Abstract
Traumatic brain injury (TBI) and traumatic spinal cord injury (SCI) are serious neurologic injuries with potentially devastating impact on patients, caregivers, and society. The pathophysiologic mechanisms of these injuries are complex. While the primary injury is likely associated with irreversible deficit, increasing research and better treatment protocols aimed at mitigating secondary injury cascades has led to consistent progress in taking care of this critically ill patient population.
9 Trauma
9.1 Acute Spinal Cord Injury
9.1.1 Introduction
Spinal cord injury (SCI) is an increasingly common problem posed to both neurosurgeons and neuro-intensivists, with an estimated incidence of up to 0.05%, with about 10,000 to 15,000 SCI occurring each year in the United States alone. 1 , 2 , 3 The consequences can be devastating, with long-term clinical, functional, and financial burdens imposed on providers, care-takers, and, of course, the patient. Managing SCI patients requires a general understanding of the involved physiology, which includes:
Primary injury: The result of direct tissue damage to the spinal cord, either from penetrating or blunt trauma, that causes irreversible tissue damage via direct compression, distraction, or laceration of the spinal cord. 4
Secondary injury: Damage to potentially salvageable tissue caused by inflammation, hypoperfusion, thrombosis, edema, and accumulation of toxic metabolites and reactive oxygen species. 5
Given that the underlying primary insult causes irreparable tissue damage, the bulk of research in the field of SCI has been directed toward modulating and reducing secondary injury cascades. This can be accomplished via medical and surgical interventions.
9.1.2 Medical Treatment of Acute SCI
Medical management of SCI begins at the scene with strict spine precautions, immobilization on a hard backboard, and placement of a hard cervical collar. Acute SCI is often caused by high-energy mechanisms with potentially unstable injuries, and particular attention must be provided to avoid causing or worsening a SCI during patient transfer and transport. Upon arrival at an appropriate trauma center, standard Advanced Trauma Life Support (ATLS) protocols should be followed to identify all injuries. Particular attention should be paid to maintenance of the airway and adequate respiration/ventilation. This is especially true in cases of cervical SCI, as such patients may have significant neck soft-tissue edema that places their airway at high risk for progressive compromise in a manner that may make urgent intubation increasingly difficult. Injuries above the C5 level are additionally at high risk for respiratory failure given the impaired or absent innervation to the diaphragm. As such, there should be a very low threshold for intubation to ensure adequate respiratory function. 6 , 7
The next most important consideration in acute SCI patients is aggressively supporting the systemic circulation. The traumatized central nervous system often displays significantly dysfunctional vascular autoregulation, and tissue perfusion is often directly dependent on mean arterial pressure (MAP). Avoidance of hypotension is critical, with many centers pursuing a prolonged (e.g., 5–7 days) course of artificially elevated MAP (>85–90 mm Hg) in an effort to ensure adequate perfusion of any potentially salvageable ischemic penumbra in the traumatized spinal cord. This often requires the administration of pressors to achieve this goal, especially in patients with neurogenic shock due to loss of sympathetic tone to the vasculature. This is common after cervical and high thoracic injuries, and often manifests as hypotension without an appropriate tachycardic response (i.e., bradycardia or an inappropriately normal heart rate). The data in the literature in support of this protocol is unfortunately of low quality, but the available data from two prospective studies support this elevated MAP goal in an effort to maximize neurologic recovery. 8
The spine is stabilized, a detailed neurologic examination is critical. This is often performed according to the standards of the American Spinal Injury Association (ASIA) scale. This takes into account strength in 10 core myotomes, as well as light touch and pinprick sensation in all dermatomes including sacral, and is graded A–E (Table 9‑1). Accurate ASIA classification is essential as there is prognostic significance and it forms the baseline for monitoring future recovery.
