Fig. 1
Lysosomal enzymes involved in the catabolism of GM1 ganglioside to GM3 ganglioside and the lysosomal storage disorders associated with loss of each enzyme
Adeno-associated virus is a replication-deficient parvovirus. The single-stranded 4.7 kb DNA genome is composed of the rep and cap genes flanked by inverted terminal repeats (ITR). The AAV virion is a non-enveloped 20 nm capsid composed of three viral proteins (VP) VP1, VP2, and VP3 at a 1:1:18 ratio (60 capsomeres total). Wild-type AAV is prevalent in humans, but to date, there are no reports linking it to any disease [54]. Recombinant AAV (rAAV) vectors are devoid of viral coding sequences and carry an ITR-flanked transcription cassette consisting of a promoter driving expression of a gene of interest and a polyadenylation signal (polyA) (Fig. 2). The ITR elements are necessary for vector genome replication and packaging during production and postinfection processing to generate stable transcriptionally active genomes in target cells.


Fig. 2
Genomic organization of recombinant adeno-associated viral vectors
Recombinant AAV has become the platform of choice for in vivo gene delivery to the CNS for a number of reasons including its ability to transduce dividing and nondividing cells at high efficiency, mediate long-term gene expression, and maintain an excellent safety profile both in animal models and human clinical trials. However, the immunology of AAV-encoded transgenes after gene transfer to the CNS is an evolving field, and it appears that new more powerful capsids, such as AAV9, are also more likely to trigger immune responses than less robust AAV serotypes, such as AAV2, when encoding either nonmammalian transgenes (GFP) or species mismatched therapeutic genes [55]. Most experiments in mouse models of LSDs have been conducted with AAV vectors encoding human enzymes. In some animal models, the therapeutic efficacy appears to be compromised by the immune system [56, 57], and it is unclear whether this is due to an immune response to a species mismatched enzyme or because of the knockout nature of most mouse models in which no protein is detectable. This aspect should be considered carefully in the planning of preclinical efficacy and safety experiments in large animal models as the use of AAV vectors encoding human enzymes can confound the interpretation of outcomes [58]. Although the degree of homology between human and other mammalian enzymes (especially monkey) is very high, there may be sufficient amino acid differences to generate new epitopes that may trigger an immune response. The approach that is often employed to test AAV vectors encoding human enzymes in large animals is to use immunosuppression [59, 60]. For obvious reasons, this can blunt or mask responses that would otherwise become apparent and possibly limiting to the implementation of a particular vector design and/or delivery route. Finally, the amino acid differences between enzymes from different species could alter protein–protein interactions in subtle ways and influence both therapeutic outcomes and toxicological profiles. For these reasons, we have used AAV vectors encoding species-specific enzymes in our preclinical efficacy and safety studies.
The most commonly used promoters to drive gene expression in AAV vectors are ubiquitous, strong promoters such as chicken beta actin (also known by the designations of CBA, CAG or CB), in which the promoter is fused to the human cytomegalovirus immediate early gene enhancer. For applications in CNS where neuronal restricted expression is desired, the human synapsin-1 promoter appears to be quite effective [61]. Despite its large size (1.7–1.8 kb), the promoter for glial fibrillary acidic protein (GFAP) can be used to restrict expression to astrocytes. Though the majority of AAV capsids display a high degree of neurotropism in rodent brain, a few serotypes such as AAV5 transduce astrocytes and neurons equally well [41, 62, 63]. The vast majority of AAV vectors carry ITR elements from AAV2, but nonetheless they can be packaged at high efficiency in a variety of AAV capsids [64]. Many types of AAV have been identified that efficiently transduce the CNS such as AAV2, AAV1, AAV5, AAV8, AAV9, AAVrh8, and AAVrh10 [41, 65–73]. Direct intracranial infusion of recombinant AAV vectors has proven its effectiveness in a large number of small and large animal models for different LSDs, and it is currently being tested in clinical trials using AAV2 and AAVrh10 for Batten disease and MPSIIIa [74–76]. Preclinical work for MPS I and MPS III using AAV5 showed promising results in dog models [77]. Nonhuman primates, such as cynomolgus macaques, are commonly used to study the safety of AAV administration and the technical protocols that will be used for clinical trials [19, 60, 78–82].
This chapter will detail the techniques used for stereotaxic infusion of AAV vectors into adult mice and intracerebroventricular infusion in neonatal mice, followed by stereotaxic injection in cats, sheep, and monkeys.
