 13 Gy did not show inferior tumor control rates,28 , 29 , 31 , 32 , 33 , 34 , 35 , 36 , 37 , 38 , 39 , 40 , 41 , 42 , 43 , 44 , 45 and a much higher proportion of patients retained serviceable hearing. There was also lower incidence of trigeminal and facial nerve injury from SRS. One of the largest series of acoustic schwannoma patients treated using GK-SRS (n = 829) comes from the University of Pittsburgh. The 10-year tumor control rate was 97%.33 An update of their 15-year experience showed that facial neuropathy risk was < 1% and that trigeminal symptoms were < 3%, with hearing preservation in up to 77% of patients.33 A separate report from the same hospital demonstrated that tumor control was not compromised when a reduced dose of 12 to 13 Gy was used.34 Other series using reduced-dose SRS reported similar high tumor control rates of up to 96%, with up to 88% retention of hearing and little or no facial deficits (0–4%).37 , 39 , 41 , 42 , 43 Recently, colleagues at Northwestern reported outcomes for 30 patients treated using GK-SRS to a prescribed dose of 11 Gy. They demonstrated 100% progression-free survival, based on freedom from surgery, and 91% freedom from persistent growth. One patient developed tumor progression requiring resection at 87 months.44 Similar long-term outcomes using a LINAC-based SRS system was reported by a group from the University of Heidelberg, Germany. Median dose was 13 Gy, prescribed to the 80% isodose line. Median follow-up was 110 months, and 5- and 10-year tumor control rates were 91%.37 Rates of radiation-induced trigeminal neuralgia and facial weakness were 8 and 5%, respectively, and hearing preservation at 9 years was 55%.
SRS using proton beam has yielded similar excellent results, albeit with a shorter follow-up time. Colleagues from Harvard University reported a 5-year tumor control rate of 95.3%, with a median follow-up of 38.7 months in those receiving proton beam SRS. The prescribed dose was 12 cobalt Gray equivalent (CGE). The trigeminal and facial nerve toxicity rates were 10.6 and 8.9%, respectively. The hearing preservation rate was 33.3%.
9.4.2 Fractionated Radiotherapy and Fractionated Stereotactic Radiotherapy for Acoustic Schwannoma
Patients who have large acoustic schwannoma not suitable for SRS may be offered FRT; FRT series for acoustic schwannomas have historically consisted of 1.8 to 2.0 Gy daily doses in five fractions per week, for a total dose of 50.4 to 54 Gy. The conventional fractionation schedule was initially developed empirically to provide an optimal “normal tissue sparing effect” between tumor control and treatment toxicity.47 The largest body of experience with FRT comes from the University of Heidelberg. A total of 106 patients who had acoustic schwannoma were treated to a prescribed dose of 57.5 Gy.38 The 5-year tumor control rate was 93%, with a median follow-up of 48.5 months. Treatment toxicities were low, with 3.4%, 2.3%, and 6% developing trigeminal nerve, facial nerve, and vestibulocochlear nerve complications, respectively. Investigators from the University of California, Los Angeles and Thomas Jefferson University demonstrated similar results subsequent to FRT. The probability of retaining serviceable hearing in patients who had sporadic tumors was ~ 81%.45 , 47 Investigators from Loma Linda University reported proton beam FRT to a prescribed dose of 54 CGE (or 60 CGE for patients who had no serviceable hearing) in 30 fractions.35 The tumor control rate was 100%, with a median follow-up of 34 months. No trigeminal or facial nerve toxicity was reported. The hearing preservation rate was 31%.
With the advent of intensity modulated radiation therapy (IMRT) and stereotactic localization, FSRT using higher doses per fraction (4–5 Gy in 5 Fx) can be delivered with minimized dose to adjacent critical structures. FSRT fills the gap between SRS and conventional FRT in terms of time commitment and patient selection strategies. FSRT regimens of 3 Gy × 10, 5 Gy × 4, 5 Gy × 5, 4 Gy × 5, 6 Gy × 3, and 7 Gy × 3 have been described for patients who have acoustic schwannoma.30 , 46 , 48 , 49 , 50 , 51 Tumor control rates are comparable, and median follow-up intervals for FSRT studies ranged from 21 to 48.5 months. Investigators from Amsterdam prospectively evaluated 129 patients treated using LINAC-based stereotactic radiotherapy either as a single fraction (10–12.5 Gy prescribed to 80%; n = 49) or FSRT (4–5 Gy × 5 fractions; n = 80). Tumor control (100% SRS vs. 94% FSRT), hearing preservation (75% SRS vs. 61% FSRT), and facial nerve preservation (93% SRS vs. 97% FSRT) were not significantly different between the two groups. There was a slight edge for better trigeminal nerve preservation (92% SRS vs. 98% FSRT; P < 0.05) in the FSRT group.46 The same group evaluated FSRT for larger acoustic schwannoma. The median tumor size was 9.4 mL (range 8–24). The tumor control rate was 94%, with a median follow-up of 48 months. Hearing was retained in 85% of patients, and 24% experienced a transient enlargement of tumor prior to arrested growth or shrinkage.52 Overall, outcomes after FSRT demonstrate similar tumor control to FRT, ranging from 94 to 100% with the benefit of lower overall time commitment to patients who had tumors that were not suitable for SRS.
9.4.3 Long-Term Complications after Acoustic Schwannoma Irradiation
The current active area of research is focused on techniques for improving hearing preservation. Unlike hearing preservation, rates of trigeminal and facial nerve toxicity after radiotherapy are low irrespective of the radiation modality used. Hearing preservation after radiotherapy is more varied, with approximately a quarter to a third of patients developing complications related to hearing. The large variation in hearing preservation is partially due to variation in hearing scales and the definition of hearing preservation. In terms of radiotherapy technique, a pooled analysis from three large German centers (Heidelberg, Munich, and Freiburg) evaluated long-term outcomes in 449 patients treated for acoustic schwannomas. Of these, 169 patients received SRS (median dose 13 Gy) and 291 patients received FRT (median dose of 57.6 Gy at 1.8 Gy per fraction). The 10-year local control rate was similar for both groups, at ~ 94%.36 There was no difference in trigeminal or facial nerve toxicity between FSRT and SRS. Serviceable hearing was preserved in 85% of patients, with loss of useful hearing in 14% of FSRT and 16% of SRS patients. Among those treated using SRS to < 13 Gy, loss of useful hearing was lower, at 13%. Dosimetric studies comparing SRS to FSRT appear to demonstrate an overall advantage to SRS because of the accuracy of a fixed head registration and the steep dose gradient of SRS. However, patients who have preexisting mass effect symptoms are more likely to develop local swelling and worsening of symptoms after SRS. The use of steroids in the immediate post-SRS period can mitigate these potential complications and reduce the need for surgical intervention. Because of its small volume and variable thickness, and the variability of user delineation on imaging, determining the dose-volume limitations for the cochlea is difficult. Several studies have evaluated the association between cochlear dose and persistent hearing loss.41 , 53 , 54
Pan et al assessed hearing changes in patients after unilateral FRT, using the contralateral ear as standard. A mean cochlear dose of > 44 Gy was associated with > 10 dB increases in bone conduction threshold, whereas the mean dose to the contralateral ear was 4.2 Gy (range 0.4–31.3 Gy).53 Similarly, other studies found a significant increase in sensorineural hearing loss when the dose received by the cochlea exceeded 45 Gy.54 , 55 In a long-term follow-up report, from the Medical University of Vienna, Austria, of 426 patients treated using GK SRS to a median dose of 12 Gy prescribed to 70% isodose line (small intrameatal tumors), 60 to 70% isodose line (Koos grade II) and 50% isodose line (Koos grade III or IV), hearing function prior to treatment and the median dose (> 6 Gy) to the cochlea were independent predictors of serviceable hearing. Studies using MRI-based dose-volume analyses of the cochlea indicate that critical cut-off doses associated with loss of serviceable hearing after SRS were between 3 and 5.3 Gy.56 , 57 , 58 Using bone window CT to delineate the cochlear volume, the median cochlear volume was 80.9 mL (range 51.6–114.2), and hearing preservation was associated with a cochlear dose < 4 Gy in those treated using a fixed-margin dose of 12 Gy.59 Similarly, in a study evaluating CT-based volumetric cochlear dose and serviceable hearing, 105 patients who had pretreatment serviceable hearing received GK SRS. The authors demonstrated that a mean cochlear dose > 4.9 Gy was associated with time to nonserviceable hearing.60
In addition to hearing and CN V and VII function, radiotherapy effects on temporal bone structures are also considered in the radiation treatment plan. Radiation-induced damage to temporal bone structures can manifest as otitis media/externa, mastoiditis, osteoradionecrosis, fibrosis, vertigo, or hearing impairment. It is still too early, owing to SRS dose reduction in the last 10 to 15 years, to draw conclusions about whether FSRT or SRS with lowered prescribed doses has the advantage in terms of the therapeutic ratio.30 For tumors up to 3 cm in size, SRS, FSRT, and FRT offer good local control and comparable toxicity. With the advent of IMRT and particle therapy coupled with stereotactic localization, the field of radiotherapy for acoustic schwannoma is rapidly evolving.
