A 53-year-old hypertensive woman (actual body weight, 70 kg; height, 160 cm) was admitted to the hospital with coma (Glasgow Coma Scale E1+M3+V1t) after acute onset ictal headache. Computed tomography (CT) of the head showed subarachnoid hemorrhage with severe intraventricular extension and right temporal intraparenchymal hematoma (Figure 43-1). Cerebral angiography demonstrated 2 aneurysms of the carotid siphon, at the origin of posterior communicating arteries, bilaterally, with likely bleeding from the right one (9 mm diameter, neck 3.5 mm). An external ventricular drain was placed, the blood pressure was controlled with nicardipine drip, and the patient underwent endovascular coiling of the right carotid artery aneurysm.
On ICU admission, after coiling, her pupils were equal and reactive to light. Sedation was maintained with infusions of propofol, 30 to 60 µg/kg/min, and remifentanyl, 0.5 to 1 μg/kg/min. The patient, whose predicted body weight (PBW) was 52.2 kg, was ventilated by pressure-limited, volume-cycled ventilation with a tidal volume of ≈8 mL/kg per PBW, respiratory rate of 20 breaths per min, positive end-expiratory pressure (PEEP) of 8 cm H2O and Fio2 of 0.4. Arterial gas analysis revealed pH 7.42, Po2 164 mm Hg, Pco2 41 mm Hg, HCO3− 27 mmol/L, base excess 3.2 mmol/L, lactate 1.3 mmol/L, Po2/Fio2 ratio (P/F) 410. Her hemodynamic profile was characterized by hypertension, which was tolerated following the aneurysm coiling procedure. Intracranial pressure (ICP) remained within normal values, neurologic examination revealed GCS E3+M4+V1T. Enteral nimodipine, 60 mg q4h, was administered Fio2
On day 6, the clinical course was complicated by fever, presence of abundant pulmonary secretions and a decrease of the P/F ratio to 170. Chest radiograph showed a right basilar infiltrate (Figure 43-2). Empiric antibiotic therapy for nosocomial, ventilator-associated pneumonia was started with linezolid, 600 mg IV q12h, and piperacillin-tazobactam, 4.5 g IV q8h.
On day 8, CT angiography of the head revealed moderate vasospasm in the territory of right anterior and middle cerebral arteries, confirmed by cerebral angiography and treated with intraarterial nicardipine. On day 10 tracheostomy was performed. On day 11, she developed severe hypoxemia and met criteria for ARDS according to the Berlin Definition,1 (the P/F ratio dropped to 140 and then to 80), pH 7.45, Pco2 35 mm Hg, Po2 56 mm Hg, HCO3− 24.8 mmol/L, base excess 0.6 mmol/L, lactate 1.4 mmol/L; chest radiograph revealed diffuse and bilateral opacities involving 3 quadrants (Figure 43-3). Transthoracic echocardiography demonstrated normal systolic and diastolic ventricular function without valvular abnormalities, excluding a cardiogenic origin of pulmonary edema. She developed septic physiology with body temperature of 38.9°C (102.02°F) that was difficult to control both with physical methods and antipyretic drugs, and the white blood count increased to 20,000 cells/μL. Mechanical ventilation settings were increased to positive end-expiratory pressure (PEEP) 16 cm H2O and Fio2 0.8, and the tidal volumes were decreased to 6 cc/kg IBW.
Because of concern for the effects of hypercarbia on cerebral edema, end-tidal carbon dioxide (ETCO2) monitoring was initiated, and the ETCO2 maintained at 30-45 with pH > 7.25. On day 12 oxygenation worsened, and the decision was made to initiate prone positioning along with inhaled nitric oxide gas therapy set at 40 parts per million. Using great caution to prevent accidental dislodgment of the EVD, and monitoring ICP throughout the process, she was placed in the prone position, with an immediate improvement in both oxygenation and ventilation. The proning procedure was performed at 8-hour intervals for 3 days and aggressive diuresis was initiated using a pulse-contour cardiac output device to verify that cardiac output was maintained during diuresis. Oxygenation steadily improved, and following tracheostomy she was weaned completely off mechanical ventilation on day 22.