2 Infusion of AAV Vectors into the Mouse Brain
2.1 Stereotaxic Injection into Adult Mouse Brain
Mouse models have been created that mimic phenotypes of patients for GM1 gangliosidosis (β-galactosidase knockout) and Sandhoff disease (hexosaminidase β-subunit knockout). A mouse model of TSD has been generated (hexosaminidase α-subunit knockout), but these mice do not show the neurological phenotype seen in patients due to an alternative catabolic pathway for GM2 ganglioside in mice. In contrast, Sandhoff disease (SD) mice develop severe symptoms early in life (~100 days of age) and succumb to disease progression by ~125 days of age. Therefore, SD mice are commonly used in gene therapy studies for GM2 gangliosidoses (TSD and SD) [51–53]. The most successful and common technique used to deliver AAV vectors to the CNS of adult mice is through direct infusion into the brain parenchyma, and its effectiveness has been demonstrated by both improved survival and behavior in a multitude of mouse models for LSDs with nervous system involvement [45, 47, 74, 83]. Gene transfer to the CNS in mice and humans was first achieved using rAAV2 serotype [84]. Since then, other more efficient serotypes for CNS gene transfer have been utilized such as rAAV1, rAAV5, rAAV8, rAAV9, rAAVrh8, and rAAVrh10 [41, 65, 66, 69, 71]. Most recently, rAAV9 and other capsids have been shown to efficiently transduce the CNS through systemic delivery via intravenous injection in adult mice and provide another potential approach for gene therapy for lysosomal storage disorders [67, 72, 73].
2.1.1 Materials and Reagents
Stereotaxic instrument; Stoelting 51730D
Injector; World Precision Instruments; UltraMicroPump III
Syringe pump controller; World Precision Instruments #SYS-MICRO4
Gastight syringe, 10 μL*; Hamilton #7653-01
33 gauge steel needles*; Hamilton #7762-06
Sterile 0.5 M sodium hydroxide
Sterile water
1 mL syringes with permanent needle; Kendall Monoject #1188128012
Ketamine (100 mg/mL)
Xylazine (20 mg/mL)
0.9 % sodium chloride; Hospira #0409-4888-10
Puralube® ophthalmic ointment; Webster #07-888-2572
Small electric shaver
70 % isopropyl alcohol prep pads
Povidone–iodine prep pads
Sterile disposable scalpels; Dynarex #4111
Sterile cotton swabs; Puritan #25-806 1WC
Small drill bits*
Handheld drill
Stereotaxic atlas
Sterile AAV vector to be injected
Reflex™ clip applier*
Reflex™ clips, 9 mm*
Reflex™ clip removing forceps*
Warm water recirculation pump
Warm water circulation pad
Ketoprofen (100 mg/mL)
*Autoclave before use
2.1.2 Protocol
1.
Set up and connect stereotaxic instrument with injector and syringe pump controller according to manufacturer’s instructions (see Fig. 3).
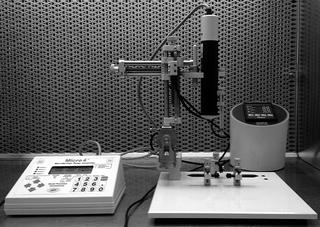
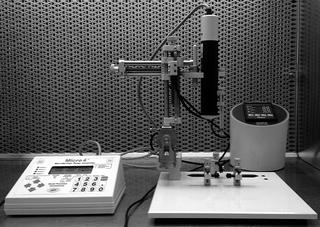
Fig. 3
Stereotaxic frame and injector setup for intracranial injections in adult mice
2.
Attach autoclaved needle to autoclaved syringea.
3.
Prepare a sterile and fresh mix of anesthesia with ketamine (10 mg/mL final concentration) and xylazine (1 mg/mL final concentration) using sterile 0.9 % saline to dilute.
4.
Anesthetize mouse by intraperitoneal injection of ketamine/xylazine mix (100 mg/kg mouse dose and 10 mg/kg mouse dose)b.
5.
Apply sterile ophthalmic ointment to the eyes to prevent drying of corneas.
6.
Shave the top of the head using a small electric shaver.
7.
Clean the shaved area with a 70 % isopropyl alcohol prep pad followed by a povidone–iodine prep pad. Repeat three times.
8.
Place mouse onto stereotaxic instrumentc.
9.
Make a longitudinal incision big enough to expose the bregma (Fig. 4) and desired target regiond.
