9 Local Stresses: Segmental Mechanism of Low Back Pain and Degeneration, and Stresses According to Spinal Orientation—Contact Forces Theory
Local Stresses: Segmental Mechanism of Low Back Pain and Degeneration
Abstract
The human spine assumes the body verticality compared with quadrupeds. This global vertical orientation of the spine and pelvis induces a permanent stronger action of the gravity on the spinal structures. The normal spine physiology can be compared to the function of a crane that is governed by a tripod of mechanical forces: the anterior downward force of the gravity (weight of the patient), the posterior forces of the muscles to erect the spine that allow walking, and the effect of the belly shape in this system, but only two main forces act in pathology: the weight gravity and posterior muscle force (MF). The total contact force on the spinal unit is the sum of these two acting forces. On the other hand, the distribution of the same vertical force is different depending on the sagittal orientation of the vertebrae. This induces different contact forces orientation is the various spinal shapes. Thus, the identification of various spinopelvic morphotypes allows understanding the physical distribution of forces induced by body weight and muscle counterbalance, regarding spinal unit orientation and range of motion. An abnormal overstress may explain the mechanical origin of pain and spine degeneration.
9.1 Introduction
The spinal column forms the axial skeleton and is a complex multiarticular system that supports the head and the trunk with economical transfer of the loads to the lower limbs. This system is under the control of the central nervous system and the local muscles and allows smooth force transfer without any increase of degenerative changes. While assuring this biomechanical role, the spinal column protects the neural elements (spinal cord, cauda equina, and nerve roots) and vascular elements (vertebral artery). While all vertebrates have a common spinal structure by vertebral unit alignment, the human spine must assume the body verticality compared with quadrupeds in which the spine behaves like a bridge between anterior and posterior legs. In quadrupeds, the thoracic and lumbar spine has almost nothing to support regarding the gravity because of its horizontal position. Their intervertebral disks aligned with their horizontal spine do not suffer direct vertical compression forces from gravity (Fig. 9‑1). The only pressure is produced by horizontal forces during acceleration by the posterior legs. This propulsion force from the posterior legs is transmitted by a very anteverted pelvis through a vertical sacral plateau positioned forward the femoral heads. In opposition to other bipeds like dinosaurs or birds, human bipedalism is characterized by the verticality. The global vertical orientation of the spine and pelvis induces a permanent stronger action of the gravity on the spinal structures. It is of paramount importance to maintain the standing position in the most physically economical and stable system.

Spinal stability was defined by the American Academy of Orthopedic Surgeons as “The capacity of the vertebrae to remain cohesive and to preserve the normal displacements in all physiological body movements.” 1 The spinal stability implies the transfer of power forces between the upper and lower limbs with active generation of forces in the trunk. This allows prevention of early biomechanical deterioration of spine components by reduction of the energy expenditure during muscle action. 2 Another factor of stability is provided by the limited segmental mobility, but a global harmonious spinal flexibility linked to the association of the whole segmental unit. The segmental mobility has been extensively studied for each level of the spine 3 , 4 and is characterized by a small range of motion rarely exceeding 5° to 6°.
This chapter reviews the mechanisms of low back pain generation focusing on contact forces and forces transmission while applying novel findings of spinal alignment on the generation of these forces. Mechanisms of segmental motion overpassing are also analyzed with its possible painful action.
9.2 Historical Review
Even though anatomy of the spine has been quite well described by Hippocrates and then Galen, and very precisely by Vesalius during the 16th Century, it was Giovanni Alfonso Borelli who approached its mechanical function in the book De Motu Animalium. He described the muscles–joint interactions and the forces acting on the spine in various situations (see Chapter 1). Later, Jean Cruveilhier used Euler’s law to demonstrate how successive spinal curvatures favor the spinal resistance. During the 19th century, numerous authors, mainly in Germany, tried to determine the position of the center of gravity and more precisely to define the position of the center of mass at each level of the human body. In 1987, Duval-Beaupère published a technique using a gamma-ray scanner to identify the mass of successive body scans and the position of their respective body mass centers. Based on the notion of spinal unit, many authors (Panjabi, Dimnet) described the intervertebral motion. Recently, using the crane concept, Dimnet defined the combination of different gravity and muscles forces that induce a global contact force on each level.
