Fig. 1
Orexin neurons expresses adenosine A1 receptors as evident by double labelled fluorescence. Panel a Orexin-A containing neurons in the perifornical region (green fluorescence). Panel b Same visual field and section as Panel a showing adenosine A1 receptors (red fluorescence). Panel c Double labeled orexin-A and the A1 receptor (green arrow, indicative of A1 receptors on orexin neurons) along with single labeled orexin neurons (purple arrow) and A1 receptor-labeled neuron are also observed in the same visual field. Calibration bar = 25 µm. (Reproduced from Thakkar et al. 2002) (color figure online)
7 A1 Receptors Expressed on Orexin Neurons Promote Sleep
The next question we asked was: Does A1 receptor expressed on orexin neuron have a physiological role in sleep regulation. We performed two experiment to address this question.
In the first set of experiments, male adult SD rats were bilaterally infused with a selective AD A1R antagonist 1, 3-Dipropyl-8-phenylxanthine (DPX) into the orexinergic hypothalamus at sleep (light) onset and its effects on spontaneous bouts of sleep–wakefulness was monitored (Daly et al. 1985).
Since AD is implicated in the homeostatic regulation of sleep and recovery sleep following sleep deprivation is an essential paradigm to study the homeostatic mechanisms regulating sleep–wakefulness, our second set of experiments monitored the effects of A1 receptor antagonist on recovery sleep following sleep deprivation. Rats were sleep deprived by gentle handling during the last 6 h of the light cycle (Thakkar et al. 2003). Bilateral microinjections of either saline (0.9 %) or 15 pmol of DPX into the orexin-rich lateral hypothalamus was performed 30 min before the animals were allowed to enter recovery sleep and recovery sleep was monitored for 3 h.
The results obtained are as follow: Bilateral microinjections of the selective A1 receptor antagonist, DPX into the orexin-rich perifornical hypothalamus, dose dependently, increased the amount of time spent in wakefulness and decreased the amount of time spent in sleep (NREM and REM phases) during 3 h of spontaneous bouts of sleep–wakefulness during the normal sleep (light) period (Fig. 2).
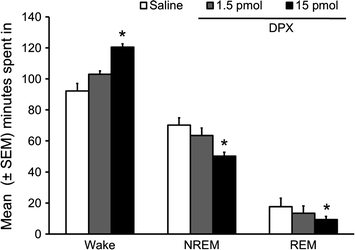
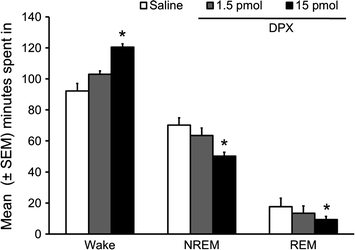
Fig. 2
Bilateral administration of DPX, adenosine A1 receptor antagonist, in the orexin-rich perifornical hypothalamus significantly and dose-dependently increased wakefulness with a concomitant reduction in NREM and REM sleep during first three hours of light period. *p < 0.05. (Reproduced from Thakkar et al. 2008)
Similarly, bilateral microinjections of DPX into the orexin-rich perifornical hypothalamus significantly increased the latency to the NREM phase of recovery sleep while significantly reducing both NREM and REM phases of sleep. Wakefulness was increased (Fig. 3). Thus, the results of our study confirm our hypothesis and suggest that A1 receptors on orexin neurons may have a physiological role in sleep-wakefulness.