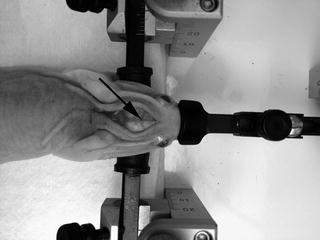
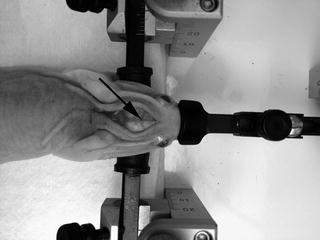
Fig. 4
Adult mouse on stereotaxic frame with longitudinal cranial incision. Arrow indicates location of bregma
10.
Open the incision by pushing the skin aside using sterile cotton swabs, and clean off the periosteum from the skull. Allow skull to drye.
11.
Load needle and syringe with sterile AAV vector preparation by handf.
12.
Place needle into injector and stereotaxic instrument according to manufacturer’s instructions.
13.
Align the needle with the bregma on the mouse skull by moving the stereotaxic manipulator arms in the X, Y axes (see Fig. 4 for location of bregma)g.
14.
Set bregma coordinates to zero on all axes on the digital coordinate display.
15.
Position needle above target structure location using the x and y coordinates by adjusting x and y manipulator armsh.
16.
Drill a small burr hole (<1 mm) using a handheld drill with gentle pressure until the skull is breached without damaging the dura mater or brain parenchymai.
17.
Position needle at the top of the skull just above the burr hole, and set z coordinate to zeroj.
18.
Insert needle slowly into the brain parenchyma to the appropriate z coordinate for target structure.
19.
Wait for 2 min before starting the infusion.
20.
Infuse AAV vector solution at a rate not exceeding 0.2 μL/min.
21.
Wait for 5 min after the infusion is completed before withdrawing the needle to prevent backflowk.
22.
Slowly withdraw the needle.
23.
Remove mouse from stereotaxic frame.
24.
Close incision using sterile wound clipsl.
25.
Place mouse in an empty cage on a heated circulating water pad and monitor recoverym.
26.
Administer ketoprofen (5 mg/kg dose), freshly diluted with sterile 0.9 % sodium chloride, subcutaneously after surgery and once a day for two days post surgery. Alternatively, use the analgesic drug and duration recommended by the local Institutional Animal Use and Care Committee (IACUC).
27.
Administer 500 μL of sterile 0.9 % sodium chloride subcutaneously after surgery and once a day for two days after surgery. Monitor mouse weight and behavior. Check incision for signs of infection.
28.
Remove wound clips one week after surgery with appropriate forcepsn.
2.1.3 Notes
(a)
Needle and syringe can be cleaned prior to autoclaving by filling with sterile 0.5 M sodium hydroxide. Let sodium hydroxide sit in filled needle and syringe for 10 min. Rinse at least 15–20 times by filling and ejecting sterile water.
(b)
Using the working stock of anesthesia prepared in step 3, dosing volume can be quickly calculated by multiplying the mouse weight (g) × 5 = μL anesthesia mix. Ensure mouse is fully anesthetized approximately for 10 min after anesthesia administration; pinch hind paw while mouse is lying flat on its stomach. If the mouse is not fully under anesthesia, it will react to this stimulus. If mouse is still responsive, administer an additional 10 μL of anesthetics mix. Wait for another 10 min; if still responsive, administer an additional 5 μL.
(c)
Scruff the mouse and place the front teeth into the tooth bar. If necessary, use the stick end of a sterile cotton swab to open the mouth to assist in placing teeth into the hole of the tooth bar. Gently tug on the tail of the mouse to ensure the mouth is hooked in. Position and tighten the ear bars. Gently tighten nose clamp and straighten the body of the mouse. The mouse should be secure so that gentle downward pressure on the head will not alter its relative position to the frame.
(d)
Apply pressure with sterile cotton swab if any bleeding occurs.
(e)
Ensure that the skull is flat and level. Adjustments can be made using the ear and jaw bars on the stereotaxic instrument.
(f)
Ensure that there are no air bubbles and that there is slightly more loaded than will be injected. Note fill level.
(g)
Make sure the stereotaxic arm is precisely at 90° to the rail on which it sits.
(h)
Coordinates of target structures can be determined using a stereotaxic atlas (Paxinos and Franklin, The Mouse Brain in Stereotaxic Coordinates). Adjustments may need to be made to account for the age or strain of mice used.
