9 Homeostatic Mechanisms in the Neurosurgical Intensive Care Unit Patient
Abstract
Although the environment of the brain is within a closed box without the ability to infinitely expand, it is completely dependent on the response of other systems of the body to deliver substrate to optimally function and survive. The brain can regulate the flow and pressure of blood, the amount of cerebrospinal fluid, the edema, and the resultant substrate delivery. The changing delivery is in response to the ever changing brain milieu. If the brain’s regulatory mechanisms cannot overcome the microscopic, tissue, or organ environment the neurointensivist should recognize the condition and assist the brain with its ability to heal without causing further damage.
Case Presentation
A 28-year-old, right-handed, Caucasian man had a pituitary macroadenoma resection. Postoperatively, the patient’s Glasgow Coma Scale (GCS) score decreased from 15 to 6. The patient started to have seizures. His temperature was 37.3°C (99.2°F), heart rate 80, respirations 16, and blood pressure 160/90. He was intubated and was transferred to the neurosurgical intensive care unit (NICU). An external ventricular drain was placed and demonstrated intracranial pressure (ICP) consistently above 30 mm Hg and cerebral perfusion pressure (CPP) 70–80 mm Hg. Arterial blood gas showed pH 7.53, pCO2 20 mm Hg, and PaO2 98%. Electrolytes showed serum sodium 129 mEq/L, potassium 3.2 mEq/L, chloride 105 mEq/L, bicarbonate 30 mEq/L, blood urea nitrogen (BUN) 5 mg/dL, creatinine 0.3 mg/dL, and serum osmolality 282 mosm/kg.
See end of chapter for Case Management.
9.1 Homeostasis of the Brain
The Monro-Kellie hypothesis 1 states the skull is a solid box within which the volume of all the components—the brain, cerebrospinal fluid (CSF), and blood—should remain constant. Alteration in pressure in one compartment of the skull is compensated by volume changes in the other. The energy requirements of the brain are met through oxygenation of glucose. In addition, the autoregulation of the cerebral vasculature attempts to maintain adequate perfusion to the brain. 2 , 3 The homeostasis of the brain results from a balance of the foregoing mechanisms.
In the neurologically injured patient, this homeostasis is altered and must be understood because, if not treated, ischemia leading to infarction and death will occur. The treatment of patients in the NICU includes a thorough understanding of cerebral metabolism, intracranial pressure (ICP), cerebral perfusion pressure (CPP), management of fluids and electrolytes, and the correction of these parameters to achieve homeostasis after brain injury (► Table 9.1).
9.2 Cerebral Blood Flow and Perfusion
Cerebral blood flow (50–75 mL/100 g/min) is affected by regional cerebral metabolism, CPP, and oxygen and carbon dioxide tension. 5 Globally cerebral blood flow decreased to one-half normal after injury and reduced even further around contusions, in punctate hemorrhages. It is the lowest with subdural hematoma (SDH), diffuse axonal injury (DAI), and hypotension. There is 3–4% change CBF/mm Hg change PCO2, increases 2–3 days after injury and if lower early worse outcome at 3 and 6 months. One of the main objectives of the neurointensivists is to prevent ischemia. Without blood flow, glucose and glycogen are depleted in 4 minutes. In the ischemic penumbra, also thought of as the “shadow of death” of the infarcted or dead core, there is an increased metabolism attempting to deliver more substrate that results in increased brain temperature. 6 If brain tissue oxygen drops below 20 mm Hg, there is anaerobic respiration, followed by glucose storage-dependent lactic acid accumulation, mitochondrial damage, decreased pH, vasodilation, failure of the Na–K adenosinetriphosphatase (ATPase) pump, and Na and Cl influx followed by water, all of which lead to cytotoxic edema. 2 , 3 Failure in the blood-brain barrier leads to vasogenic edema. Hydrogen ions accumulate and pH drops to 6.0 from 6.4, causing inhibition of mitochondrial respiration and failure to sequester calcium, and, in turn, leading to further cell activation in the face of no substrate, and in turn, the cell dies. Cerebral autoregulation attempts to maintain blood flow according to this or any other change in cerebral metabolism.
Cerebral perfusion is the blood pressure gradient across the cerebral vasculature and usually is equal to the mean arterial pressure minus the intracranial pressure (MAP – ICP). The ability to maintain cerebral autoregulation is affected by pathological conditions, such as hyperventilation, edema, and hypoxia. ICP is controlled through regulation of the intracranial constituents of CSF, blood, and brain volume. The pressure volume index is the ability of the brain to compensate for increased intracranial volume. ICP slowly rises to a point where a slight increase in intracranial volume causes a big change in ICP. When ICP is consistently increased to greater than 20 mm Hg, it may lead to ischemia and herniation, which eventually lead to brain death. The goal in the management of high ICP is to reduce the CSF, blood, and brain volume. This may be accomplished by CSF drainage, prevention of vasodilation, and surgery in the form of a decompressive craniectomy to allow more space for the brain to swell. ► Table 9.2 outlines the basic approach to managing elevated ICP and CPP.