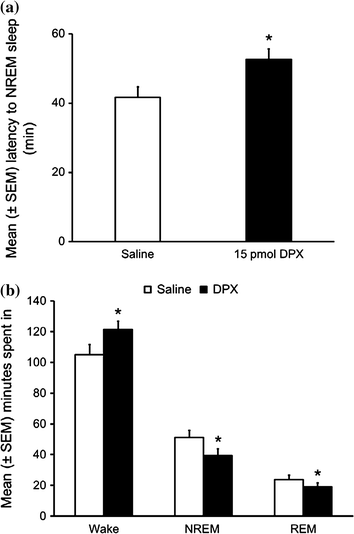
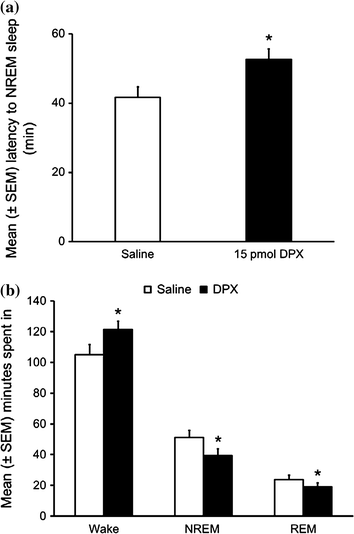
Fig. 3
Bilateral local microinjections of A1 receptor antagonist, DPX, into the orexin-rich perifornical hypothalamus significantly attenuated the recovery sleep following 6 h of sleep deprivation as evident by increased NREM sleep latency (a) and reduced total time spent in NREM sleep during first three hours of recovery sleep with a concomitant increase in the wakefulness (b). *p < 0.05. (Reproduced from Thakkar et al. 2008)
Thus, these results clearly demonstrate that AD via A1 receptors expressed on orexin neurons may play a significant physiological role in regulating sleep. Indeed, our results were confirmed by several subsequent studies. For example, in vitro electrophysiological studies have confirmed the role of AD via A1 receptor in the inhibition of orexin neurons (Liu and Gao 2007). In vivo studies in rats suggested that activation of A1 receptor in the orexin-rich perifornical hypothalamus suppressed the discharge activity of wake-active neurons and reduced the number of orexinergic neurons exhibiting c-Fos. Behaviorally, activation of A1 receptor in the orexin-rich perifornical hypothalamus increased sleep and reduced wakefulness. In contrast, blockade of A1 receptor resulted in increased wakefulness coupled with reduction in sleep (NREM and REM phase) along with an increase in the numbers of orexinergic neurons exhibiting c-Fos (Alam et al. 2009; Rai et al. 2010).
Now that we have established that AD via A1 receptor interacts with orexinergic system. Our next series of experiments were designed to examine the role of AD and orexin in alcohol’s effects on sleep.
7.1 Alcohol Is a Potent Somnogen
Our first experiment verified the effects of acute alcohol administration on spontaneous bouts of sleep-wakefulness in freely behaving SD rats. The experimental design was simple. Rats were instrumented for electrographic recording to examine and quantify sleep-wake states. To examine the potency of alcohol in sleep promotion, we administered alcohol (3 g/kg; intragastric) at the onset of circadian active period when rats are maximally active and all wake-promoting systems are very active. Our results suggest that alcohol administration at dark onset significantly reduced sleep onset latency (Fig. 4a) and increased NREM sleep (Fig. 4b). While wakefulness was reduced, REM sleep remained unaffected. Maximal sleep promoting effects of alcohol were observed during the first six hours after alcohol administration. These results suggest that alcohol is a potent somnogen and is able to counteract a strong wake-promoting drive, initiated by combined activation of all wake-promoting centers, to promote NREM sleep (Thakkar et al. 2010).
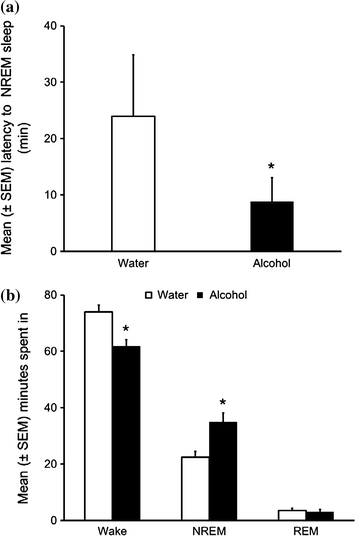
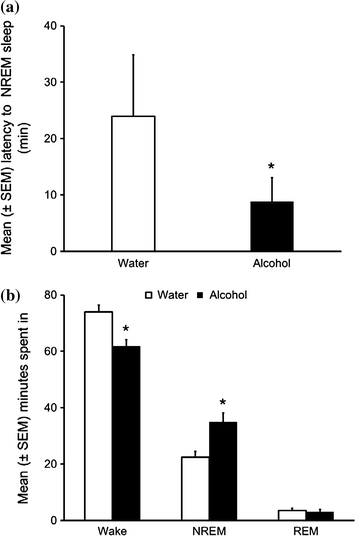
Fig. 4
Systemic alcohol administration (3 g/kg) at dark onset promote sleep as evident by reduction in NREM sleep latency (a) and increase in time spent in NREM sleep during 12 h of the dark period with a concomitant reduction in wakefulness whereas REM sleep remained unaffected (b). *p < 0.05. (Reproduced from Thakkar et al. 2010)
7.2 Alcohol Inhibits Orexin Neurons
Since the orexinergic system is among the most potent wake-promoting systems, we asked: Does alcohol mediates its sleep promoting effects by inhibiting orexin neurons? Our next experiment was designed to address this. Adult male SD rats were used as our animal model. Expression of c-Fos in the nucleus of orexin neurons was used as a marker of orexinergic activation. Double-label, orexin and c-Fos, immunohistochemistry (IH) revealed that alcohol (3 g/kg; intragastric) administration at dark onset significantly reduced the activation of orexin neurons as evident by a significant reduction in orexin neurons with c-Fos immunoreactivity (Fig. 5). Thus, based on these results we suggest that alcohol inhibits orexin neurons to promote sleep. The next question we asked was: What mediates alcohol’s inhibition of orexin neurons?