9.5 Meningioma
Incidental, asymptomatic, and radiographically stable meningiomas may be observed, with treatment withheld until symptoms and/or growth develops. Skull base meningiomas can be addressed using skull base surgical techniques. Meningiomas tend to infiltrate the critical structures that reside in the region of the skull base and can prevent complete resection from being performed safely, leading to increased risk of tumor progression. To improve tumor control, postoperative radiation therapy is indicated for patients who have subtotally or minimally resected newly diagnosed or recurrent skull base meningiomas.61 CN complications are in general higher for meningiomas than paragangliomas or schwannomas for a given location of the skull base.
Abundant data in the literature support the use of conventional fractionated 2D and 3D-CRT therapy for management of patients who have subtotally or minimally resected newly diagnosed or recurrent skull base meningiomas.61 The majority of the series included meningiomas at the skull base as well as in non–skull base locations. In most cases, the typical prescribed dose ranged from 50 to 54 Gy for benign meningiomas. For patients who receive radiation therapy after subtotal resection, it is reasonable to expect a 10-year local control rate of at least 70 to 80% based on the long-term reports in the literature. For patients who have unresectable disease, radiation therapy provides a degree of tumor control and symptomatic relief. In the series from Royal Marsden Hospital, United Kingdom, the reported 5-„, 10-„, and 15-year disease-free survival rates for patients who had unresectable meningiomas were 53, 47, and 47%, respectively.62 For patients who had recurrent meningiomas, there was some indication that outcomes were not compromised if radiation therapy was given at the time of recurrence instead of immediately after subtotal tumor resection.63 However, because of the presence of critical structures such as the optic apparatus, CNs in the cavernous sinus, and brainstem, progression of a skull base meningioma can result in significant neurological morbidity. This question is best answered in a clinical trial setting. The European Organization for Research and Treatment of Cancer is conducting a trial that randomizes patients who have subtotally resected or biopsied World Health Organization grade 1 cerebral meningiomas to observation or postoperative external beam radiation therapy or SRS.
9.5.1 Conformal Radiotherapy for Skull Base Meningioma
Because meningiomas have a sharp margin of demarcation with normal brain parenchyma, they are excellent targets for highly precise radiation techniques such as IMRT, SRS, FSRT, and proton beam therapy (Fig. 9.1). The main advantage of using these techniques is minimizing radiation dose delivery to the areas outside the target volume. IMRT can produce highly conformal isodose distribution around a meningioma target while minimizing dose to surrounding critical structures or organs. It is well suited for targets with complex shapes, such as meningiomas. Colleagues from Baylor College of Medicine treated 40 meningioma patients (32 who had skull base tumors) with IMRT to a dose of 50.4 Gy. For a median follow-up of 30 months, the 5-year tumor control rate was 93%.64 Acute and late complications were low and occurred in 2.5 and 5% of the patients, respectively. Another study, from the University of Heidelberg, examined the treatment outcomes of 20 patients who had skull base meningiomas treated using IMRT to a prescribed dose of 57.6 Gy. For a median follow-up of 36 months, the tumor control rate was 100%. Approximately 25% of treated tumors showed shrinkage. The incidence of late complications was 10%.63 A study of 35 patients who had 37 meningiomas treated using IMRT to a prescribed dose of 50.4 Gy at the Cleveland Clinic Foundation showed similar findings. The median follow-up was 19.1 months and the 3-year local control was 97%. No late complications were observed.65 Although the results are promising, these studies had relatively short follow-up intervals ranging from 19.1 to 36 months. Extended follow-up is required to determine whether IMRT can improve the therapeutic ratio.

9.5.2 Stereotactic Radiosurgery for Meningioma
Data on SRS for the treatment of skull base meningioma have matured in the literature over the past two decades.61 , 66 , 67 The typical prescribed dose was 12 to 18 Gy. Numerous reports demonstrate high efficacy and low toxicity associated with SRS for treatment of skull base meningioma.66 , 68 , 69 , 70 Colleagues from Mayo Clinic reported the results of 49 patients who had cavernous sinus meningioma treated using GK-SRS.70 The mean margin dose was 15.9 Gy. For a median follow-up of 58 months, the tumor control rate was 100%. Symptoms improved for 26% of patients who had preexisting diplopia or facial numbness/weakness. Trigeminal nerve function worsened after treatment in 10% of patients. At the University of Pittsburgh, 129 patients who had cavernous sinus meningioma were treated using GK-SRS. The median margin dose was 13 Gy.71 Neurologic status remained stable or improved after treatment in 91% of patients. Tumor progression occurred in 6% of patients. Adverse effects occurred in 6.7% of patients. The 5- and 10-year tumor control rates were both 93.1%. At the same institution, 62 patients who had petroclival meningiomas were treated using GK-SRS to a median dose of 11 to 20 Gy.72 Median follow-up was 37 months, and tumor progression occurred in 8% of the patients. New CN deficits occurred in 8% of patients.