Use of steroids in the setting of blunt trauma to the spinal cord has been a topic of debate in the literature. The NASCIS 9 , 10 , 11 trials attempted to establish the role of steroids, specifically methylprednisolone, in SCI with the results suggesting benefit when used within 8 hours of injury, for a duration of up to 48 hours. However, neurologic recovery gained in follow-up periods was modest at best, and the use of high-dose steroids in this setting is maintained cautiously as an option but with the caveat that the evidence is more convincing for adverse effects, including death, than it is for significant clinical benefit. There are a variety of other pharmacologic agents being studied in myriad clinical trials, but these are used only in carefully constructed research protocols and none have reached standard clinical practice as yet.
9.1.3 Surgical Management of Acute SCI
Many patients with SCI require surgical intervention for stabilization of unstable spinal column injuries and/or decompression of neural elements, with an added benefit being early mobilization and avoidance of prolonged periods of bedrest that are associated with complications such as pneumonia, deep venous thrombosis, pulmonary embolism, and general deconditioning. The exact timing of surgery for SCI has been an area of debate. Several animal studies have shown that earlier decompression of the spinal cord leads to improved neurologic outcomes, presumably as a result of improved tissue perfusion and reduced secondary injury cascades. 12 , 13 This bench research led to optimism that early decompression would clearly benefit actual SCI patients, and led many surgeons to support earlier (originally defined as <72 hours after injury) rather than later surgery for decompression and stabilization. However, early studies did not necessarily support this hypothesis, with one review of incomplete cervical SCI showing no direct correlation between early surgery and functional recovery, 14 and another review of about 800 patients who underwent early, late, or no surgery showing no difference in recovery or functional improvement between the early and late surgical groups, and in fact at 1-year follow-up noted motor improvements were more likely in the nonsurgical cohort. 15 A high degree of variability in defining “early” versus “late” surgery was thought to be a factor in this lack of clinical benefit. A follow-up systematic review, using <24 hours as the cutoff for “early surgery” found significant benefit for early surgery, which led the Spine Trauma Study Group to adopt this threshold in its clinical guidelines. However, the quality of the evidence remained relatively poor. Several small studies have noted better neurologic recovery in those operated on early (<24 hours after injury), as well as fewer complications related to reduced length of stay and bedrest. 16 , 17 , 18 , 19 In 2012, the first multicenter, prospective cohort study evaluated early versus late surgery for acute cervical SCI, with 19.8% in the early surgery group versus 8.8% in the late surgery group showing at least a 2 grade improvement on the ASIA scale, and the benefit remained significant when adjusted for administration of steroid protocols and the degree of neurologic impairment. 20 Subsequent systematic reviews on the issue have noted marginal benefits in favor of early surgery, noting that the strength of the evidence remains relatively poor. 21 , 22 , 23 At this time, most centers continue to favor early (<24 hours from injury) surgery for decompression and stabilization of acute spinal cord injuries, especially for incomplete injuries, in order to maximize their potential for neurologic recovery as well as to support early mobilization and rehabilitation and reduce complications associated with prolonged bedrest.
9.2 Traumatic Brain Injury
9.2.1 Introduction
Traumatic brain injury (TBI) is an unfortunately common event in the developed world, and often cited as one of the leading causes for mortality and long-term disability in young patients. Sources have quoted 2.5 million cases of TBI per year and 50,000 to 100,000 deaths annually in the United States alone, with associated costs approaching $100 billion. The leading cause of these injuries includes falls, motor vehicle accidents, and assault. 24 , 25 , 26 Not only are these injuries common, but the pathophysiology is extremely complex. With the emergence of neurocritical care as a distinct medical subspecialty over the past several decades, the progress in treating this challenging patient population has been slow but steady. An understanding of the mechanisms of injury and appropriate medical care are essential to maximize outcomes in this difficult patient population. 27 Classification of TBI is commonly based on the Glasgow coma scale (GCS) score, shown in Table 9‑2. This classification is useful because outcomes in terms of morbidity and mortality correlate with this classification, and it provides a simple framework that allows quick communication between care providers across multiple disciplines and specialties. With that in mind, it should be understood that the GCS is a very simple classification scheme, and that injuries to the central nervous system can be quite different despite an identical GCS score. Thus, further understanding the mechanisms and pathophysiology behind TBI is critical. 27 Key to this is the difference between primary and secondary injury.