Acute respiratory distress syndrome (ARDS) is characterized by an inflammatory response of the lung following direct or indirect insults. The clinical syndrome is characterized by severe hypoxemia, reduced pulmonary compliance and diffuse radiographic infiltrates.2 ARDS occurs in 4% to 38% of patients with isolated brain injury and is an independent predictor of poor outcome.3 Understanding of the pathophysiological mechanisms of this syndrome may lead to the development of therapeutic strategies that could improve outcomes for patients with acute brain injury. This chapter focuses on current knowledge regarding (1) the incidence of ARDS in patients with acute neurologic status, (2) pathophysiological mechanisms of ARDS in patients with severe brain injury, (3) the role of mechanical ventilation in the prevention and treatment of ARDS, and (4) other therapeutic strategies available for this selected group of patients.
ARDS is a clinical syndrome of acute diffuse lung injury characterized by inflammation leading to increased pulmonary vascular permeability and loss of aerated lung tissue. Hallmarks are severe hypoxemia and bilateral radiographic opacities on standard chest radiograph or CT scan. Physiological derangements include increased pulmonary venous admixture, increased physiological dead space, and decreased respiratory system compliance. Patients with ARDS are at increased risk of developing ventilator-induced lung injury, which can be reduced by the provision of lung protective ventilation. As such, the early identification of ARDS is important.
The 1994 American-European Consensus Conference Committee defined ARDS as a syndrome of acute onset, with bilateral infiltrates on chest radiography consistent with pulmonary edema, pulmonary-artery wedge pressure < 18 mm Hg or clinical absence of left atrial hypertension, and hypoxemia with a ratio of partial pressure of arterial oxygen to fraction of inspired oxygen (Pao2/Fio2) < 200.2 Patients meeting the above criteria, but with Pao2/Fio2 ratios < 300 were diagnosed as having acute lung injury. More recently, the Berlin Definition1 clarified the exclusion of hydrostatic edema, added basic informations about ventilator settings, and provided a slight improvement in predictive validity (Table 43-1).
Criteria for ARDS by the Berlin Definition | |
---|---|
1. | Acute: Onset during ≤1 week |
3. | Bilateral opacities consistent with pulmonary edema present on CT or chest radiograph |
3. | P/F ratio < 300 mm Hg with a minimum of 5 cm H2O PEEP (or CPAP) |
4. | Infiltrates are not fully explained by cardiac failure or fluid overload. An “objective assessment” should be performed in most cases if there is no clear cause such as trauma or sepsis. |
The incidence of ARDS in patients with severe brain injury has been reported at 5% to 20%.3-13 This variability may be explained by the specific types of patients included in the studies and by different definitions of ARDS. Most of the studies included patients with traumatic brain injury (TBI) but with different severities of injury as suggested by the Glasgow Coma Scale (GCS)14 on admission. Restricting the analysis to patients with severe TBI (GCS < 9) gives an incidence of 5% to 10%.4 In patients with subarachnoid hemorrhage (SAH),6,7,11,12 a higher incidence of ARDS has been observed, suggesting that although the cause of the primary brain injury was different, similar injurious stimuli were present to trigger the development of ARDS.
ARDS is reported to be an independent predictor of increased mortality and poor neurologic outcome in patients with TBI, SAH, and other brain injuries (Table 43-2). It is associated with prolonged intensive care unit (ICU) and hospital length of stay and a decreased number of ventilator-free days.8,12 Conversely, TBI is independently predictive of poor outcome among patients with ARDS.15
Severely and globally altered initial brain CT scan5 and low GCS3-5 are predictors of ARDS after TBI, as are extracranial factors such as administration of vasoactive drugs and a history of drug abuse.5 The development of ARDS in patients with acute brain injury has a bimodal distribution with an early peak on day 2 or 3 after the initiation of mechanical ventilation and a late peak on day 7 or 8,9 the latter often being related to concurrent pneumonia.16 Ventilator settings (tidal volume and respiratory rate) and a lower Pao2/Fio2 ratio are independent predictors of early ARDS (within 72 hours after mechanical ventilation), among major underlying ARDS risk factors (aspiration, pneumonia, and lung contusion) and treatment variables.8 These findings support the hypothesis that therapeutic strategies, as well as the severity of injury, may contribute to the development of ARDS.