Colleagues from the University of Pittsburg recently published their long-term outcomes in 290 patients treated for meningioma with GK-SRS. The 10- and 20-year freedom from tumor progression rates were 88 and 87%, respectively.73 Symptoms improved for 20% of patients, and 94% of asymptomatic patients remained asymptomatic. No differences in control rates were found between those who had undergone surgery prior to SRS (89%) versus SRS alone (93%). Treatment toxicity was low, at 3.1%. Other SRS series, either GK- or LINAC-based, showed similar tumor control and toxicity rates for skull base meningioma.74 , 75
FRT can be offered to patients who have skull base meningiomas that are not suitable for treatment using SRS, either due to the size limit or due to their proximity to critical structures such as the optic apparatus. Data on FRT for skull base meningiomas have emerged over the past 10 years. Colleagues from Royal Marsden Hospital, in the United Kingdom, reported their outcomes for patients who had mostly skull base meningiomas treated using FRT.76 The prescribed dose was 50 to 55 Gy. None of these patients developed any tumor recurrence over the period of follow-up. The group from the University of Heidelberg reported results for one of the largest FRT series for skull base meningiomas. The prescribed dose was 56.8 Gy. The tumor control rate was 98.3% and the complication rate was 1.6%, with a median follow-up of 35 months.77 , 78 Other studies using the same approach showed similar tumor control and complication rates.77 , 79 , 80
9.5.3 Proton Beam Therapy for Skull Base Meningioma
Secondary to the lack of wide availability of proton beam facilities worldwide, protons have not been routinely used in patients who have skull base meningiomas. A few studies have reported on proton beam therapy as the sole modality, or combined with photon beam therapy, for the treatment of skull base meningioma. Investigators from Institut Curie, France, treated 51 skull base meningioma patients with a combination of photon and proton beam therapy.81 For a median follow-up of 25.4 months, the 4-year local control was 98%. The prescribed dose was 60.6 CGE. Investigators from South Africa treated 23 patients using proton beam radiotherapy, either hypofractionated (31.5 CGE in three fractions) or conventionally fractionated, ranging from 54 CGE in 27 fractions to 61.6 CGE in 16 fractions.82 For median clinical and imaging follow-ups of 40 and 31 months, respectively, tumor control was achieved in 88% of the patients treated using hypofractionation and in 100% of those treated using conventionally fractionated proton beam therapy. Other proton beam radiotherapy series for meningiomas that included tumors of all locations showed similar outcomes.83 , 84 The follow-up times of these studies are relatively short. Considering the indolent nature of benign meningiomas, a prolonged follow-up duration is necessary to determine long-term outcomes with proton beam therapy.
9.6 Craniopharyngioma
Craniopharyngiomas are benign tumors arising from Rathke’s pouch. Although they are slow-growing and well circumscribed, their frequent involvement of adjacent structures such as the optic apparatus, pituitary stalk, hypothalamus, and major blood vessels can contribute to significant morbidity and render safe complete surgical resection difficult. Surgical intervention is the standard initial therapy for the purpose of tissue diagnosis and decompression. Attempts at achieving complete surgical resection can be associated with significant morbidity. When complete resection is not possible, limited surgical resection and postoperative radiation therapy can be used to achieve satisfactory rates of tumor control. Conventional fractionated radiation therapy has been shown to be effective in the setting of postoperative treatment as well as for salvage treatment. Because craniopharyngiomas are very well circumscribed tumors, they are very suitable targets for advanced radiation therapy techniques such as IMRT, SRS, FSRT, and proton beam therapy. In patients who have a cystic lesion, intralesional phosphorus-32 (P-32) may be used to treat the tumor.
9.6.1 Fractionated Radiotherapy for Craniopharyngioma
Conventional radiation therapy may be offered in two different settings: for initial treatment of a subtotally resected tumor and for salvage treatment of recurrence after surgical resection. Data in the literature showed that radiation therapy is effective at reducing the risk of recurrence in both settings.85 The most commonly prescribed dose is 50 to 54 Gy. For patients who received immediate postoperative radiation therapy, 10- and 20-year local control or progression-free survival rates ranged from 57 to 89.1% and from 54 to 79%, respectively.85 For patients who received postoperative radiation therapy as salvage treatment, similar outcomes were observed. This raises the question of whether delayed instead of immediate radiation therapy should be employed, especially in young children.
Colleagues from Harvard University reported their experience combining photons and protons for treatment of craniopharyngioma.86 A total of 15 patients, 5 children (median age 15.9 years) and 10 adults (median age 36.2 years), were treated using 160 MeV proton therapy either for the entire course of treatment or in combination with photon beam therapy. The median dose given was 56.9 CGE. For a median follow-up of 11 years, 5- and 10-year local control rates for the 11 surviving patients were 93 and 85%, respectively. The 10-year overall survival (OS) rate was 72%. None of the 10 adults treated had any change of functional status or working ability. One of the five children had learning difficulties comparable to preradiation therapy level; the remaining children had professional achievements.
9.6.2 SRS for Craniopharyngioma
Both GK- and LINAC-based SRS have been used to treat craniopharyngiomas.87 , 88 , 89 , 90 Because of the proximity of most craniopharyngiomas to the optic apparatus, there is a risk of radiation-induced visual disturbance if the radiation dose exceeds the tolerance level. Typically, a 3- to 5-mm gap between the tumor and optic apparatus is needed to limit the radiation dose to the structure. Most SRS series showed a local control rate of 86 to 90%.90 , 91 In one Swedish series, the rates of tumor progression were 85 and 33% for tumors receiving < 6 Gy and ≥ 6 Gy, respectively.92 Longer follow-up is needed to determine the efficacy and toxicity of SRS for craniopharyngiomas, because the majority of series had follow-up times of less than 3.5 years. In a few series that had longer-term follow-up, 5-year progression-free survival (PFS) and OS rates were 52 to 68% and 86 to 97%, respectively. Taipei Veterans Hospital recently reported on a 20-year experience treating 137 patients using GK-SRS for craniopharyngiomas.91 Median follow-up was 45.7 months, and control rates were 73% (solid), 74% (cystic), and 66% (mixed tumors). The 5-year PFS rate was 70%, and 8% had new onset or worsening pituitary deficiency.

9.7 Paraganglioma
A paraganglioma is a tumor that arises from specialized neural crest cells called paraganglia and that can develop from various sites of the body. Clusters of paraganglia are called glomus bodies (or glomus tumors) and are associated with autonomic ganglia. The majority (~ 90%) are benign and sporadic.93 Approximately 10% of cases are familial and often develop multiple tumors and occur in younger patients.94 They are associated with mutation of the succinate dehydrogenase gene family, among other genes. Approximately 3% develop within the head and neck, and the main pattern of spread is local. Nodal and distant metastases are rare. Only about 2 to 5% secrete catecholamines. Paragangliomas of the head and neck can originate from four primary sites (Fig. 9.2) and are named by their location:
Carotid body tumor arises at the bifurcation of the common carotid artery.
Glomus vagale arises along the extracranial course of the vagus nerve.
Glomus jugulare arises at the jugular bulb.
Glomus tympanicum arises in the middle ear from the inferior tympanic branch (Jacobson’s nerve) of the glossopharyngeal nerve (CN IX).
Glomus jugulotympanicum arises from the mastoid branch (Arnold’s nerve) of the vagus nerve (CN X) and is found between the middle ear and jugular foramen.