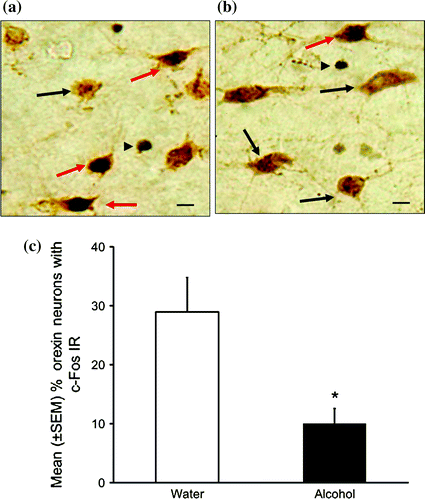
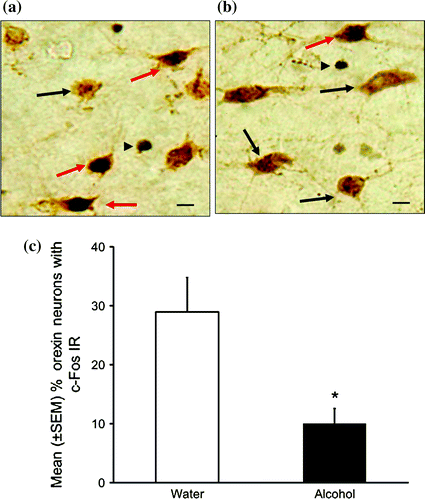
Fig. 5
A representative photomicrograph describing orexin neurons with c-Fos immunoreactivity (IR) in controls (a) and alcohol group (b). Red arrow double labeled orexin and c-Fos +ve neuons; black arrow single labeled orexin neurons; black arrowhead single labeled c-Fos +ve cells. Calibration bar = 10 µm. The percentage of orexin neurons with c-Fos IR was significantly reduced after acute alcohol administration (c). *p < 0.05 versus water (color figure online)
8 Alcohol Induced Inhibition of Orexin Neurons Is Mediated by Adenosinergic A1 Receptor
Since A1 receptors, expressed on orexin neurons, regulate sleep-wakefulness (see Figs. 1, 2 and 3), we hypothesized that alcohol may act via AD to activate A1 receptor resulting in the inhibition of orexin neurons and sleep promotion. Thus, we examined the effects of bilateral infusion of A1 receptor antagonist DPX on alcohol induced sleep. We performed the infusion of DPX at dark onset because the animals are maximally awake and spent >80 % time in wakefulness. DPX infusion at dark onset will have minimal effects on spontaneous wake-promotion due to “ceiling” effects (Thakkar et al. 2014). However, DPX will suppress alcohol induced sleep and promote wakefulness. The results of our studies are described in Fig. 6. Bilateral infusion of DPX into orexin-rich perifornical hypothalamus resulted in an attenuation of alcohol induced sleep promotion implicating an interaction of orexin, AD and its A1R in sleep promoting effects of alcohol.