9.3 Functional Spinal Unit and Normal Range of Motion
The spinal biomechanical unit is the functional spinal unit (FSU). An FSU is formed by two adjacent vertebrae with one intervertebral disk and corresponding ligaments (Fig. 9‑2). 5 The FSU’s main function is to provide spinal movement while protecting the neural elements. Another function of the FSU is to transmit the weight of the body to the lower limbs via the pelvis and the femoral heads. 5 The FSU function relies greatly on the anatomy of its components as well as on the interactions between the bony structures, the surrounding muscles and ligaments, and the control of the central nervous system. 2

In everyday activity, the vertical loads on the spine are thought to be around 500–1000 N, nearly twice the body weight. These compressive forces increase to nearly 5000 N with lifting, achieving nearly half the failure load. 6 The transmission of these loads is assured by the anatomy of the vertebral bodies, the intervertebral disk, and the facets and the sagittal curvatures of the spine. 2
The vertebral body anatomy and architecture plays an important role in the load transmission. The load-bearing ability increases from 200 N in cervical vertebra to around 8000 N in the lower lumbar vertebrae. This is caused by the increasing size of the vertebral body going from the cervical spine to the lumbar spine (C1 has no vertebral body and S1 has the largest vertebral body). This increase of size of the vertebral bodies abides by Wolff’s law: “bones adapt their mass and architecture in response to the magnitude and direction of forces applied to them.” Bony microarchitecture also plays an important role in the load transmission. In normal vertebrae, the trabecular bone is arranged with vertical and horizontal trabeculae. While the vertical trabecular system transmits the compressive forces, the horizontal system decreases the bending forces on the vertical system by transmitting the bending forces to the outer cortical shell. 7 With osteoporosis, there is a rarefication of the horizontal system with elongation of the vertical system. This phenomenon is more marked in the anterior body, thus the prevalence of anterior wedge osteoporotic fractures. 8
The intervertebral disk is another actor in the load transmission. It has the proprieties of both a ligament (tension resisting) and a synovial joint (compression resisting). The orientation of the fibers of the annulus fibrosus limits the movements of the FSU in axial (limits rotation), sagittal (limiting flexion, extension), and coronal planes (limiting side bending). On the other hand, the nucleus pulposus acts as a spacer between the two endplates and as a shock absorber and load transmitter. It is interesting to note that the same unit has very different functions in quadrupeds compared to humans. In quadrupeds, intervertebral disks are used for motion stabilization and for propulsion forces transmission but no gravity support, whereas in humans, gravity loads are the main constraints the FSU has to sustain. This may explain the apparent disk fragility in humans who develop more diskopathies than quadrupeds.
The final load-transmitting components are the articular facets. The articular facets contribute to the posterior column (in the Denis model) and acts primarily counteracting shear forces. Similar to vertebral bodies, the shape, size, and orientation of the facets change from C1 to S1 to accommodate the increasing load transmission requirements. Classically, it has been stated that for a normal spine, the intervertebral disk receives more than 90% of the loads. There is concern on the definition of a normal spine. Contemporary studies have shown that there are various spine shapes from more straight (or less curved) to more curved and that the stress/load bearing is divided between disk forward and facets backward, depending on the sagittal orientation of the FSU. With increasing disk degeneration, causing loss of disk height, the loads are transmitted via the posterior facets that receive more than half of the compressive loads. This induces facet joints hypertrophy and osteoarthritis.
As the disk acts as a synovial articulation, it could be compared to another osteoarticular articulation as, for example, the knee or the elbow. Every joint has a normal range of motion that, when beyond it, movement is painful (hyperextension and hyperflexion). This principle is applied to an FSU albeit with a lesser range of motion (5°) compared to the elbow. With the degenerative cascade, the range of motion is ultimately decreased by degenerative changes. Disk degeneration limits flexion and brings the hyperflexion threshold closer to the average position, whereas facet joints arthritis limits hyperextension and brings the hyperextension threshold close to the average position (Fig. 9‑3). When the FSU is in a hyper lordosis state, the remaining possibility of increasing extension is limited. In this kind of structure (i.e., type 1 lordosis), an increasing thoracolumbar kyphosis induces a painful caudal compensation by increasing the distal lordosis in an already much-extended area. As the painful caudal position in extension is very close to the neutral posture, an excessive postural lordosis such as supine with knees extended may be quickly intolerable. If hyperextension is needed (like in osteoporotic fracture in the thoracolumbar area in type 1 lordosis), the hyperextension is painful and generates low back pain and there is increased risk of facet hypertrophy (Fig. 9‑4). Therefore, the back pain of these patients is relieved by lying on the side and rounding the back. On the other hand, an FSU in hypolordosis has the average positioning closer to a hyperflexion threshold. This phenomenon is seen is type 2 spines where the patient may adopt a lumbar kyphosis alignment as a result of multilevel diskopathies. Back pain is generated by the inability to escape the hyperflexion zone and the patients are relieved by lying in a hyperextended position (Fig. 9‑5). In addition, different degenerative mechanisms would also modify this range of motion of the FSU (Fig. 9‑6).