(i)
After drilling the burr hole, bring needle back to bregma and zero coordinates again. Confirm that the burr hole is in the appropriate coordinates and that the needle will be able to enter through the burr hole straight and easily.
(j)
Ensure x and y coordinates are also accurate for target structure.
(k)
Note level of virus in syringe after injection. Ensure that appropriate virus volume was delivered.
(l)
Alternatively, the incision can be closed with sutures.
(m)
Once the mouse has awoken from anesthesia and is active, place it in normal cage setup with gel pack and food.
(n)
Alternatively, remove sutures if used.
2.2 Injection of AAV Vectors into the Cerebral Lateral Ventricles of Neonatal Mice
Infusion of AAV vectors into the cerebral lateral ventricles (intracerebroventricular route) of neonatal mice is an outstanding approach to achieve widespread gene delivery throughout the brain [85]. The advantage of intracerebroventricular delivery in neonatal mice for LSDs is the diffuse nature of CNS gene transfer [66, 85, 86] and early intervention at an age that may precede the onset of disease symptoms. In addition, the degree of neuronal gene transfer achieved by ICV delivery is considerably higher than achieved by systemic administration of the new blood–brain barrier-crossing AAV vectors (AAV9, AAVrh10, and others) [67, 73] using total doses 10–100-fold lower. CSF infusion of AAV vectors in adult mice shows limited distribution in the CNS [87], but recent studies in pigs and monkeys have shown that intrathecal or cisterna magna infusion of AAV9 vectors may be an effective route to achieve widespread transduction in the CNS [78, 88]. The therapeutic effectiveness of neonatal ICV infusions has been demonstrated in a number of mouse models of LSDs, including MPS VII [85], Krabbe disease [89], GM1-gangliosidosis [90], and mucopolysaccharidosis type I (MPS I) [91]. This CNS delivery route, especially in the context of AAV-encoded enzymes for the treatment of LSD, is both potent and viable.
2.2.1 Material and Reagents
Sterile AAV vector to be injected
10 μL NanoFil syringe (World Precision Instruments)*
36 gauge beveled needle (NF36BV-2; World Precision Instruments)*
Kimwipes
70 % ethanol
0.5 M sodium hydroxide
Isoflurane
Fiber-optic illuminator (optional)
*Autoclave before use
2.2.2 Protocol
1.
Remove the mother from the cage with the pups, and put in a separate clean cage.
2.
Place two pieces of Kimwipes into the bottom of a 50 mL conical tube. Add 1 mL of isoflurane onto the Kimwipes, and then add another piece of tissue.
3.
Load the syringe with the virus to be injected. We recommend injecting 2 μL into each of the lateral ventriclesa.
4.
Place a neonate pup (0–1 day old) into the conical tube and lightly tighten the cap. Do not screw it on fully. Watch the pup for movement. Remove immediately once the pup has stopped moving.
5.
Gently, but securely, hold the pup between your thumb and index finger, and identify the lambda suture landmark. Insert the syringe tip 0.5 cm ventral, 1 cm lateral from the sagittal suture, and 2 cm anterior from the transverse suture. Slowly inject 2 μL.
6.
Repeat for the contralateral ventricleb.
7.
Place the pup on a warm surface, and wait for it to wake up from anesthesiac.
8.
Rub the pup with bedding from its home cage, and place it back into its cage.
9.
Once all the pups have been injected and returned to their home cage, return the mother as welld.
10.
Clean the syringe with 0.5 M sodium hydroxide, as noted above (step 3)e.
2.2.3 Notes
(a)
The syringe should be cleaned every time a new virus is used. Cleaning should be done by loading the syringe with 0.5 M sterile sodium hydroxide for ten minutes, followed by 15–20 flushes with sterile water.
(b)
If the sutures are hard to distinguish, place the animal on a dissecting illuminator, and illuminate the head from below. However, be certain that the illuminating surface is not too hot, as to not burn the animal. Preferably use a fiber-optic light to prevent this potential issue.
(c)
The pup should wake up within 5 min.
(d)
If problems arise with the mother’s rejection of the pups, rub down the animal first with a paper towel moistened with ethanol. Likewise, pat the nose of the mother with the same paper towel. If the pups have been marked in any sort of way (i.e., a sharpie, tattoo paste), also mark the mother (on the tail or fur). Note that it is common for the mother to pick up the pups and carry them to a different spot in the cage.
(e)
It is common for the needle to clog, so having a stock of needles is recommended.