Primary injury: Direct tissue injury related to the force of impact. The injury can be blunt or penetrating, with resulting injuries either focal (e.g., subdural hematoma or parenchymal contusion) or diffuse (e.g., diffuse axonal injury) and result in largely irreparable tissue damage.
Secondary injury: Progressive injury to surrounding brain parenchyma as a result of functional and metabolic derangements in the traumatized brain. This represents tissue that can potentially be salvaged by appropriate neurocritical care protocols. Examples include: 27 , 28 , 29
Loss of cerebrovascular autoregulation
Abnormal neuronal circuitry with imbalance in excitatory/inhibitor throughput
Metabolic derangements
Historically, the two most important parameters associated with expanding secondary injury have been hypotension and hypoxia. 30 , 31 Commonly quoted thresholds to maintain are systolic blood pressure >90, and Pa O2 >60 mm Hg. Treatment aimed at avoiding falling below these values should be aggressive in all phases of care, including pre-hospital, as even transient episodes below these thresholds have been associated with worse functional outcome and higher mortality.
Arrival at an appropriately equipped trauma center begins the intense hospital phase of TBI care. ATLS protocols should be strictly adhered to. Systemic injuries should be evaluated and the patient stabilized efficiently, with particular attention paid to blood pressure and oxygenation. Of note, aggressive hyperventilation should not be pursued prophylactically, as this may lead to severe vasoconstriction and worsening ischemic damage to at-risk penumbra. 24 Home medications and lab values should be reviewed, particularly for anticoagulant and antiplatelet medication. Once stable, a noncontrast computed tomography (CT) scan of the head should be performed and reviewed immediately for the presence of hematomas that warrant surgical evaluation. Guidelines have been published to help streamline decision-making. While each case is unique, in general acute subdural hematomas associated with clot thickness >10 mm, midline shift >5 mm, and/or abnormal pupillary function should be evacuated. An epidural hematoma with clot volume >30 mm3, midline shift >5 mm, and/or abnormal pupillary function should be strongly considered for evacuation regardless of GCS. Parenchymal hematomas >50 mm3 should always be considered for decompression, and the threshold for surgery is lower for lesions in the temporal lobe or associated with significant midline shift of basal cistern effacement. Posterior fossa hemorrhages should prompt a very low threshold for suboccipital craniectomy for clot size >3 cm, distortion of the fourth ventricle, obstructive hydrocephalus, and basal cistern or brainstem mass effect.
In surgical patients, the threshold for leaving the bone flap off (i.e., craniectomy) should be low, especially for acute subdural hematomas, which are often associated with more severe parenchymal injury and swelling compared to an epidural hematoma. 24 , 26 , 32 This is being further evaluated by the RESCUE-ASDH trial, results of which are not yet published. 33 Additionally, in the setting of intracranial hemorrhage, antiplatelet and anticoagulant agents should be reversed. This may require consultation with a hematologist to discuss reversal options for some newer anticoagulant agents.