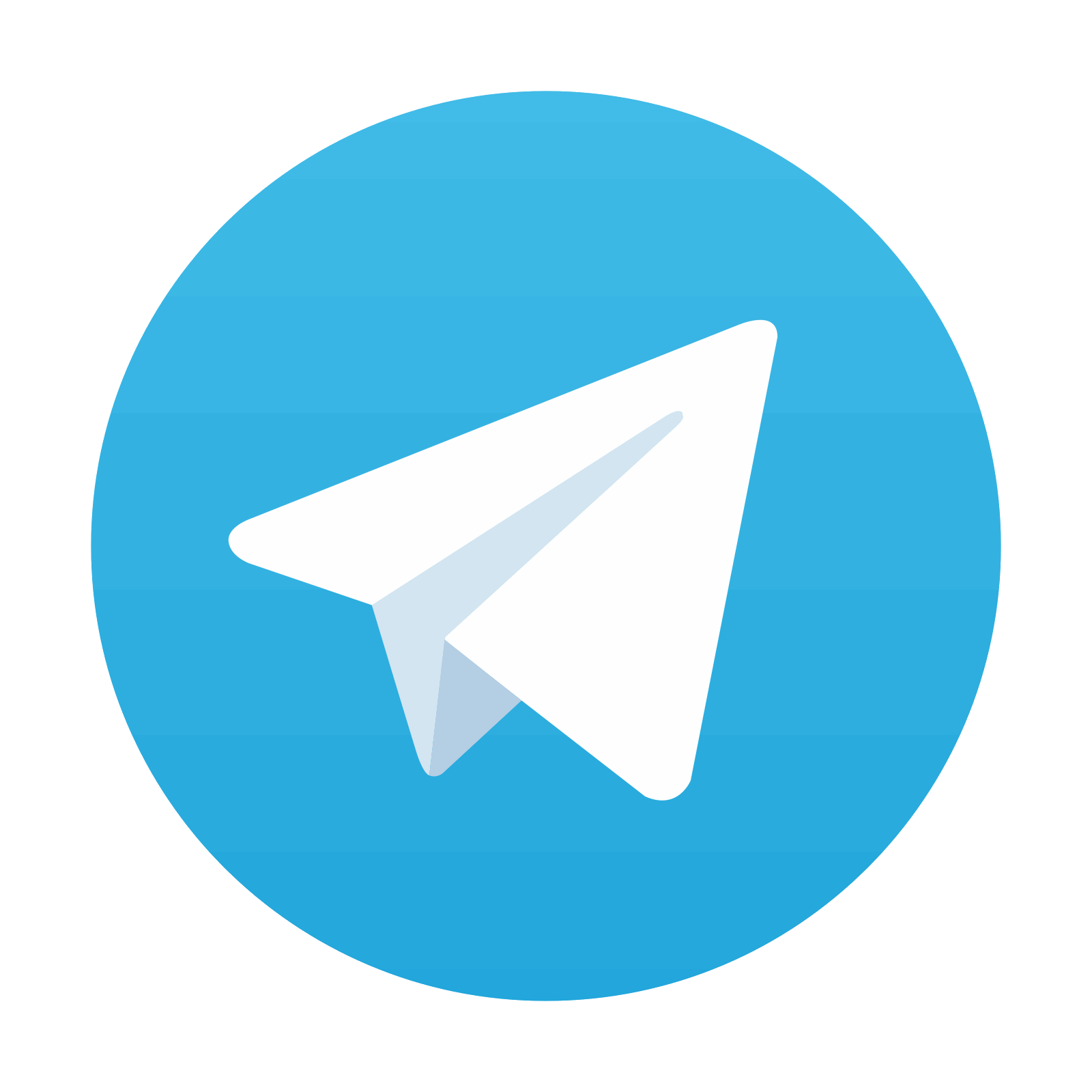
Stay updated, free articles. Join our Telegram channel

Full access? Get Clinical Tree
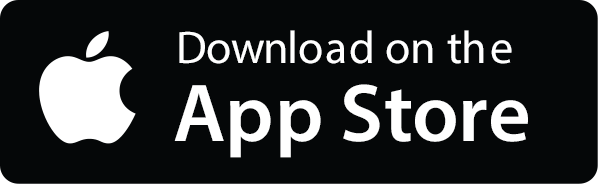
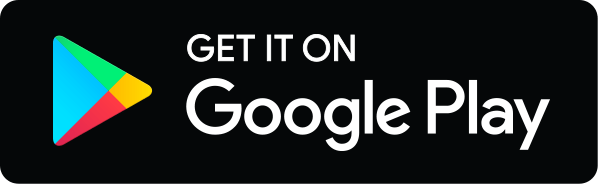