Rogers and colleagues17 found a significant increase in the weight of the lungs but not of other organs in 50% of patients who died within 96 hours after an isolated head injury. Histologic examination showed edema, congestion, and hemorrhage, which, associated with the increased lung weight, supported a diagnosis of neurogenic pulmonary edema (NPE), characterized as an increase in extravascular lung water in patients who have sustained an acute neurologic injury.18 In patients who survived more than 96 hours, high initial intracranial pressure (ICP) and low cerebral perfusion pressure (CPP) were associated with severe deterioration of the Pao2/Fio2 ratio, even in the presence of an initially normal chest radiograph.
The “blast injury” theory”19 proposes that the sympathetic storm caused by an acute increase in ICP induces a transient increase in intravascular pressure, damaging the endothelium and enabling protein-rich plasma to escape into the interstitial and alveolar spaces. This theory postulates the coexistence of hydrostatic and high-permeability mechanisms of edema. Smith and Matthay20 suggested that hydrostatic edema was the principal underlying mechanism for NPE; however, in an experimental model of intracranial hypertension, McClellan et al21 concluded that extravascular protein accumulation resulted from direct adrenergic stimulation triggered by brain injury. The degree of capillary hypertension determines whether unbalanced Starling forces increase water flux across the endothelium or whether structural damage of the capillary wall allows plasma to escape into the interstitium and alveolar spaces.