Upon completion of an ATLS evaluation and with no emergent operative interventions planned, the patient should be quickly transferred to a neuro- or surgical intensive care unit (ICU). Invasive monitoring, usually with arterial and central venous lines, is often indicated. Aggressive avoidance of hypotension and hypoxia is the rule. With that in mind, all administered fluids should be isotonic or hypertonic, as hypotonic fluids may exacerbate cerebral edema due to blood–brain barrier disruption in the setting of TBI. Further, blood transfusion thresholds higher than 7 g/dL showed no benefit at 6 months in a randomized trial. 34 Historically, an intracranial pressure (ICP) monitor is placed in those with GCS <9 and an abnormal head CT. However, due to low quality evidence, the most recent guidelines make no statement on selecting patients for ICP monitoring, or on open versus continuous cerebrospinal fluid (CSF) drainage, and they stress that the clinical judgment of treating physicians should select which patients are at risk for elevated ICP. 25 , 35 Although the BEST TRIP study suggested severe TBI patients could be successfully managed without ICP monitor placement, the accepted practice in many severe TBI patients is to directly assess ICP. 31 Options for this include the following:
Epidural and subdural monitors
Direct parenchymal monitors
Intraventricular monitor (aka external ventricular drain or EVD)
EVD placement is often considered the gold standard due to the ability to both monitor ICP and therapeutically drain CSF as needed; however, all of the above options provide acceptable ICP measurements. 26 Additionally, ICP monitors allow for calculation of cerebral perfusion pressure (CPP), by the formula:
CPP should be maintained between 50 and 70 mm Hg to ensure adequate metabolic supply to the brain. Ancillary invasive monitoring techniques, such as jugular bulb oximetry, direct brain tissue oxygen monitors, and microdialysis catheters, also exist, although discussion of these is beyond the scope of this chapter. 26 , 36 , 37 , 38 , 39
Category | Behavior | Score |
Eye opening | Spontaneously | 4 |
To command | 3 | |
To painful stimulus | 2 | |
None | 1 | |
Verbal | Oriented | 5 |
Confused speech | 4 | |
Inappropriate speech | 3 | |
Incomprehensible | 2 | |
None | 1 | |
Motor | Follows commands | 6 |
Localizes pain | 5 | |
Withdraws to pain | 4 | |
Decorticate/flexor posturing | 3 | |
Decerebrate/extensor posturing | 2 | |
None | 1 | |
Mild TBI: 13–15, Moderate TBI: 9–12, Severe TBI: 3–8 | ||
Abbreviation: TBI, traumatic brain injury. |
