First described over 200 years ago by Tissot, childhood absence epilepsy (CAE) is a common pediatric epilepsy syndrome that affects otherwise normal children, with onset between ages 4 and 10 years and with a peak onset between 6 and 7 years.1–5 Commonly misperceived as a “benign” epilepsy syndrome, patients with CAE demonstrate variable response to therapy, exhibit cognitive deficits, encounter elevated rates of accidental injury, demonstrate long-term psychosocial difficulties, and have variable remission rates. Similar to other epilepsies, the current therapeutic approach for children with CAE is empiric: an antiepileptic drug (AED) is selected based on available data and clinical experience, then titrated to an acceptable balance of seizure control and side effects.
Electroencephalography (EEG) is absolutely essential in the diagnosis and management of CAE, as well as other epilepsy types that include absence seizures. Video-electroencephalography monitoring (VEM) has several benefits over routine EEG in the diagnosis and management, including distinguishing absence seizures from nonepileptic events, identifying subtle clinical signs in seizures otherwise felt to be subclinical, and potentially identifying specific clinical signs during seizures that could affect outcome. This chapter will review CAE, with an emphasis on how EEG and VEM affect diagnosis, classification, study design, and treatment response.
Approximately 10 to 15% of all children with epilepsy have CAE.2,3 The annual incidence ranges from 6.3 to 8.0 per100,000 in children younger than 15 years.4,5 CAE is often considered a “benign” epilepsy syndrome, likely to be outgrown, and with no risk of injury or other complications. However, accidental injury is common in CAE, with 20% of young adults with CAE reporting an injury during an absence seizure.6 The risk of accidental injury resulting from an absence seizure has been estimated to be 3% per person year.7,8 Also, in contrast to the “benign” description, seizure remission is far from certain. The long-term remission rates for absence epilepsy range from 21 to 89%. This wide range likely results from inconsistent and variable study inclusion criteria, methods, follow-up length, and outcome definitions.9,10 Furthermore, children with CAE have comorbid cognitive, behavioral, and/or psychosocial problems. Compared with matched controls, the mean percentiles in patients with absence epilepsy are lower for general cognitive functioning (25th vs 55th percentile), visuospatial skills (32nd vs 62nd percentile), nonverbal memory (50th vs 71st percentile), and delayed recall (24th vs 65th percentile).6 In a population-based study in Nova Scotia, psychosocial functioning, including social relationships, school function, and overall behavior, was found to be impaired in one third of children with CAE. Legal conviction rates and alcohol abuse were also elevated in young adults with a history of CAE, with 13% of CAE patients having a legal conviction excluding a traffic offense, compared with the province-wide conviction rate 5.4% of men and 1.2% of women in Nova Scotia. A history of alcohol abuse was also present in 39% of young adults with CAE.11
Absence seizures are characterized by brief episodes of staring, behavioral arrest, and unresponsiveness and are often associated with eye fluttering, facial myoclonus, or extremity automatisms (Video 12-1). Changes in neck or truncal tone are less common, and it is unclear whether such changes in tone suggest an “atypical absence seizure” or dispute a diagnosis of CAE. Pending further study, we accept changes in neck or truncal tone during an absence seizure as within the broad array of clinical signs potentially consistent with typical absence seizures and with CAE.
The term absence seizure, in isolation, implies nothing about the neurologic status of the child and does not automatically imply CAE, an epilepsy syndrome. The terms typical absence seizure and atypical absence seizures are confusing and unclear, but they are part of the clinical vocabulary historically and used when discussing absence seizures. In our view, typical absence seizures should not be defined based on specific semiologic features during a seizure. Rather, typical absence seizures occur in otherwise normal children, are brief (usually 5–30 s), are often precipitated by hyperventilation, and display bilateral, symmetric, synchronous, approximately 3 Hz spike and slow-wave activity on EEG superimposed on a normal EEG background. The frequency of the epileptiform activity typically slows to 2.0 to 2.5 Hz during runs lasting at least several seconds. Brief seizures may occur hundreds of times per day, with each followed by abrupt return to baseline functioning (Video 12-2). Many of these seizures are so brief that they are unrecognized. Typical absence seizures are a key component of the diagnosis of the epilepsy syndrome CAE, but a diagnosis of CAE requires additional criteria, such as the particular age of onset.
Atypical absence seizures may occur in neurologically abnormal children, may evolve to tonic or atonic “drop attacks,” may be prolonged, may be accompanied by slow spike and slow-wave complexes (≤ 2.5 Hz) activity on EEG, and may be superimposed upon a slow or disorganized EEG background. When defined in this way, atypical absence seizures are not compatible with a diagnosis of CAE.
Childhood absence epilepsy (CAE) is an idiopathic generalized epilepsy (IGE) defined by the International League Against Epilepsy (ILAE) as “pyknolepsy that occurs in children of school age (peak manifestation age 6–7 years), with a strong genetic predisposition in otherwise normal children. It appears more frequently in girls than in boys.12 It is characterized by very frequent (several to many per day) absence seizures. During adolescence, generalized tonic-clonic seizures may develop. Otherwise, absences may remit or, more rarely, persist as the only seizure type.”