Increased intracranial production of pro-inflammatory cytokines after acute brain injury results in secondary damage22,23 and release of pro-inflammatory mediators into the systemic circulation.24 McKeating reported an increased transcranial gradient of interleukin (IL)-6 within 48 hours after brain injury, suggesting intracranial production, probably involving glial cells.24 More recently, Hutchinson et al25 noted that in TBI patients with a favorable outcome, microdialysis concentrations of IL-1 receptor antagonist (IL-1ra, an anti-inflammatory cytokine) were significantly higher than those of IL-1β (a pro-inflammatory molecule). It is also known that pro-inflammatory cytokines, such as IL-1, IL-6, and tumor necrosis factor (TNF)-α, can modulate the expression of adhesion molecule activity, favoring migration of inflammatory cells out of the vasculature and into the lung.26 A strong association between systemic concentrations of soluble intercellular adhesion molecule-1 (ICAM-1) and poor neurological outcome has been demonstrated in patients with TBI.27
Based on this evidence, a “double hit” model has been proposed to explain the development of organ failure associated with acute brain injury. First, a TBI activates systemic inflammation.28 Once primed, the system is then vulnerable to later, normally innocuous secondary inflammatory insults, such as infections, mechanical stress induced by mechanical ventilation, and surgical procedures. These insults, which occur within hours or days after the primary injury, can lead to further damage of the central nervous system and may contribute to the failure of several organs distant from the brain, leading to the development of the multiple organ dysfunction syndrome. Recent evidence suggests the respiratory system is among the organs most susceptible to such insults.29
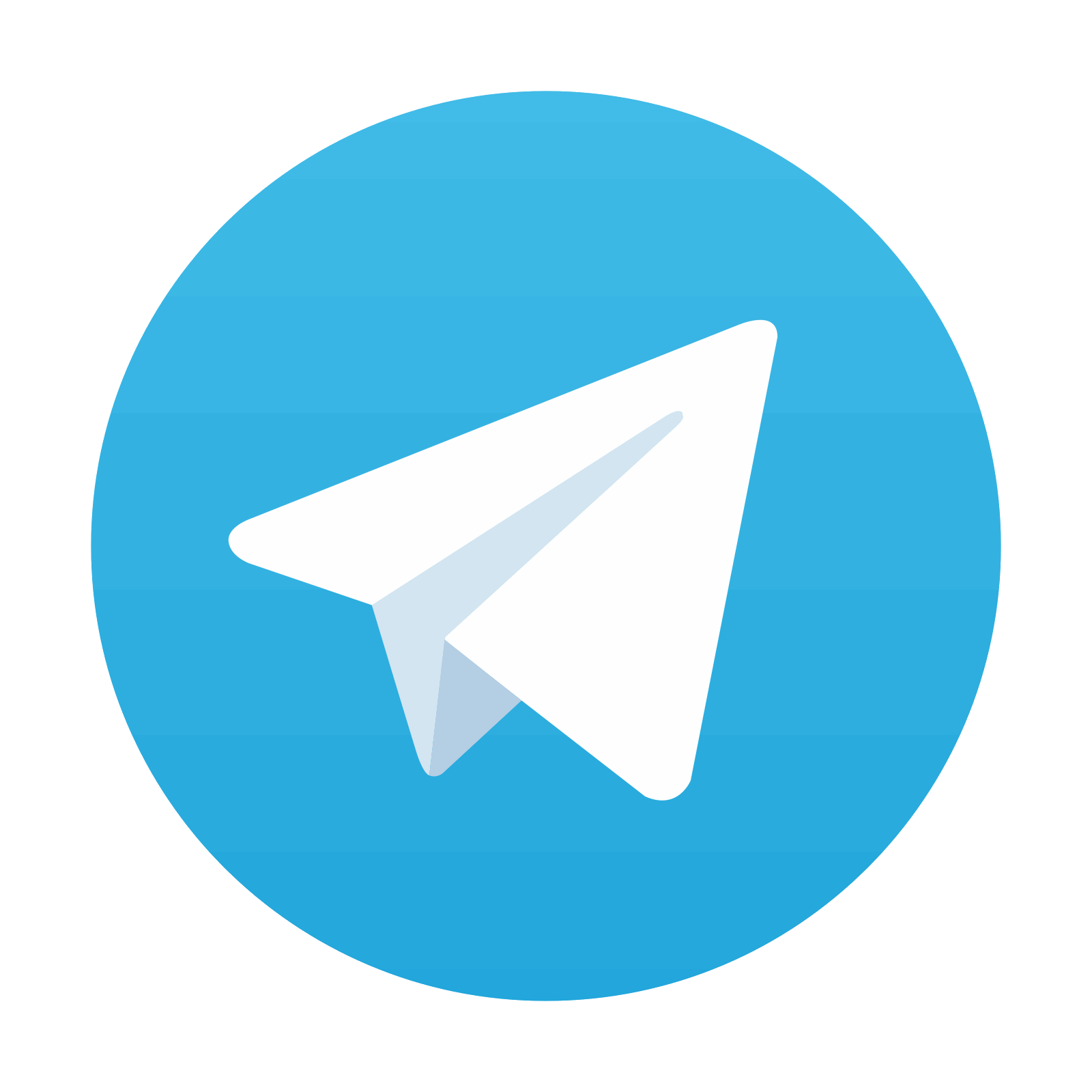
Stay updated, free articles. Join our Telegram channel

Full access? Get Clinical Tree
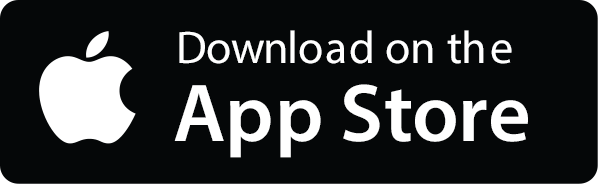
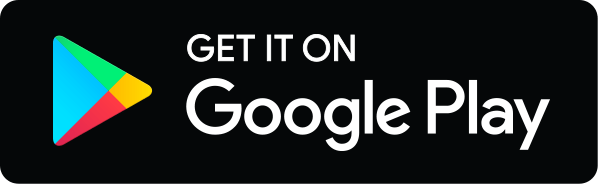