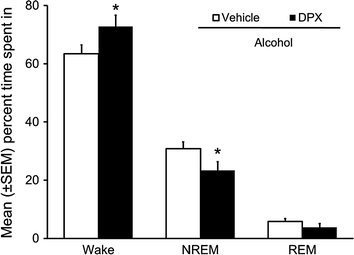
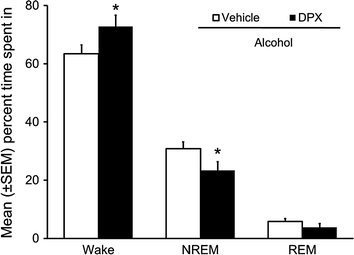
Fig. 6
Bilateral infusion of A1R antagonist, DPX, into the orexin-rich perifornical hypothalamus attenuated alcohol-induced NREM sleep promotion and promoted wakefulness during 12 h of dark period post-alcohol. Rapid eye movement (REM) sleep remained unchanged. *p < 0.05 versus vehicle
8.1 Alcohol Increases Extracellular AD in the Orexin-Rich Perifornical Hypothalamus
The “litmus test” to demonstrate the interaction of AD and orexin in mediating sleep promoting effects of alcohol will be to demonstrate local increase in extracellular AD following alcohol administration. Thus, in our next set of experiments, the effect of alcohol on extracellular release of AD in the orexin-rich perifornical hypothalamus was examined. Microdialysis was used to measure AD release. The specificity of alcohol’s action on orexin neurons was provided by reverse microdialysis, through the same microdialysis probe, delivery of pharmacological relevant alcohol doses into orexin-rich perifornical region.
As predicted, our results suggest that alcohol produced a dose-dependent increase in extracellular AD in the orexin-rich perifornical hypothalamus with maximal increase observed with 300 mM dose of alcohol perfused (Fig. 7). Reverse microdialysis perfusion of 300 mM dose of alcohol provides a concentration of ~25 mM directly outside the probe. This concentration of alcohol is in the range of alcohol concentration observed in the brain after a systemic administration of sedative dose (~3 g/kg) of alcohol (Sharma et al. 2014c). This study provides confirmatory evidence support the role AD and orexins in sleep promoting effects of alcohol.
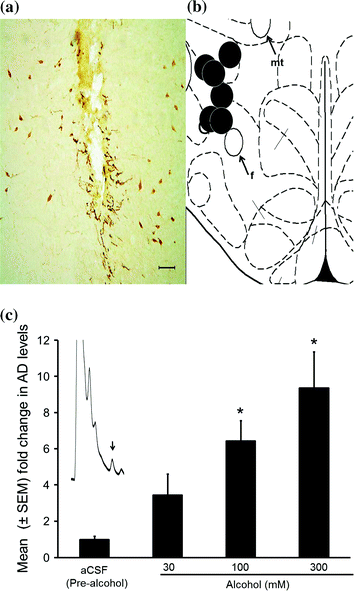
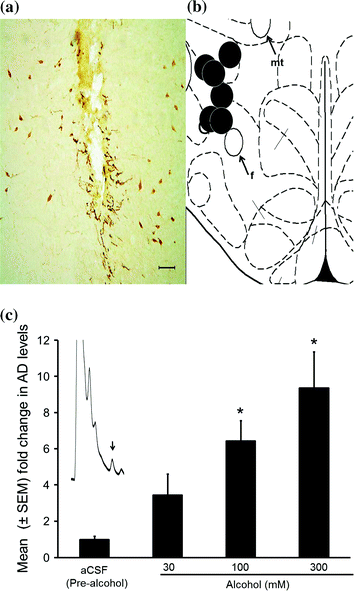
Fig. 7
Representative photomicrograph depicting lesions caused by microdialysis guide cannula (black arrow) in the midst of orexin-A containing neurons (a). All probe sites (black circles) were located between AP −3.2 to −3.6 mm from the bregma and are mapped onto one side of the coronal schematic brain section at the level of AP −3.3 (b). f = fornix; mt = mammillothalamic tract. Calibration bar = 100 μm. Local alcohol perfusion significantly and dose-dependently increased adenosine (AD) release in the orexin-rich perifornical region (c). The chromatogram in panel (c) depicts the AD peak from a sample (10 µl) collected from the orexinergic perifornical hypothalamus during 100 mM alcohol perfusion (c). *p < 0.05 versus aCSF. aCSF Artificial cerebrospinal fluid
To summarize, based on the results of our studies described above, we suggest that alcohol increase extracellular AD in the orexin-rich perifornical hypothalamus. Increased AD acts on A1 receptors expressed on orexin neurons to inhibit them. Inhibition of orexin neurons will result in sleep promotion.
8.2 Alcohol Withdrawal Disrupts Sleep and Downregulates Orexin Expression
The next question we asked was: Are sleep disruptions observed during alcohol withdrawal due to impaired orexinergic system?