3 Infusion of AAV Vector into the Brain of Large Animal Models of LSDs
3.1 Materials and Reagents
Drugs
Atipamezole (Antisedan, Zoetis)
Buprenorphine (Buprenex, Reckitt Benckiser)
Dexmedetomidine (Dexdomitor, Zoetis)
Florfenicol (Nuflor, Schering-Plough)
Flunixin meglumine (Banamine, Schering-Plough)
Isoflurane (Isothesia, Butler Animal Health Supply)
Ketamine (Ketaset, Fort Dodge)
Lactated Ringer’s Solution
Midazolam (Hospira Inc.)
Morphine (West-Ward)
Injection contrast reagent
Gadoteridol (Prohance, Bracco Diagnostics, 279.3 mg/mL)
Equipment
Intravenous catheter (Surflash, Terumo)
Rectal temperature probe (ReliOn)
Electrocardiogram (Cardiocap II, Datex-Ohmeda)
Capnograph (Capnomac Ultima, Datex-Ohmeda)
Pulse oximeter (Cardiocap II, Datex-Ohmeda)
Doppler peripheral pulse monitor (Parks Medical)
Sphygmomanometer (Parks Medical)
Veterinary patient warming system (Hot Dog, Augustine Biomedical Design)
Horsley–Clarke stereotaxic apparatus (David Kopf Instruments)
Hamilton syringe (Harvard Apparatus)
Non-coring needle (22–25 G) (Harvard Apparatus)
8–5 MHz ultrasound probe (Philips HDI 5000)
4–0 monofilament suture (Monocryl, Ethicon)
Oscillometric blood pressure monitor (Cardiocap II, Datex-Ohmeda)
0 monofilament suture (PDS II, Ethicon)
3.2 Stereotaxic Injection of AAV Vectors into the Brain of Feline Models of Gangliosidoses
Feline models of GM1 and GM2 gangliosidosis were first described in the 1970s [92, 93] and have been extremely well characterized in the intervening years [94, 95], presenting an unparalleled opportunity to test therapeutic options for translation to human clinical trials. The cat brain is only ~16 times smaller than the infant human brain, which it closely resembles in terms of complexity and organization. Also, an alternative catabolic pathway for GM2 ganglioside has been reported in mice [52] which is physiologically insignificant in humans and less active in cats [96], suggesting that cats may better model the human GM2 degradative process.
Feline models of both GM2 (SD) and GM1 gangliosidosis have similar clinical presentations, beginning with slight head and/or tail tremors that progress to severe whole-body tremors, ataxia, loss of balance, and inability to stand (humane endpoint). Neurologic disease onset in SD cats occurs at ~1.7 months of age, progressing to humane endpoint at 4.5 ± 0.5 months (mean ± standard deviation, n = 11). Due to a low level of residual β-galactosidase activity in GM1 cats, the disease phenotype is less severe, with onset at ~3.5 months and humane endpoint at 7.7 ± 0.8 months (mean ± standard deviation, n = 9).
We have shown that intracranial injection of recombinant AAV vectors in GM1 and GM2 cats has a dramatic therapeutic effect, considerably extending survival and improving motor function and quality of life [58, 97–99]. Initial therapeutic studies were conducted by bilateral injections of AAV vectors into the thalamus and deep cerebellar nuclei (DCN). However, implementing DCN injections in humans is fraught with considerable difficulties: (1) the majority of experience with human stereotactic procedures (injections, deep brain stimulation, biopsy) is supratentorial, so the optimal strategies and techniques for targeting cerebellar structures safely are not as well understood; (2) frameless techniques that rely on intraoperative registration are hindered by the relatively featureless occiput, so an implanted fiducial system may be necessary; (3) hemorrhage in the posterior fossa may harm critical brain stem structures, either directly or indirectly (through excessive pressure). In the relatively small volume of the posterior fossa, even minor hemorrhages can be devastating. Therefore, our team has investigated delivery routes that could replace DCN injections. We have shown that unilateral ICV delivery of AAV vectors is therapeutically equivalent to bilateral DCN injection, as long as either route is performed in conjunction with bilateral thalamic treatment. Intracerebral (thalamus, DCN, and ICV) injection procedures are described below. Surgeries have been performed on GM1 and GM2 gangliosidosis cats at 4–12.5 weeks of age and 0.4–1.4 kg body weighta.
Protocol:
Anesthesia and Monitoring
1.
Insert aseptically an intravenous catheter (22–24 G) into a cephalic veinb.
2.