Despite the classic EEG presentation, there are a number of variations in EEG patterns seen in children with absence seizures. These patterns vary from “lead-in” patterns of epileptiform discharges arising from the frontal or occipital regions, which occur prior to the generalized 3 Hz spike and slow-wave activity. In addition, some generalized discharges appear to be poorly synchronized, and focal interictal discharges may be seen.13 The clinical significance of these findings is unclear, although one study suggested that a focal onset in ictal EEGs may confer a higher chance of seizure relapse. However, this finding was not statistically significant.13 In addition to background slowing and disorganization, atypical absence seizures may have EEG features, such as multifocal epileptiform discharges and generalized paroxysmal fast activity.14
The boundary between interictal abnormalities and ictal discharges in CAE is not always clear in clinical practice. Recent studies have defined an absence seizure as a generalized spike and wave discharge (SWD) lasting at least 3 seconds.15 The “3 seconds” rule is clinically reasonable, as >90% of generalized SWDs lasting at least 3 seconds are accompanied by some clinical manifestation.15 The onset of the discharge corresponds with the acute onset of altered mental status in the child. The child’s behavior normalizes as quickly as the discharge ends. Although 3 seconds is considered the typical minimum duration of epileptiform activity to evoke a clinical change, shorter bursts may be accompanied by clinical signs in some patients. VEM can aid in identifying such altered behavior and correlating it with EEG findings.
In clinical practice, EEG is essential in the evaluation of absence seizure frequency and AED efficacy. Seizure burden and treatment response can be assessed in various ways, including (1) parental report of clinically obvious seizures; (2) office-based hyperventilation test; (3) routine 20-minute EEG with hyperventilation trials; (4) 1-hour awake EEG (with or without video monitoring), including trials of hyperventilation; and (5) prolonged (6–24 hr) EEG, with or without video. Parental reports of clinically obvious seizures tend to underestimate the seizure burden because absence seizures, especially brief ones, are notoriously difficult to detect. A study comparing parental report of seizures with measures on EEG found that parental reports frequently underestimated the seizure burden by 5- or 10-fold.16 Office-based hyperventilation tests have been used to assess response to therapy in CAE, but false-positive and false-negative test results occur. False positives have been attributed to transient decreases in verbal recall and motor responsiveness in normal children subjected to hyperventilation, nonepileptic spells (e.g., fatigue and inattention during hyperventilation) clinically indistinguishable from absence seizures but unaccompanied by epileptiform activity on EEG, and complex partial seizures mistaken for absence seizures.17–19 False negatives also occur because only two thirds of patients with documented CAE have clinical seizures provoked with hyperventilation.20 A routine 20-minute EEG with hyperventilation testing (without video) is often used in clinical practice as a means of assessing therapy in CAE and appears to accurately define seizure control in 90% of patients.16 In a study of 24-hour ambulatory EEG in CAE, the first hour of awake recording was highly correlated with total seizure frequency during wakefulness.21 The generalized SWDs usually persist during sleep but become briefer, more fragmentary, and more irregular in morphology.22 Patients with CAE do not display epileptic encephalopathy, developmental regression, or excessive daytime sleepiness, suggesting that nocturnal EEG abnormalities may have no impact on daytime performance. In clinical practice, sleep recordings will not add significant clinically relevant information. An EEG lasting between 20 and 60 minutes, with two trials of hyperventilation, appears to be a reliable tool to accurately diagnose CAE and assess treatment efficacy.
The goals of treatment of absence seizures include the elimination of clinically obvious seizures and normalization or near-normalization of generalized spike and SWDs on EEG without clinically significant medication adverse effects.
Older studies regarding treatment of absence seizures included some neurologically abnormal patients with atypical absence seizures, which limited the accuracy of the results toward outcomes in CAE. Newer treatment studies have excluded patients with other neurologic abnormalities, but little attention has been paid to specific semiologic or EEG features in predicting treatment outcome.
Until the 1940s, CAE was essentially not treatable, as available AEDs such as phenobarbital and phenytoin are not effective in the treatment of CAE. Tridione was an early effective treatment for CAE, as was reported in a case series by Lennox in 1945.23 In this study, the complexities of epilepsy classification were obvious. Some patients had only petit mal seizures, but others had atypical absence, myoclonic, and akinetic seizures. Of 40 patients, 28 were determined to have only petit mal seizures. After treatment with tridione, 28% of these 40 patients were seizure-free, and another 52% experienced 75% reduction. Adverse events associated with tridione included sedation, skin rash, and sensitivity of the eyes to sunlight.23
Based on short-term clinical trials, three AEDs—ethosuximide, lamotrigine, and valproic acid—are efficacious against absence seizures. However, their long-term efficacy is unknown, their toxicity profiles differ from one another, and their long-term effects on the developing nervous system of children have not been well studied. Ethosuximide, released in 1957, was a major advance in the treatment of CAE, as was valproic acid, released in 1978. Although the actual data supporting their use for CAE are not as strong as many clinicians believe, both ethosuximide and valproic acid are acceptable first-line choices in the treatment of absence epilepsy.24 Lamotrigine, released in 1994, is another acceptable first-line choice. The choice of ethosuximide, valproic acid, and lamotrigine is dependent on the side effect profiles, dosing formulations, and titration schedules.