Our first experiment examined sleep-wakefulness during alcohol withdrawal. We used the extensively used (>300 citation of the original article in last 20 years) Majchrowicz’s chronic binge alcohol method to induce alcohol withdrawal in SD rats (Faingold 2008; Majchrowicz 1975). This method offer other advantages including (1) mimicking high blood alcohol levels and heavy alcohol consumption in alcoholics, (2) rapid induction of alcohol dependency and relatively high and sustained blood alcohol concentration achieved within a short duration, (3) high incidence of overt signs of withdrawal (Sharma et al. 2010).
A brief description of Majchrowicz protocol used is as follows: Two hour before the light onset, experimental animals are primed with an intragastric administration of 5 g/kg dose of alcohol. Subsequent doses are adjusted depending on the level of intoxication (assessed by observing the behavior of the animal (see Table 1 in Sharma et al. 2014b) and administered every 8 h for 4 days (treatment days). As shown in Fig. 8a, during alcohol withdrawal, rats displayed significant increase in wakefulness, both during their sleep (light) and active (dark) period with a concomitant reduction in sleep (NREM and REM) suggesting insomnia-like symptoms observed during alcohol withdrawal in humans.
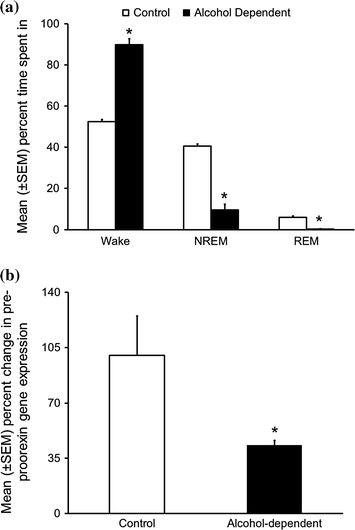
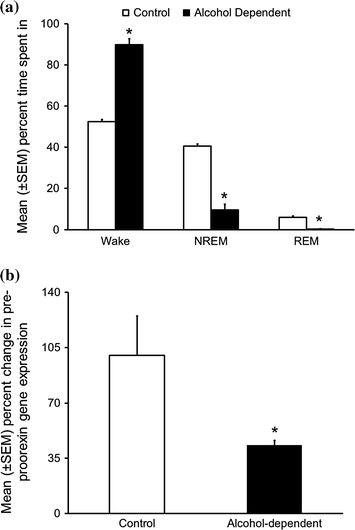
Fig. 8
Alcohol withdrawal following chronic binge alcohol exposure disrupts sleep and downregulates orexin expression. a During 24 h of withdrawal, alcohol dependent rats displayed significant increase in the amount of time spent in the wakefulness along with a concomitant reduction in NREM and REM sleep as compared to the controls. *p < 0.05 versus control. (Reproduced from Sharma et al. 2010). b As compared to controls, animals exposed to chronic binge alcohol followed by withdrawal displayed a significant reduction in the pre-proorexin gene expression in the hypothalamus. *p < 0.05 versus control. (Unpublished results)
Our next experiment examined the expression of orexin gene during acute alcohol withdrawal. The same Majchrowicz protocol (described above) was used to induce alcohol dependency in SD rats. The animals were euthanized 12 h after the last administration. At this point, blood alcohol level is almost zero and the withdrawal symptoms are at peak (Faingold 2008; Morris et al. 2010; Penland et al. 2001). The hypothalamus was rapidly dissected out and processed for quantitative Real-Time PCR to examine the expression of pre-proorexin gene (Sharma et al. 2010).
Interestingly, our preliminary/unpublished results suggested that as compared to controls, the rat undergoing alcohol withdrawal showed a significant reduction in pre-proorexin gene expression (Fig. 8b). While we are in the process of understanding the mechanisms responsible for the downregulation of orexin gene expression, we believe that chronic alcohol exposure induced perturbations in the epigenome may be responsible for reduction in orexin gene expression (Lodhi et al. 2011; Pandey et al. 2008). Downregulation of orexin gene expression is likely to result in increased REM sleep which is a common phenomenon observed in recovering alcoholics (Gillin et al. 1994).