Administer buprenorphine (0.01 mg/kg) intravenously as a premedication and for analgesia during the surgery.
3.
Anesthetize cats with ketamine (10 mg/kg) and dexmedetomidine (0.04 mg/kg) by injection through the intravenous catheter and maintain using isoflurane (0.5–1.5 %) in oxygen delivered through an endotracheal tube. Intravenous fluids are administered at a rate of 10 mL/kg/h.
4.
Monitor continuously physical parameters and anesthetic depth using a rectal temperature probe, electrocardiogram, capnograph (end tidal CO2), pulse oximeter (O2 saturation) and Doppler peripheral pulse monitor, and sphygmomanometer (blood pressure)c.
5.
Provide supplemental heat (Hot Dog veterinary patient warming system) to prevent hypothermia.
Craniotomy
6.
Place animal in a sternal position, and fix the head in a Horsley–Clarke stereotaxic apparatus.
7.
Clip the hair off of the dorsal cranium, and prepare it aseptically. Make a single longitudinal incision through the skin and periosteum to expose the skull.
8.
Create craniotomy entry sites through the skull and dura with a 20 G hypodermic needle (which is more than sufficient to penetrate the soft skull in the 4–12.5 weeks old cat).
Thalamic Injection
9.
Stereotaxic coordinates (in cm) for the thalamus relative to bregma are given in three planes, anterior–posterior (AP), mediolateral (ML), and dorsoventral (DV): AP −0.7, ML ±0.4, DV −1.6. The DV zero point is marked when the needle tip touches meningesd.
10.
Make a single craniotomy directly above each thalamic target.
11.
Use a sterile 100 μL Hamilton syringe fitted with a non-coring needle (22–25 G) to inject the AAV vector.
12.
Advance the needle through the skull and into the target structure (thalamus) manually at a rate of 1 mm/s.
13.
Inject a total of 70 μL in 10–20 μL boluses at a rate of 2 μL/min. Raise the needle by 0.15 cm between each injection bolus, so that the final needle position is 1.15 cm ventral to meninges (~2.5 mm ventral to the lateral ventricle).
14.
Leave the needle in the thalamus for 1 min after the final injection, and then raise it halfway. After another minute, withdraw the needle completely over 30 se.
DCN Injection
15.
Stereotaxic coordinates (in cm) for the deep cerebellar nuclei (DCN) relative to lambda are AP 0.0, ML ±0.4, DV −1.25. The DV zero point is marked when the needle tip touches meningesd.
16.
Make a craniotomy above each DCN using a 20 G hypodermic needle inserted vertically (i.e., at an angle perpendicular to the stereotaxic apparatus). Advance the needle through the skull and cerebellar tentoriumf.
17.
Use a sterile 100 μL Hamilton syringe fitted with a non-coring needle (22–25 G) to inject the AAV vector. Lower needle through the skull and into the target area at a rate of 1 mm/s, using the micromanipulator affixed to the stereotaxic unit.
18.
Inject a total of 24 μL in 10–14 μL boluses at a rate of 2 μL/min. Raise the needle by 0.15 cm between each bolus, so that the final needle position is 1.1 cm ventral to meninges.
19.
Leave the needle in the DCN for 1 min after final injection, and then raise it halfway. After another minute, withdraw the needle completely over 30 se.
ICV Injection
Due to the narrow lateral ventricle of cats (≤2 mm wide), ultrasound guidance is used to confirm correct placement of the injection needle. Since the thalamic injection needle transects the lateral ventricle on its descent, it may disrupt ventricular volume and architecture. Therefore, it is prudent to perform ICV injection prior to treatment of the thalamus.
20.
Make a 2 cm incision in the skin over the bregmatic fontanelle.
21.
Attach the ultrasound probe and biopsy guide to a micromanipulator on the stereotaxic unit. This requires a custom positioning device that we devised for this purpose.
22.
Visualize the left lateral ventricle using an 8–5 MHz ultrasound probe.
23.
Insert a 20 G, 2.5″ spinal needle through the biopsy guide to penetrate the skull and serve as an anchor and guide for the injection needle.
24.
Load AAV vector into a sterile 250 μL Hamilton syringe fitted with a 25 G, 3.5″ needle, and manually insert it through the guide needle into the lateral ventricle. The needle bevel should face dorsally for easier ultrasound confirmation of proper placement within the lateral ventricle.
25.