9.2.2 Management of Elevated ICP
Prolonged elevated ICP is associated with worse outcomes. 25 , 40 , 41 Thus, timely management is critical. Any acute change in ICP should prompt an urgent head CT to evaluate for surgical lesions and the degree of parenchymal edema. If an external ventricular drain is placed, CSF should be drained to bring ICP <20 if possible. Medical management of elevated intracranial pressures should proceed in stepwise fashion, and include:
Patient positioning
Head of bed elevated by >30 degrees
Head midline position
Ensure hard cervical collars are not too tight and avoid neck kinking to optimize venous outflow
Adequate pain control
Adequate sedation to decrease metabolic demand of brain parenchyma with optimization of blood pressure for cerebral blood flow
Use of hyperosmolar therapy can be used to reduce intracranial pressure in severe TBI patients 42 , 43
Assess serial bladder pressures when appropriate for early identification of abdominal compartment syndrome 24
Further details on the medical management of elevated ICP can be found in Chapter 6. For the surgical management in severe TBI, there have been two prospective, randomized clinical trials evaluating the role of decompressive craniectomy. 44 , 45 The consensus is that while ICP is likely reduced with craniectomy, this comes at a high risk of poor long-term functional recovery, and frank discussions with family members is required to make sure that expectations are reasonable prior to pursuing this treatment modality. Given the lack of clarity in the data, the most recent Brain Trauma Foundation Guidelines only recommend that if craniectomy is pursued, a large decompressive hemicraniectomy should be pursued compared to a smaller flap, with the anterior-posterior dimension often quoted as 12 to 15 cm. 35 Another consideration in the treatment of ICP is hypothermia, either on a prophylactic basis for neuroprotection after severe TBI or as a therapy for refractory ICP elevations. Two recent reviews on the topic suggested reduced mortality and poor neurologic outcome in patients with severe TBI treated with hypothermia, but there was significant heterogeneity in a variety of therapeutic parameters and much of the data was of relatively low quality leaving the authors to suggest its use only in carefully constructed research settings. 46 , 47
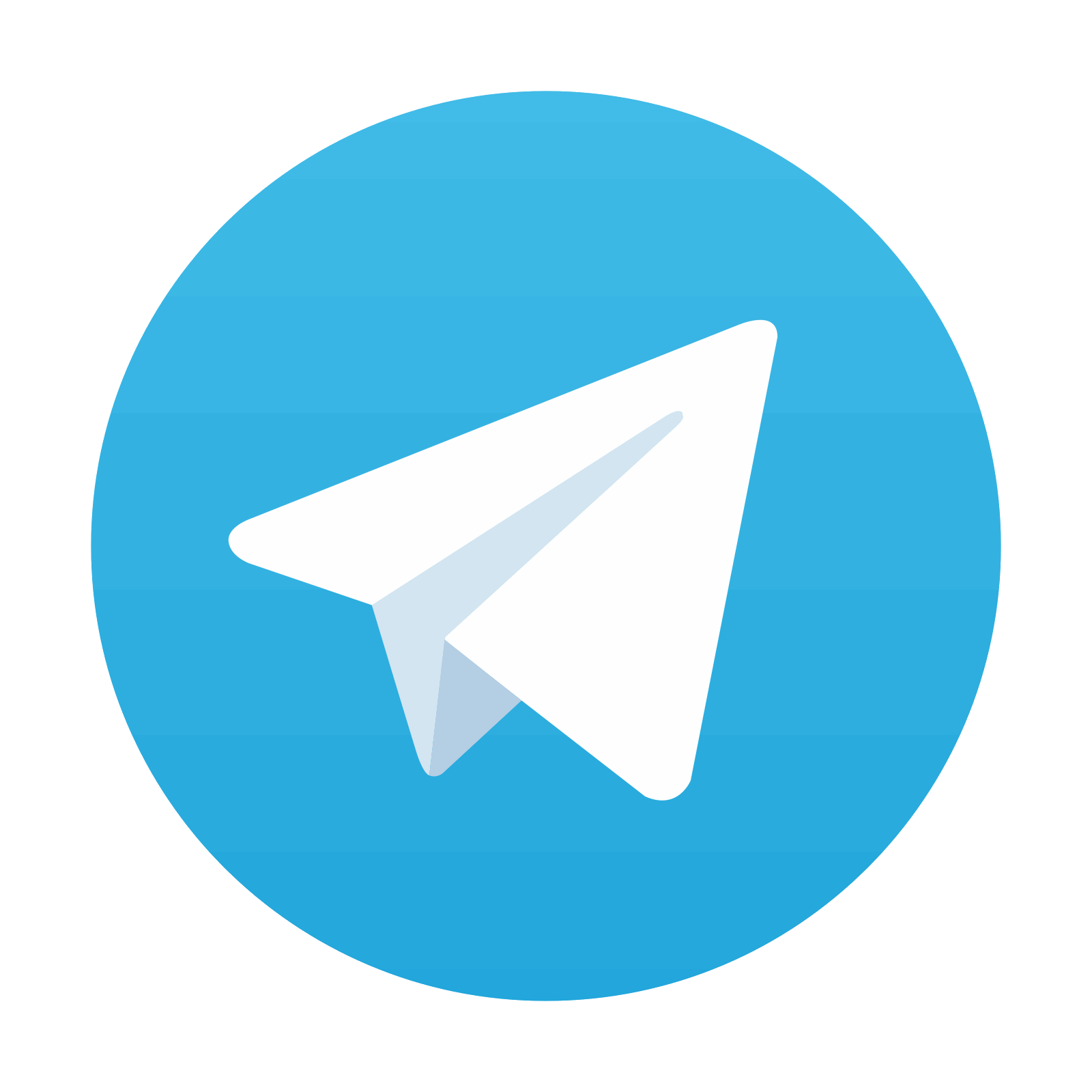
Stay updated, free articles. Join our Telegram channel

Full access? Get Clinical Tree
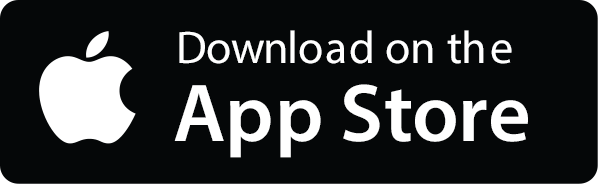
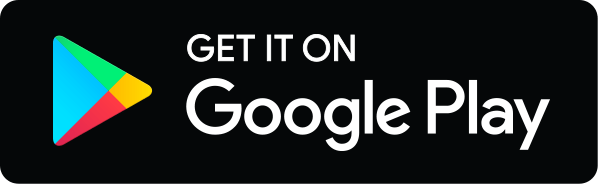