9 Summary and Conclusion
As described above, we have gathered strong and convincing evidence suggesting that alcohol’s effects on sleep may be mediated via an interaction between AD and the orexin neurons. Interestingly, human studies suggest that acute alcohol consumption, (especially moderate to high doses) in non-alcoholics results in robust NREM sleep promotion followed by increased REM sleep during the second half of the sleep period (Roehrs and Roth 2001; Ebrahim et al. 2013). We believe that both, increased NREM sleep followed by increased REM sleep may occur only if orexin neurons are inhibited and silent. AD is one such candidate that will inhibit via its action on A1 receptors expressed on orexin neurons.
In conclusion, the results of our studies suggest that alcohol interacts and inhibits ENT1 on orexin neurons resulting in extracellular accumulation of AD. Extracellular AD will act on A1 receptors expressed on orexin neurons resulting in the inhibition of orexin neurons and sleep promotion. Thus, alcohol promotes sleep via AD induced inhibition of orexin neurons.
References
Aalto J, Kiianmaa K (1984) Increased voluntary alcohol drinking concurrent with REM-sleep deprivation. Alcohol 1(1):77–79PubMed
Adamson J, Burdick JA (1973) Sleep of dry alcoholics. Arch Gen Psychiatry 28(1):146–149PubMed
Aguayo LG, Peoples RW, Yeh HH et al (2002) GABA(A) receptors as molecular sites of ethanol action. Direct or indirect actions? Curr Top Med Chem 2(8):869–885
Alam MN, Szymusiak R, Gong H et al (1999) Adenosinergic modulation of rat basal forebrain neurons during sleep and waking: neuronal recording with microdialysis. J Physiol 521(Pt 3):679–690PubMedCentralPubMed
Alam MN, Kumar S, Rai S et al (2009) Role of adenosine A(1) receptor in the perifornical-lateral hypothalamic area in sleep-wake regulation in rats. Brain Res 1304:96–104PubMedCentralPubMed
Arrigoni E, Chamberlin NL, Saper CB et al (2006) Adenosine inhibits basal forebrain cholinergic and noncholinergic neurons in vitro. Neuroscience 140(2):403–413PubMed