Inject a total of 200 μL of AAV vector (with or without 4 % gadoteridol in the injectateg) in 10–15 μL boluses at a rate of 3–5 μL/s, with ~1 min gap between boluses.
26.
Leave the injection needle in place for 2 min after the final injection and then withdraw completely. Finally, remove the guide needlee.
Postoperative Care
27.
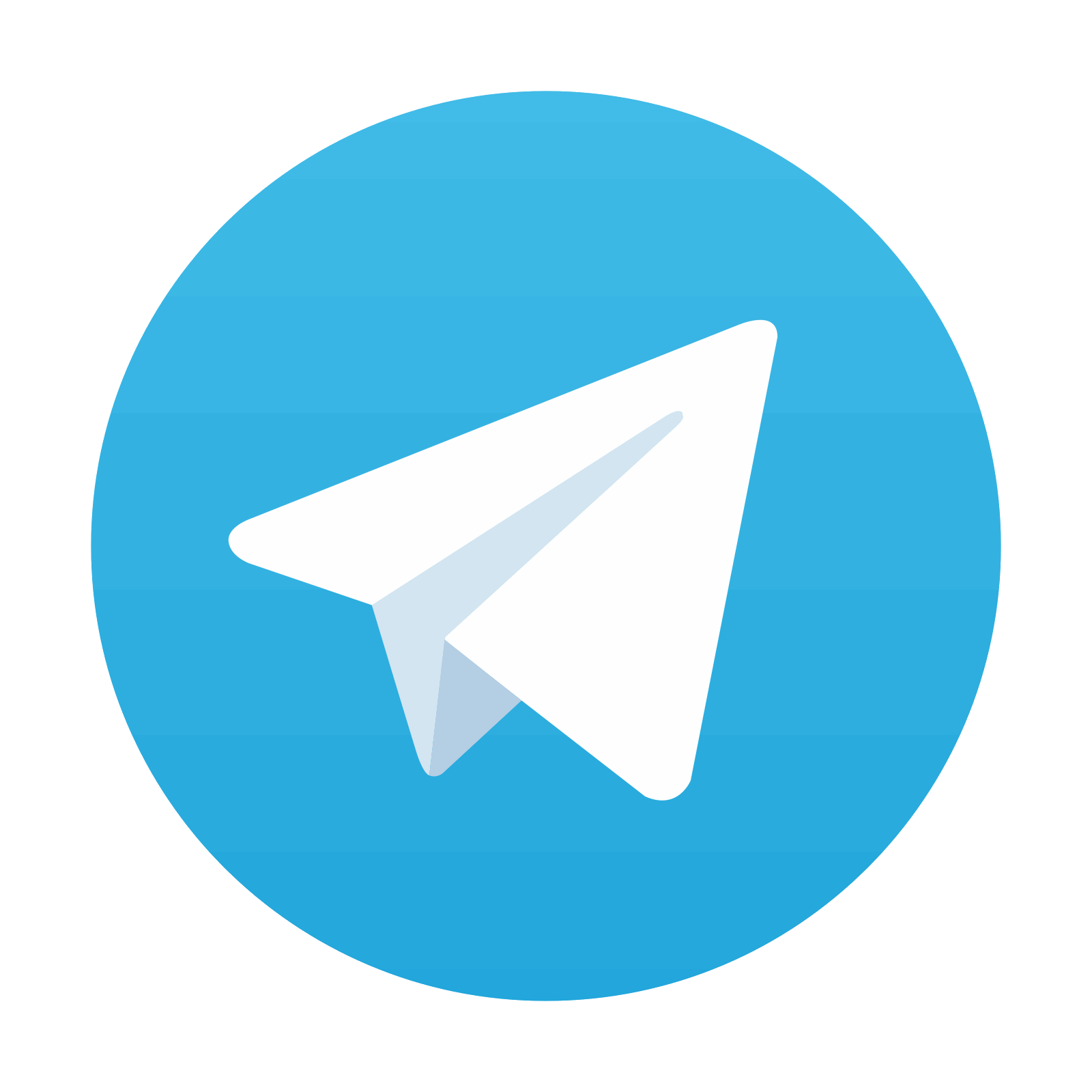
Close the skin incision with a single layer closure using a simple interrupted pattern with 4–0 polyethylene suture.