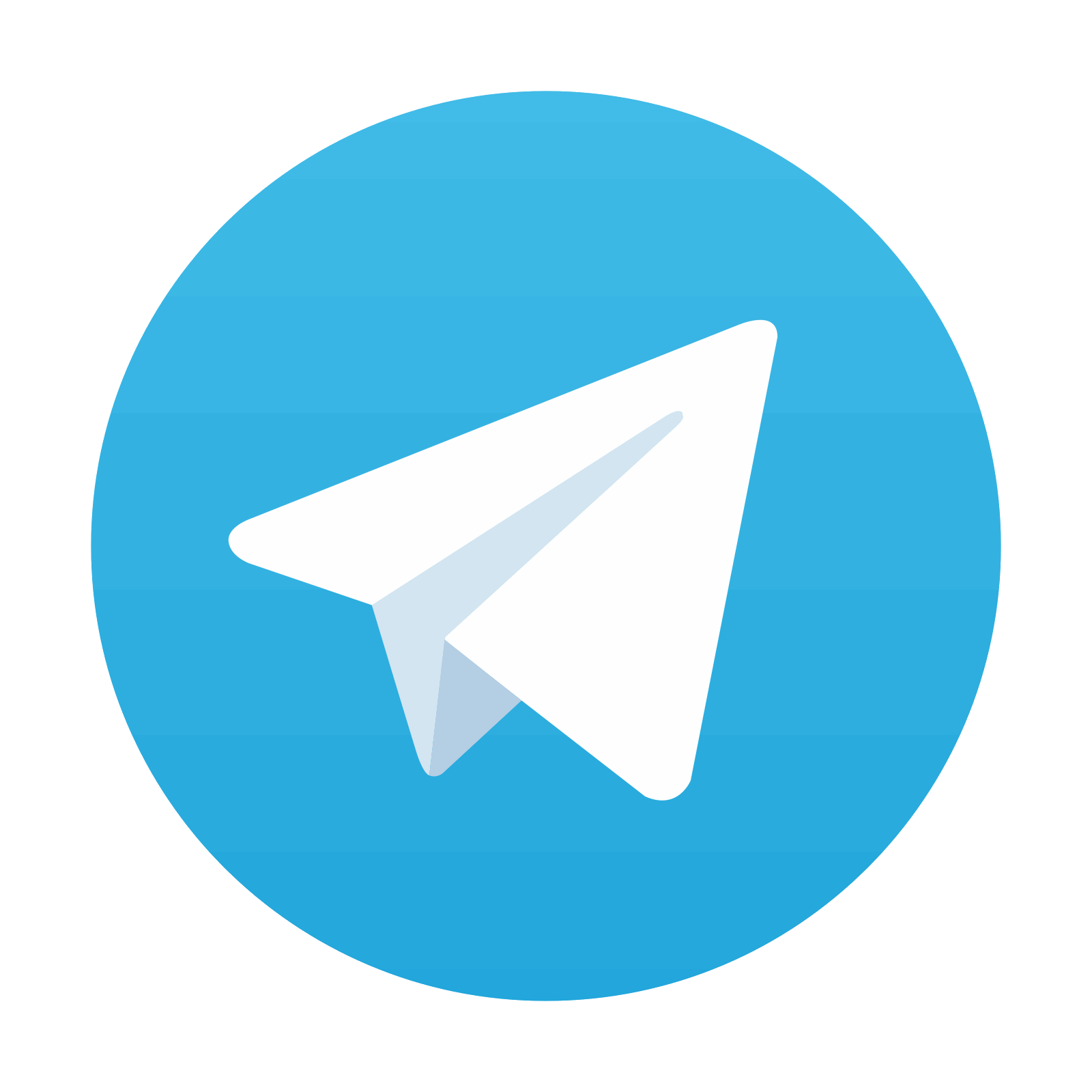
Stay updated, free articles. Join our Telegram channel

Full access? Get Clinical Tree
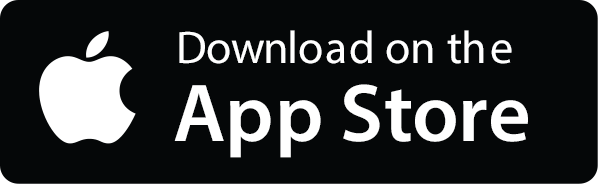
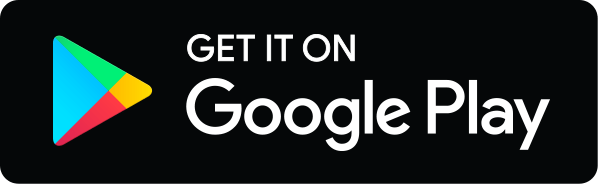