Barwick VS, Dar MS (1998) Adenosinergic modulation of ethanol-induced motor incoordination in the rat motor cortex. Prog Neuropsychopharmacol Biol Psychiatry 22(4):587–607PubMed
Basheer R, Porkka-Heiskanen T, Strecker RE et al (2000) Adenosine as a biological signal mediating sleepiness following prolonged wakefulness. Biol Signals Recept 9(6):319–327PubMed
Basheer R, Halldner L, Alanko L et al (2001a) Opposite changes in adenosine A1 and A2A receptor mRNA in the rat following sleep deprivation. NeuroReport 12(8):1577–1580PubMed
Basheer R, Rainnie DG, Porkka-Heiskanen T et al (2001b) Adenosine, prolonged wakefulness, and A1-activated NF-kappaB DNA binding in the basal forebrain of the rat. Neuroscience 104(3):731–739PubMed
Basheer R, Strecker RE, Thakkar MM et al (2004) Adenosine and sleep-wake regulation. Prog Neurobiol 73(6):379–396PubMed
Batista LC, Prediger RD, Morato GS et al (2005) Blockade of adenosine and dopamine receptors inhibits the development of rapid tolerance to ethanol in mice. Psychopharmacology 181(4):714–721PubMed
Benington JH, Heller HC (1995) Restoration of brain energy metabolism as the function of sleep. Prog Neurobiol 45(4):347–360PubMed
Boissard R, Gervasoni D, Schmidt MH et al (2002) The rat ponto-medullary network responsible for paradoxical sleep onset and maintenance: a combined microinjection and functional neuroanatomical study. Eur J Neurosci 16(10):1959–1973PubMed
Borbely AA (1982) A two process model of sleep regulation. Human Neurobiol 1:195–204
Bouchery EE, Harwood HJ, Sacks JJ et al (2011) Economic costs of excessive alcohol consumption in the U.S., 2006. Am J Prev Med 41(5):516–524PubMed
Breese GR, Criswell HE, Carta M et al (2006) Basis of the gabamimetic profile of ethanol. Alcohol Clin Exp Res 30(4):731–744PubMedCentralPubMed
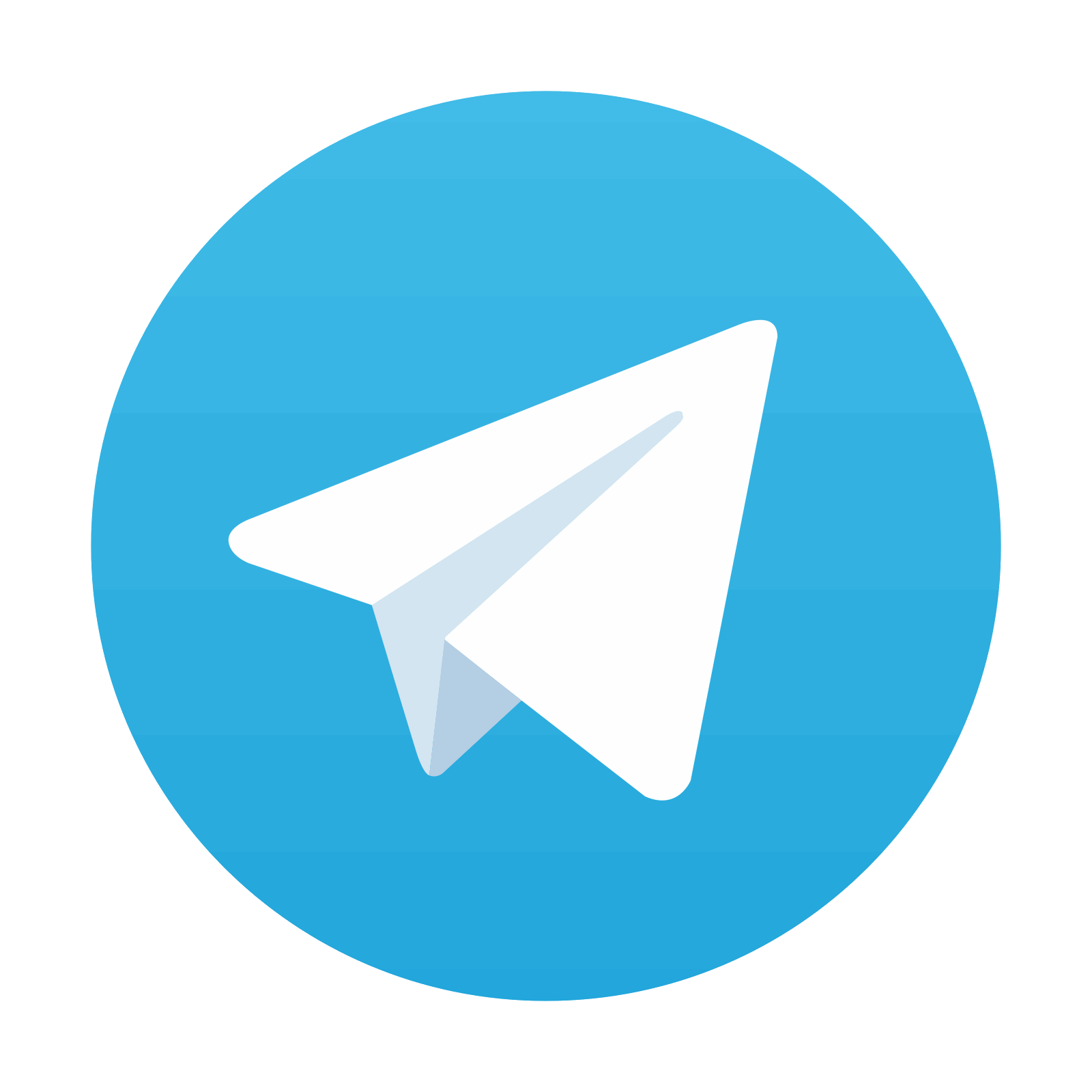
Stay updated, free articles. Join our Telegram channel

Full access? Get Clinical Tree
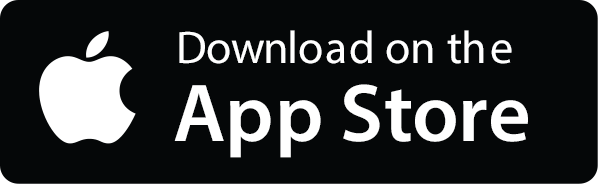
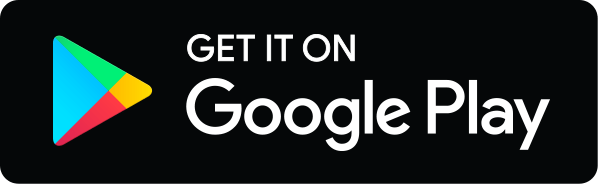