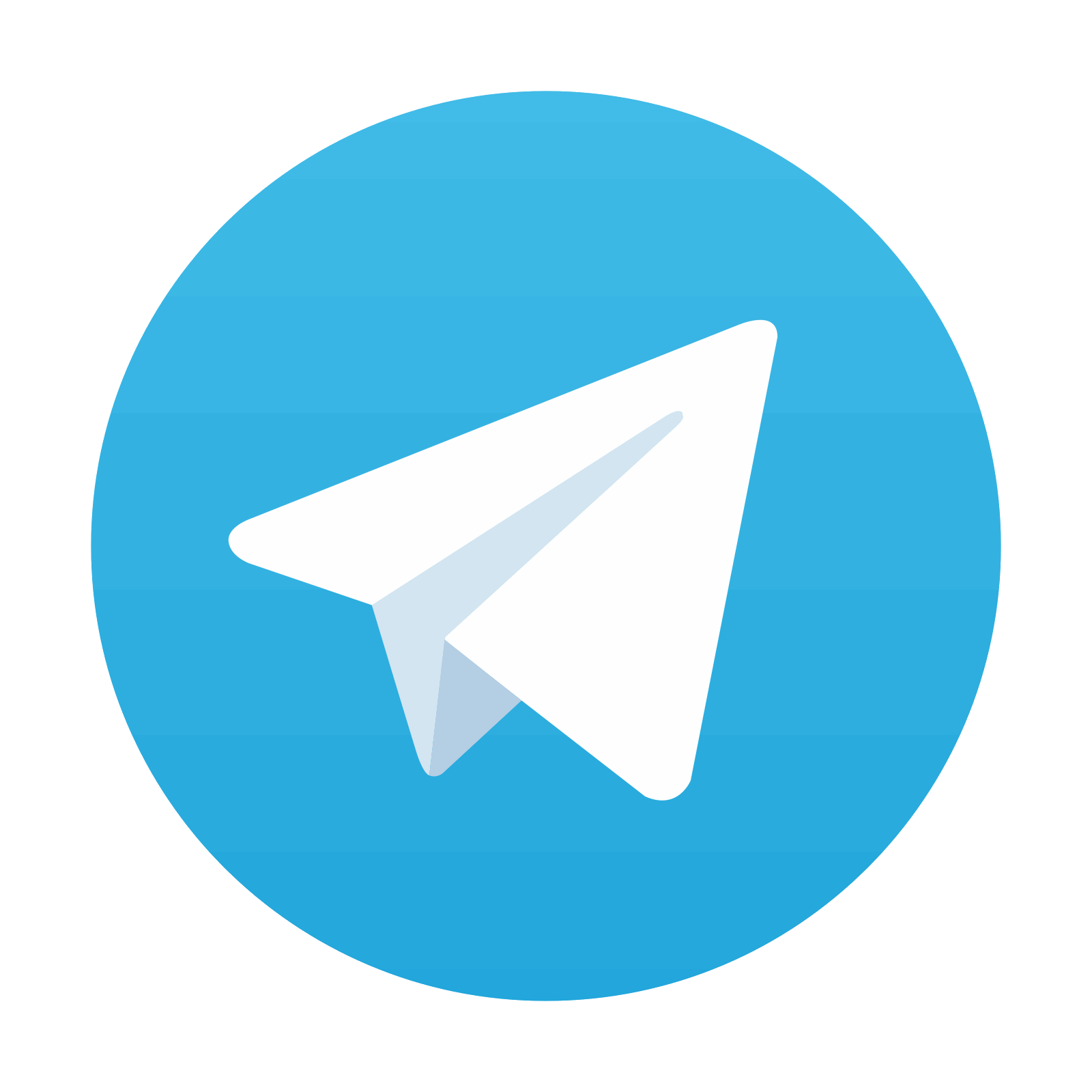
Stay updated, free articles. Join our Telegram channel

Full access? Get Clinical Tree
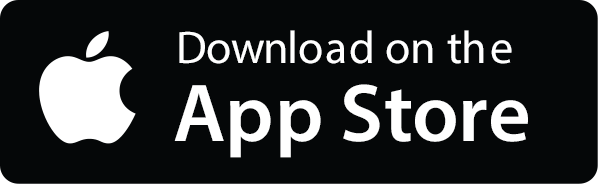
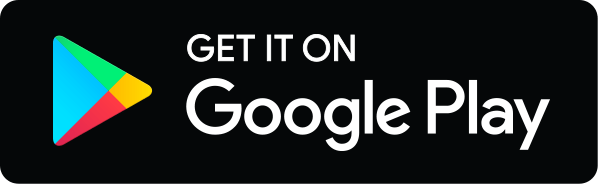