Presentation varies depending on the location of origin and the affected CN. Carotid body tumors are the most common (accounting for 60% of paragangliomas) and often present as a slow-growing, mobile, painless neck mass. Larger tumors can be associated with CN palsies of the vagal (CN X) and hypoglossal (CN XII) nerves and the sympathetic chain. Temporal bone paragangliomas such as glomus jugulare tumors can result in local bone destruction and present with impairment of CNs IX, X, XI, and XII from mass effect. Glomus tympanicum tumors are associated with hearing loss, pulsatile tinnitus, and disequilibrium and appear as a reddish mass behind the ear drum (known as the “red drum”) on physical exam.95 Glomus vagale tumors are the least common of the head and neck paragangliomas and can present as an intraoral parapharyngeal mass or a painless lateral neck mass behind the angle of mandible; they are associated with dysphagia and hoarseness. Glomus tumors typically grow at a rate of 1 mm per year, with a median doubling time of 4 years.96 Because these tumors are hypervascular, they are usually demonstrated very well on CT with contrast. CT can be useful to evaluate bone erosion. They also have a characteristic appearance on MRI. Angiography is used to evaluate patients preoperatively.
9.7.1 Radiotherapy for Paraganglioma
The goals of the treatment are tumor and symptomatic control. Treatment options include surgical resection, conventionally fractionated FRT, hypofractionated FSRT, and SRS. Because glomus tumors are rare, even in large medical centers, no consistent treatment algorithm has been developed. The optimal treatment approach depends on tumor location and size as well as on the medical comorbidities of the patient. Surgery is the preferred first-line option, particularly for carotid body tumors, with the goal being a complete resection. However, resection of a skull base paraganglioma such as those of jugulotympanic origin may require sacrifice of one or more CNs, resulting in permanent treatment morbidity. In a study by Fayad et al of 83 patients who had glomus jugulares, a gross total resection was achieved in 81% of cases, but 18.9% of patients developed new CN deficits after surgery. CN injury for larger skull base paragangliomas and vagale tumors can remain high even when using more advanced skull base surgery techniques and preoperative embolization.97
In cases in which the risk of surgical resection is too high, or after a subtotal resection, radiation therapy is a safe and effective treatment.98 , 99 , 100 , 101 , 102 Historically, radiotherapy was reserved for elderly or debilitated patients who had unresectable or extensive tumors. However, radiotherapy advances and emergence of long-term data have shown that radiotherapy is associated with high rates of tumor control and low rates of long-term complications. Radiotherapy is often considered first-line treatment for paragangliomas located in the skull base or vagale tumors. Unlike the goal of surgery, which is complete tumor removal, the goal of radiotherapy is to stop tumor growth and/or reduce tumor size over time. In terms of target delineation, CT, MRI, and angiography can be used. Because the risk of nodal metastasis is low and tumors are often confined, the clinical target volume typically does not include regional nodes or require extensive subclinical coverage of microscopic infiltration. This makes highly conformal radiotherapy techniques highly attractive for paragangliomas.
9.7.2 Fractionated Radiotherapy for Paraganglioma
FRT delivered to a moderate total dose of 45 to 50 Gy with conventional fractionation (1.8–2.0 Gy per fraction daily) offers excellent tumor control and has the longest and largest experience. In a comprehensive review of all the articles published on radiation therapy for glomus tumors from 1965 to 1988 using a variety of delivery techniques and dosing schedules, the median tumor control was 93%, and the risk of severe complication was 2 to 3%.103
Table 9.3 summarizes the treatment outcomes of selected radiation therapy series for glomus tumors. The efficacy and complication rates were not associated with site of origin, whether the carotid body or the vagal ganglia or jugulotympanic in nature. Tumors that recurred typically manifested between 1 and 8 years after treatment. Most of the severe complications were related to use of older 2D and 3D techniques, doses that exceeded current recommendations, and re-irradiation.
9.7.3 Stereotactic Radiosurgery and Fractionated Stereotactic Radiotherapy for Paraganglioma
Disadvantages of FRT include the longer treatment course (typically 5 weeks), the need for larger planning target volume (PTV) margins to account for daily treatment setup uncertainties, and, in younger patients, the risk of a secondary radiation-induced malignancy. SRS or FSRT offers an attractive alternative, because treatment can be administered in five or fewer fractions with similar local control and complication rates (Fig. 9.3). SRS/FSRT can be used as first-line treatment or as salvage therapy after treatment failure.

Long-term outcomes data after SRS are limited compared with those for surgery and FRT. Follow-up times range from 8 to 12 years for SRS and from 3 to 7 years for FSRT. Across multiple SRS series for glomus jugulare tumors, median tumor control rate was 95% (range 63–100%) and symptomatic improvement rate was 40% (range 25–60%).100 , 108 , 109 , 110 , 111 , 112 , 113 , 114 , 115 , 116 , 117 , 118 , 119 , 120 , 121 Complications such as worsening of preexisting CN deficits, tinnitus, and vertigo occurred in 8.5% of patients (range 4–40%), of which 6.5% were transient and 2% permanent. The median dose was ~ 15 Gy (range 12–32 Gy) prescribed to the 50% isodose line in a single session. The use of 13 Gy or less was associated with higher recurrence rates. In a meta-analysis of 19 studies representing 335 patients who had glomus jugulare tumors, performed by Guss et al at John Hopkins, Stanford, and UCSF, the rate of tumor control was 95% among studies with median follow-up of longer than 36 months.122 There were no differences among SRS systems, with similar high rates of tumor control seen for GK (~ 97%; range 94–99%) and LINAC/CyberKnife (~ 97%; range 92–100%) studies. The authors felt that the clinical outcomes with SRS might be better than reported in the study, because radiosurgery is usually reserved for patients who have contraindications for surgery, residual disease after surgery, or recurrent disease, thus imparting a potential inherent bias whereby tumors that are refractory to surgery may be more aggressive. In a published study by the North American Gamma Knife Consortium (Sheehan et al) on 132 patients from eight GK centers whose paragangliomas were treated to a median dose of 15 Gy, tumor control was 88% at 5 years, with a median follow-up of 50.5 months. Patients demonstrating new or progressive CN deficits were more likely to demonstrate tumor progression (P = 0.002). Pulsatile tinnitus improved in 49% of patients, and 11% had improvement in CN deficits. At the Mayo Clinic, 57 patients were treated using SRS between 1990 and 2007 to a mean dose of 15.3 Gy, and local control rates were 100 and 83% at 7 and 10 years, respectively.
Data using FSRT are limited to smaller series with limited follow-up. In general, the tumor volumes treated using FSRT were larger than for single-session SRS series. Several reports used LINAC or CK techniques to doses of 21 to 27 Gy in three fractions or 25 to 30 Gy in five fractions and reported 96 to 100% tumor controls rates with a follow-up duration of ~ 2 years.123 , 124 , 125 Treatment complications rates were minimal, with no new or worsening of preexisting neurologic deficits in all but one study (Wegner and Lieberman), which showed 19% grade 1/2 adverse events (Chun).
Randomized prospective clinical data comparing surgery with FRT or SRS/FSRT are not available. In a systemic literature review by Suarez et al of 2,042 patients who had glomus jugulare and glomus vagale tumors treated using surgery (n = 1,310), FRT (n = 461), or SRS (n = 261), median follow-ups were 66 months, 113 months, and 41 months, respectively. Tumor local control rates were higher with radiotherapy (91.5%) than with surgery (78.1%; P < 0.05), and the probability of a major complication was lower with radiotherapy (11%) than with surgery (26%; P < 0.05). The authors calculated an iatrogenic cranial neuropathy rate of 1.0 per patient with surgery versus 0.08 per patient with radiotherapy (P < 0.001). Local control rates for temporal bone paragangliomas were similar between FRT and newer surgical techniques, but complication rates (CN palsies) were higher with surgery.