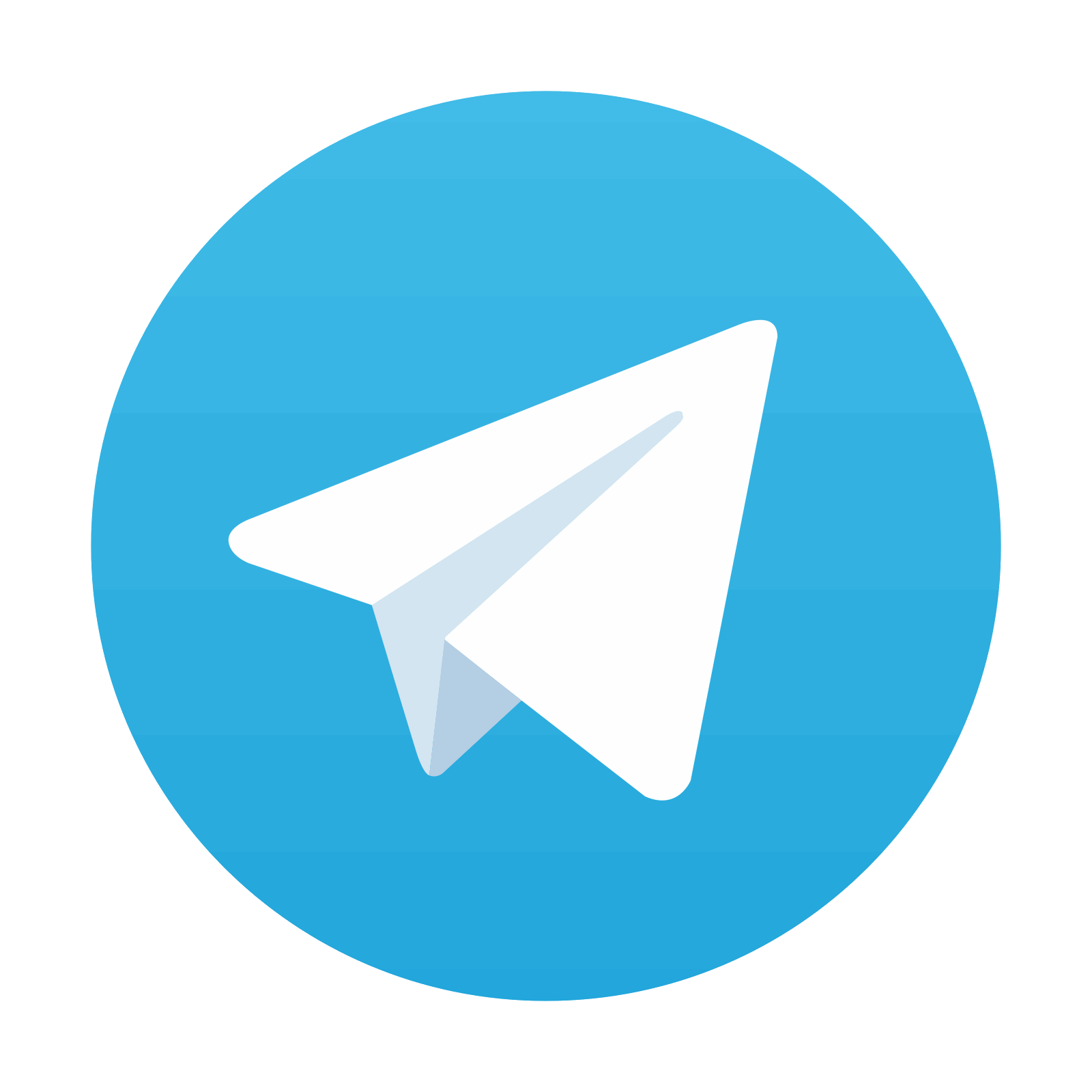
Stay updated, free articles. Join our Telegram channel

Full access? Get Clinical Tree
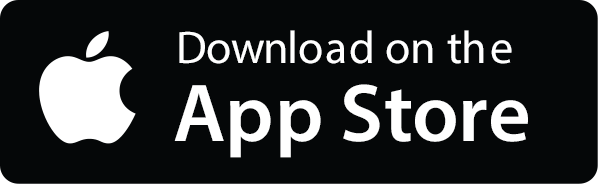
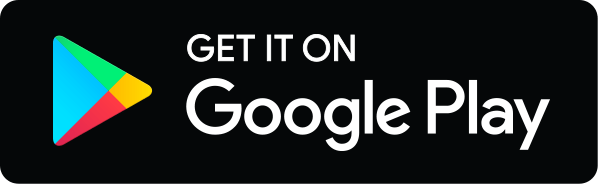