An important consideration in the use of radiotherapy for patients who have benign skull base tumors with long-term survivorship is the risk of radiation-induced second malignancies. Although the overall risk is low, development of a radiation-induced sarcoma of the skull base is often fatal. Lalwani et al and Gilbo estimated the rate of radiation-induced sarcoma to be 0.5 to 5% at an average of 8 to 15 years.126 , 127 The risk of secondary malignancy with FRT is about 1 in 500, with a latency period of 8 years or longer. The predicted risk of radiation-induced second malignancies with SRS appeared lower. To date, very few cases of radiation-induced malignancy after SRS have been reported, leading some to estimate that the risk of radiation-induced malignancy is negligible with SRS.108
Factors that help determine the optimal treatment algorithm for patients who have paraganglioma include tumor location, presenting symptoms, age, comorbidities, tumor volume, imaging characteristics, and rate of growth. Close observation is reasonable for older patients who are asymptomatic and who have comorbidities. Younger patients who have large tumors and significant associated mass effect or high rates of circulating catecholamines may benefit from immediate surgical resection and cytoreduction with consideration of adjuvant radiotherapy based on pathologic risk factors. However, in cases in which CN injury with surgery is likely, radiotherapy can be considered. For paragangliomas of skull base and temporal bone, radiotherapy can be considered as a first-line approach. Good candidates for SRS are those having small- to moderate-volume (< 15 mL) tumors and nonsecreting tumors located above C2. Larger tumors and tumors below the skull base are better suited for FRT. FSRT offers a promising alternative to FRT by combining the advantages of highly precise stereotactic planning and delivery with the convenience of a short-treatment course and the functional sparing advantages of fractionation.
9.8 Chordomas
Chordomas are rare tumors that originate from the remnants of the embryonal notochord. Approximately a third of these tumors occur in the skull base. They are low-grade tumors that grow locally and aggressively, with very high recurrence rates.128 Local control is the most prognostic factor for survival,129 and complete surgical resection is the cornerstone to optimal local control, which is the key prognostic factor in survival; however, gross total resection can be challenging due to local infiltration of skull base structures and the proximity of critical neurovasculature. Accordingly, these tumors are most often treated using maximal safe resection and postoperative radiation therapy. Postoperative radiation therapy is employed to reduce the risk of local recurrence. The most common cause of death is uncontrolled local tumor progression, so local tumor control is of utmost importance.130 Unfortunately, the location of these tumors renders the delivery of a sufficiently high dose of radiation to the area difficult even when using highly conformal techniques that involve photon beam therapy, subsequently resulting in nondurable tumor control. Treatment outcomes achieved with the use of conventional fractionated radiation therapy have been disappointing.
9.8.1 Radiotherapy for Chordoma
The typical prescribed dose for conventional fractionated radiation therapy is 50 to 60 Gy.131 , 132 , 133 Other strategies for intensifying the radiation dose to skull base chordomas, such as SRS and FSRT, have also been used.133 In the SRS series from Mayo Clinic, for a median follow-up of 4.8 years, 2- and 5-year tumor control rates were 89 and 32%, respectively.134 The median margin dose was 15 Gy. Patients who had prior fractionated radiotherapy had a higher risk of radiation-induced complications. More recent reports on SRS have suggested that higher biologic radiation doses improve local control. Kano et al from the University of Pittsburgh reported a 5-year control rate of 66%. Other studies also suggested that SRS may be an effective treatment for skull base chordomas.135 , 136 FSRT has also been used to treat skull base chordomas. The group from the University of Heidelberg treated patients who had skull base chordomas using FSRT. The prescribed dose was 66.6 Gy. The 2-and 5-year local control rates were 82 and 50%, respectively.137
9.8.2 Proton Therapy for Chordoma
Proton radiation therapy is well suited for the treatment of clival chordomas. Because of the absence of an exit dose, a very conformal dose distribution around the target volume can be achieved with proton radiation therapy (PRT). This allows delivery of a higher radiation dose to the tumor so as to improve local control (Fig. 9.4). A large body of experience has been accumulated over the years on the use of PRT for skull base chordoma.138 In the United States, much of the early clinical experience published on use of PRT for skull base chordomas came from the Lawrence Berkeley National Laboratory, Loma Linda University, and Harvard University. Investigators from Harvard University reported one of the largest experiences (n = 519) using PRT (five fractions per week, four with protons and one with photons) for the treatment of skull base chordoma (n = 290) and low-grade chondrosarcoma (n = 229).130 , 139 The prescribed dose was 66 to 83 CGE, conventionally fractionated, and a relative biological effectiveness (RBE) of 1.1 was used. Median follow-up was 41 months, and 5- and 10-year local recurrence-free survival rates were 64 and 42%, respectively. Severe complications occurred in 8% of patients. Hug et al from Loma Linda University treated 58 patients who had skull base chordoma (n = 33) and low-grade chondrosarcoma (n = 25) using proton beam therapy. The median dose was 64.8 to 79.2 CGE.130 For a mean follow-up period of 33 months, 8 (24%) of 33 patients who had chordomas experienced recurrence. The 3-year local control rate was 67%. The 5-year OS rate was 79%. A group from Institut Curie treated 100 patients who had base of skull or cervical spine chordomas using fractionated radiotherapy that combined photons and protons. A prescribed dose of 67 CGE was given. For a median follow-up of 31 months, a total of 25 patients developed recurrence. The 2- and 4-year tumor control rates were 86.3 and 53.8%, respectively.140 Colleagues from Boston (Munzenrider et al) published their results from 169 patients treated using combined proton and photon with a median follow-up of 41 months. Their 5- and 10-year local control (LC) rates were 73 and 54%, and corresponding OS rates were 80 and 54% at 10 years after photon–proton combination.139 The French group (Noel et al) showed 4-year LC and OS rates of 54 and 80% in 100 patients at 31-month follow-up using combined proton and photon (Centre de protontherapie d’Orsay).140 Other proton beam radiotherapy series have shown similar results.141 , 142 , 143 , 144 , 145

More recently, spot scanning proton therapy and intensity-modulated proton therapy (IMPT) have been used for treatment of skull base chordomas.142 , 143 In contrast to passive scatter, IMPT offers greater control over the proximal aspects of the beam and improved conformality of high-dose regions and allows use of a simultaneous integrated boost technique similar to IMRT for treating multiple target volumes to discrete doses. Investigators from the Paul Scherrer Institute evaluated the use of IMPT for treatment of skull base chordomas and reported a 5-year local control rate of 81% in 64 patients treated to a median dose of 73.5 Gy (RBE), with 94% freedom from grade 3 or 4 toxicity at 5 years.142 Similarly, at the University of Texas’s MD Anderson Cancer Center, IMPT prescribed to a mean dose of 69.8 Gy has been used for treatment of patients who have skull base chordomas, with similar results.146 Compared with passive scatter plans, IMPT demonstrated improved high-dose conformality and showed better sparing of temporal lobes and brainstem. Given the more favorable outcomes associated with the use of PRT than can be achieved using photon beam irradiation alone, proton beam radiotherapy should be considered in most patients who have skull base chordomas.
9.8.3 Carbon Ion Therapy for Chordoma
Carbon ion therapy combines the physical advantages of protons with the differential increase in relative biologic effectiveness. It is currently not available in the United States and in fact is available only in a few facilities worldwide. Early clinical data from investigators at the University of Heidelberg reported a 3-year tumor control rate of 81% for patients who had chordomas, with no excessive toxicity observed.147 At the German Ion Research Center (GSI), in a phase I/II trial of carbon ion therapy for the treatment of skull base chordomas and chondrosarcomas, with a median follow-up of 32 months, the 4-year actuarial local control for chordomas was 74%.148 The prescribed dose was 60 GyE at 3 GyE daily, 7 days a week. These results appeared to be as favorable as those achieved using proton beam therapy. However, longer follow-up is required to determine the long-term efficacy and toxicity associated with the treatment. Uhl et al recently published their long-term data on 155 patients treated with carbon ions using an active raster scanning technique for skull base chordomas.149 , 150 The median dose was 60 Gy (RBE 3 Gy), and median follow-up was 72 months. The 5-year LC and OS rates were 72 and 85%, respectively; 10-year rates were 54 and 75%, respectively. Age < 48 and boost volume > 75 mL were associated with improved outcomes. This study represents the largest cohort examined after carbon ion treatment and the second-largest cohort of patients to date with skull base chordoma treated with carbon ion therapy.
Clinical data from other users of particle therapy, including Loma Linda (Hug EB et al),151 the Paul Scherrer Institut (Ares et al),143 and the National Institutes of Radiological Science (Mizoe et al),152 suggest better LC and survival rates than seen with conventional techniques. Currently there are no randomized data comparing use of protons, SRS, and carbon ion therapy.
9.9 Chondrosarcomas
Chondrosarcomas are heterogeneous neoplasms that constitute 10 to 20% of all malignant bone tumors. Those occurring in the head and neck region most commonly arise from the sphenopetrosal and the spheno-occipital synchondroses, the nasal cavity, and the paranasal sinuses. Complete surgical resection is the mainstay of treatment. Postoperative radiation therapy is needed to reduce the risk of recurrence in higher-grade lesions. A high dose of radiation is required to achieve permanent local control. Much like skull base chordomas, skull base chondrosarcomas are subject to the radiation dose constraints of surrounding critical or normal structures, so that the delivery of a high dose of conventional photon beam radiotherapy is often not possible.
9.9.1 Radiotherapy for Chondrosarcoma
Advanced radiation techniques such as conventionally fractionated IMRT, SRS, and FSRT have been used to treat patients who have skull base chondrosarcoma, particularly those who have smaller primary tumor volumes (Table 9.4). At the University of Heidelberg, eight patients were treated for skull base chondrosarcoma using FRT. The prescribed dose was 64.9 Gy. None of the patients developed recurrence after 5 years.137 In the series from Mayo Clinic, 29 patients who had skull base chordoma (n = 25) and chondrosarcoma (n = 4) were treated using GK-based SRS. All four patients who had skull base chondrosarcoma achieved tumor control with a median follow-up of 4.8 years.134 Similarly, good results were shown in the study from the University of Pittsburgh.136 In a study by Hasegawa et al, those who had tumor volumes < 20 mL showed significantly improved tumor control and survival.153 Iyer et al from Stanford evaluated 22 patients treated with GK-SRS for skull base chondrosarcoma. The 5-year local control and survival rates were 72 and 70%, respectively. Patients who were > 40 years of age and who had no prior radiotherapy showed improved outcomes.154
In a study by Jiang et al, patients were treated using CyberKnife FSRT to a median dose ranging from 22 Gy in a single fraction to 30 Gy in five fractions. FSRT was used for larger tumors. No differences were observed by radiotherapy approach. Tumor control rates in those who had nonrecurrent and recurrent disease were 80 and 50%, respectively. More recently, Kano et al from the University of Pittsburgh published data from the North American Gamma Knife Consortium for skull base chondrosarcomas consisting of seven centers and 46 patients.155 The median tumor volume was 7.8 mL (range 0.9–41 mL), and median dose was 15 Gy (range 10.5–20 Gy). The 10-year local control and survival rates were 70 and 76%, respectively. Patients who had no prior radiotherapy and smaller tumors were associated with improved outcomes. In fact, those who had no prior radiotherapy and tumor volumes < 5 mL had 100% progression-free survival at 10 years.
9.9.2 Particle Therapy for Chondrosarcoma
High-dose proton beam radiotherapy to treat skull base chondrosarcomas has resulted in excellent local control. Several proton beam radiotherapy studies pertaining to management of skull base chondrosarcoma have been reported. A study from Harvard University of 200 patients treated to a prescribed dose of 72.1 CGE showed a 5-year local control of 99%. Other proton beam studies using similar radiation dose levels showed similar tumor control rates.139 , 143
Carbon ion therapy has been used to treat patients who have skull base chondrosarcomas. Compared with proton beam radiotherapy, carbon ion therapy appears to yield similar tumor control and lower toxicity rate. Fifty-four patients were treated for skull base low- or intermediate-grade chondrosarcoma at GSI in Germany. A dose of 60 CGE (weekly dose 7 × 3 CGE) was prescribed to the tumor volume.156 For a median follow-up of 33 months, tumor control rates at 3- and 4-years were 96.2 and 89.8%, respectively. The grade 3 late-toxicity rate was 1.9%. In 2010, Uhl et al reported on their carbon ion therapy experience treating 79 patients who had skull base chondrosarcomas to a median dose of 60 CGE at 3 CGE per fraction. Median follow-up was 91 months, the 5- and 1-year local control rates were both 88%, and the 10-year survival rate was 78.9%. Treatment toxicities consisted of vertigo (20%) and hearing deficits (10%). In this study, primary versus recurrent disease did not impact disease control or survival outcomes. Currently, there is an ongoing randomized clinical trial comparing proton to carbon ion radiation therapy in patients who have skull base chordoma, sponsored by Combs et al from Heidelberg University (ClinicalTrials.gov identifier: NCT01182779).157 , 158 The estimated enrollment is 319, and the primary outcome is local progression-free survival.
9.10 Nasopharyngeal Carcinoma
NPC is the most common head and neck cancer in Southeast Asia but is relatively rare in the Western Hemisphere. In contrast to the NPC phenotype from Asia, patients who have NPC in the Americas are typically diagnosed at a locally advanced stage, and many tumors are negative for the Epstein-Barr virus phenotype.159 The nasopharynx is an anatomically complex site in which a surgical resection of tumor in this region can be challenging and can be associated with significant morbidity. Accordingly, NPC is managed primarily using radiation therapy, with or without chemotherapy. The role of surgery is typically limited to neck dissection if there is residual disease after radiation therapy or to salvage therapy for recurrent disease after primary radiotherapy. The typical radiation dose for gross disease is 70 Gy in 33 to 35 fractions. The typical dose for subclinical disease is 59.4 Gy. Because of this cancer’s tendency to involve the fissures and the foramina in the skull base, these areas should be included in the radiation field. NPC also carries a high risk of bilateral neck node metastases. Even if there is no clinical evidence of nodal involvement at presentation, elective coverage should include the level II through V and retropharyngeal (RP) nodal basins of the bilateral neck.
Multiple phase III trials have established the role of cisplatin-based concurrent chemoradiation followed by adjuvant cisplatin and 5-fluorouracil therapy in the management of NPC.160 , 161 , 162 Locoregional control (LRC) rates in patients treated with concurrent chemoradiation using IMRT are excellent. The predominant pattern of recurrence is distant metastasis. Several meta-analyses have demonstrated that the addition of adjuvant or neoadjuvant chemotherapy to concurrent chemoradiation in those who have locally advanced NPC provides a small but significant reduction in distant metastasis rate while improving OS.163
Nasopharynx cancer treatment has particularly benefited from the rapid evolution of radiotherapy techniques from 2D to 3D-CRT to IMRT and then to proton therapy and MRI-guided radiation therapy. Treatment of NPC initially consisted of conventional 2D radiotherapy (2D-RT) approaches using lateral-opposed fields. This led to satisfactory disease control but high complication rates, particularly for patients who had T3 to T4 disease in which a 72.2 Gy boost to the skull base was used. In the 1990s, 3D-CRT based on CT imaging, which allowed for better target delineation and reduced toxicity to normal tissue, became the standard treatment approach. Because of the complex shape of the target volume and the close proximity of the target volume to various critical structures, IMRT was considered a major advance in the treatment of NPC in terms of improved disease control and reduced toxicity. Randomized clinical studies in NPC treatment have associated IMRT, compared with 3D-CRT, with improved parotid sparing and patient-reported quality of life (QOL) as well as with lower temporal lobe necrosis (TLN) rates.164 The use of IMRT has also been associated with excellent local control rates of > 85% consistently across multiple studies.164 , 165 , 166 , 167 In a recent meta-analysis of eight studies comparing patients treated using IMRT (n = 1,541) to 2D and 3D conventional techniques (n = 2,029), IMRT was associated with better 5-year OS and locoregional control rates as well as lower rates of late xerostomia, trismus, and TLN.168 In a study from the University of California, San Francisco of NPC patients treated using IMRT, the 4-year local progression-free, local–regional progression-free, and distant metastasis-free rates were 97%, 98%, and 66%, respectively.169 The 4-year OS was 88%, and toxicity rate was low. Other studies also showed favorable results after IMRT for NPC.166 , 170
9.10.1 Radiotherapy for Stage T3 to T4 Nasopharyngeal Carcinoma
Patients who have stage T3 disease (tumor invasion of the paranasal sinuses, clivus, and other structures of the skull base) or T4 disease (intracranial extension, involvement of CNs, or extension to orbit or infratemporal fossa) present a clinical challenge for the radiation oncologist. The use of IMRT allows the delivery of an adequate tumoricidal dose with an acceptable toxicity risk. Table 9.5 shows the clinical outcomes of selected series after IMRT for patients who have T4 NPC. Overall, tumor control and survival rates were very good, with acceptable treatment toxicity. Patients from endemic areas represented the majority of studies. The results of non-endemic T4 NPC patients treated using IMRT were reported in a study from the MD Anderson Cancer Center. Takiar et al showed that the results were similar to those for endemic patients.171 The 5-year locoregional, progression-free survival and OS rates were 80%, 57%, and 69%, respectively. The late grade 3 toxicity rate was 49% at 5 years, with hearing impairment accounting for nearly half (43%) of cases. In this cohort, > 80% of patients received high-dose cisplatin. The incidence of TLN was 14%, of which the majority of cases were subclinical, with 15% of patients with radiographic TLN demonstrating a clinically measurable cognitive impairment.
9.10.2 Proton Therapy for Nasopharyngeal Carcinoma
The use of PRT for treatment of NPC is currently under investigation. Dosimetric benefits of PRT over IMRT include decreased beam path toxicity and lower mean doses to the anterior oral cavity, brain, brainstem, and spinal cord without compromise of tumor coverage. PRT can potentially improve radiation-induced nausea and vomiting by delivering lower doses to the area postrema and dorsal vagal complex.179 , 180 Clinical data on use of PRT for NPC are emerging. Massachusetts General Hospital (MGH) presented in abstract form early experience with PRT for NPC, demonstrating a 92% local control rate at 3 years for 19 patients who had T4 disease. Preliminary phase II data from MGH evaluating combined photon–proton therapy using a 3D passive scatter PRT technique with concurrent cisplatin and fluorouracil demonstrated > 90% disease control and low toxicity with short follow-up. Similarly, early clinical data from the MD Anderson Cancer Center using IMPT for NPC indicate excellent tumor control and a low treatment toxicity profile and a low treatment toxicity profile. In a 2:1 case-control study of 13 patients treated with IMPT, when compared to IMRT, IMPT was associated with a significantly lower rate of feeding tube dependence (7.7% vs 19.2%), which is likely attributed to lower oral cavity dose.181 , 182 , 183 , 184
9.10.3 Proton Therapy for Adenoid Cystic Carcinoma of the Nasopharynx
PRT offers a unique advantage in the treatment of patients who have unresectable adenoid cystic carcinoma (ACC) of the nasopharynx. ACC of the nasopharynx typically presents at a locally advanced stage and is not amenable to surgery. ACC is also an aggressive histology that has a reputation for being somewhat radioresistant. Management strategies that have been used for ACC of the nasopharynx have included high-dose radiotherapy with concurrent chemotherapy (Fig. 9.5). The locoregional control rates in small retrospective studies vary from 9 to 66%. Preliminary clinical data from colleagues at MD Anderson Cancer Center in patients with resectable and unresectable ACC after PRT demonstrate promising local control. In 16 patients with resectable ACC who received adjuvant PRT after surgery, Holliday et al showed local control in 15 (93.7%) patients, with a median follow-up of 24.9 months. Bhattasali et al reported on 8 patients with unresectable ACC of the nasopharynx treated with PRT combined with concurrent cisplatin chemotherapy. Four patients achieved a complete response and 4 patients achieved stabilization of local disease. One patient developed local disease progression. There were 5 acute grade 3 toxicity events and 1 grade 4 optic nerve disorder.185 , 186

9.11 Paranasal Sinus Tumor
Paranasal sinus tumors are rare. Histologic subtypes include epithelial tumors such as squamous cell carcinoma, undifferentiated carcinoma, malignant salivary gland tumors (adenocarcinoma, ACC, and mucoepidermoid carcinoma), and esthesioneuroblastoma, melanoma, rhabdomyosarcoma, plasmacytoma, and lymphoma. For lymphoma and plasmacytoma, low- to moderate-dose (30–50 Gy) radiation therapy, given with or without chemotherapy (depending on histology), is the mainstay of treatment. For other histologies, surgical resection should be considered. The role of radiotherapy in the adjuvant setting is often to enhance tumor control in patients who have high-risk pathology features. Typically, a high dose of radiation (60 Gy) is required for these tumors. This presents a therapeutic challenge, because the target volume to be treated is often in proximity to critical structures such as the eyes and the optic apparatus, whose maximum tolerated dose is typically below 60 Gy. The staging for paranasal sinus tumor differs according to the histology. Staging for squamous cell carcinoma of the paranasal sinus is based on the site of origin, according to the American Joint Committee on Cancer, for tumors of the maxillary, ethmoid, or nasal cavity. For esthesioneuroblastoma, by contrast, staging is based on anatomic extension as defined by the Kadish system.
Inoperable tumors of the paranasal sinus represent a challenge because of the need to deliver tumorocidal doses in excess of 66 Gy while attempting to spare adjacent critical neurovascular and optic structures. For gross disease, 70 Gy is recommended. A dose of 66 Gy is typically prescribed for gross disease near critical structures when 70 Gy is not achievable (delivered in 2.0 to 2.12 Gy per fraction). For the clinically negative neck, nodal coverage typically includes the level IB (submandibular) and II (subdigastric) and lateral RP regions. For patients who have a lateralized maxillary sinus tumor, coverage includes the ipsilateral IB and II nodes. For those who have nodal metastases at diagnosis, consideration is given to coverage of the entire unilateral neck (levels I–V) or bilateral neck. For tumors crossing midline, the bilateral neck is typically treated. Coverage of facial and buccal nodes should be considered in those who have tumors that involve anterior structures of the nasal cavity and paranasal sinuses, whereas tumors that involve the posterior regions of the nasal cavity and paranasal sinuses often drain to the RP and deep cervical nodes. Nodal metastasis for carcinomas of frontal and sphenoid sinuses is less common. In addition to elective coverage of first- and second-echelon nodes, subclinical dose coverage of perineural pathways, including the skull base, is critical. Common pathways include CNs VII and V1 to V3.
Highly conformal radiotherapy techniques such as IMRT and PRT are the preferred treatment approach for these cases. Troung et al evaluated proton therapy for primary sphenoid sinus cancers and showed an excellent 2-year locoregional control rate of 86%. Oropharyngeal involvement and anterior cranial fossa invasion served as predictors of poor outcome.187 , 188
9.11.1 Squamous Cell Carcinomas
Squamous cell carcinoma of the sinonasal tract is rarer than at other head and neck subsites. For such patients, surgical resection followed by postoperative radiation therapy appears to be the optimal approach.189 , 190 However, in cases of very advanced disease that renders surgical resection infeasible, primary radiotherapy can be offered. The prognosis in those who have sinonasal squamous cell carcinoma is poor; carcinomas that arise from Schneiderian papillomas or that are associated with transcriptionally active high-risk human papillomavirus may have a better prognosis.191 Despite aggressive therapy, clinical outcomes after conventional radiotherapy remain suboptimal, with 5-year tumor control rates below 50% and 5-year survival as low as 27%.192 The use of highly conformal radiotherapy such as IMRT and GK-SRS can improve local control and reduce treatment morbidity, particularly when the tumor is adjacent to a critical structure. In patients who show no clinical evidence of nodal involvement at diagnosis, high regional nodal failure rates have been reported when elective neck irradiation is not given in those who have squamous cell or high-grade carcinomas.
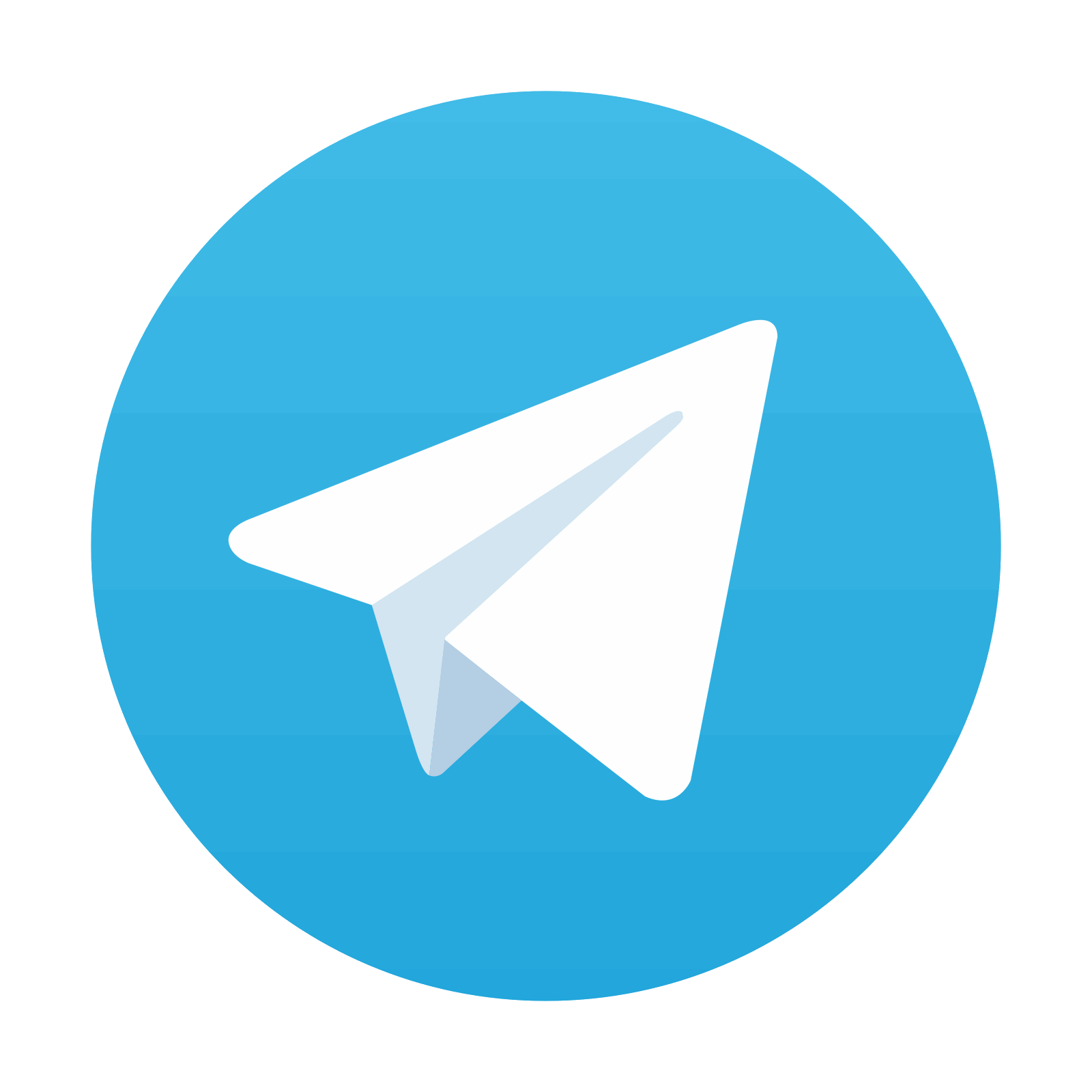
Stay updated, free articles. Join our Telegram channel

Full access? Get Clinical Tree
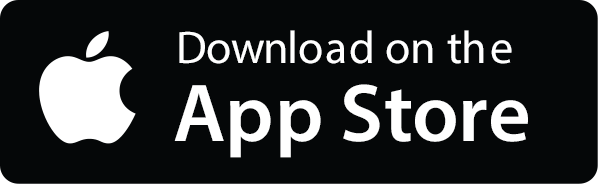
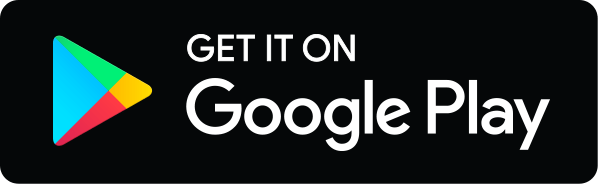
