© Springer Science+Business Media New York 2014
Vladimir Parpura and Alexei Verkhratsky (eds.)Pathological Potential of Neuroglia10.1007/978-1-4939-0974-2_55. Alexander Disease and Astrotherapeutics
(1)
Department of Neurobiology and the Civitan International Research Center, University of Alabama at Birmingham, SRC R552, 1530 3rd Avenue South, 35294-0021 Birmingham, AL, USA
(2)
Department of Comparative Biosciences, School of Veterinary Medicine, University of Wisconsin-Madison, Waisman Center, Rm 713, 1500 Highland Ave, 53705 Madison, WI, USA
Abstract
Alexander disease is a protein aggregation disorder resulting from mutations in the intermediate filament protein, GFAP. Progress in the past 15 years has defined numerous aspects of astrocyte function that are impacted by these mutations, and that might be amenable to correction. Since reversal of astrocyte dysfunction is likely to be valuable in a wide variety of conditions, Alexander disease offers unique opportunities for exploring the newly emerging field of astrotherapeutics.
Keywords
Alexander diseaseNeurodegenerative disorderGFAPGFAP mutationsAstrocytesAstrotherapeuticsProtein aggregates5.1 Alexander Disease: Clinical Manifestations and Etiology
Alexander disease (AxD) is a usually fatal neurodegenerative disorder resulting from astrocyte dysfunction (for reviews, see (Brenner et al. 2009; Flint and Brenner 2011; Messing et al. 2012a)) . Its defining feature is the abundant presence in astrocytes of protein aggregates , called Rosenthal fibers, which are especially prevalent in subpial, periventricular and perivascular locations . Historically, AxD has been classified as a pediatric leukodystrophy because it was first and then most often diagnosed in young children, and because in this age group it is accompanied by massive myelination defects. Common clinical manifestations of childhood AxD include failure to meet mental and physical developmental milestones, megalencephaly, seizures , hyperreflexia and pseudobulbar signs, such as difficulty speaking and swallowing. Magnetic resonance imaging (MRI) criteria are highly diagnostic for childhood AxD, with a major feature being striking myelination defects bilaterally in the frontal lobes (van der Knaap et al. 2001) . The classification of AxD was extended to adult cases based on similar findings of profuse astrocytic protein aggregates, despite these patients often having symptoms quite different from the childhood disorder. Adult AxD patients typically display normal development, normal head size, and rarely have seizures. They do share hyperreflexia and pseudobulbar signs with the infantile cases, but more uniquely often have ataxia, visual and autonomic abnormalities, sleep disturbances and palatal myoclonus. For both the childhood and adult forms, no single symptom is present in all cases, and both may also display multiple other symptoms with lesser frequencies (Prust et al. 2011; Yoshida et al. 2011) . MRI diagnosis of adult cases is usually much more difficult than for children, since many of the criteria that apply for children do not hold for adults (van der Knaap et al. 2006) . In particular, instead of the frontal predominance of pathology seen in the childhood cases, the pathology in adult AxD is typically more caudal, involving the brain stem, cerebellum and cervical spinal cord. Importantly, white matter lesions are not found in about half of the adult onset cases (Yoshida et al. 2011) . Accordingly, AxD is now better considered as an astrogliopathy rather than a leukodystrophy.
Until recently, AxD was divided into three categories: infantile, with onset before 2 years of age; juvenile, with onset between 2 and 12 years; and adult, with onset at 13 years of age or later (Russo et al. 1976) . The infantile and adult onset cases usually differed from each other as noted above, whereas the juvenile cases could be like either of these, or display selected symptoms of each. Statistical analysis of 215 cases by Prust et al. (2011) found the data are better fit by a two class model rather than by three classes, Type I having an early onset and largely mirroring the infantile form, and Type 2 usually having a later onset and largely mirroring the adult form. Average age at onset for Type I was 1.7 years, and average lifespan following onset was 14 years; for Type 2 average onset was at 22 years, and average lifespan thereafter was 25 years. Here we adopt this Type I and Type 2 classification, which are also referred to as early onset and late onset.
Due to the lack of specificity of its clinical presentations, the late onset form of AxD was diagnosed much less frequently than the early onset form until the discovery that approximately 95 % of both are due to heterozygous missense mutations in the gene encoding glial fibrillary acidic protein (GFAP) , the primary and largely cell-specific intermediate filament protein in astrocytes (Brenner et al. 2001; Rodriguez et al. 2001; Gorospe et al. 2002; Li et al. 2005) . With the advent of this simple genetic diagnostic, adult cases are now discovered at a frequency similar to or even greater than early onset cases (Pareyson et al. 2008; Yoshida et al. 2011) , and include both de novo and familial occurrences. The cause of the remaining 5 % of cases for which a GFAP mutation has not been discovered is unknown. Since overexpression of wild type GFAP can produce AxD-like pathology in a mouse model (Messing et al. 1998) , the possibility of GFAP gene duplication has been examined for several patients without a detectable mutation, but with negative results (Yoshida and Nakagawa 2012) . The overall incidence of AxD has been estimated to be on the order of one in a million (Yoshida et al. 2011) .
The disease-causing mutations have been found throughout the length of the protein, with the exception of the non-helical N-terminal domain, and nearly all are 100 % penetrant. Genotype/phenotype correlations have been established for a few of the mutations and the clear absence of a correlation for several others, but the majority have been too rare for statistical analysis. As examples, mutations at R79 and R239 are associated with early onset and poor prognosis, whereas those at R88 and R416 have no correlation with age of onset or severity, suggesting other contributing factors to disease severity (Prust et al. 2011) . The GFAP mutations appear to act by a dominant, gain of function mechanism, since mice lacking GFAP have only mild deficits which do not resemble AxD (Gomi et al. 1995; Pekny et al. 1995; McCall et al. 1996) . This has implications for gene therapy, since provision of wild type GFAP to AxD patients is unlikely to be beneficial, and might instead exacerbate disease by increasing the GFAP load.
5.2 Disrupted Functions of Alexander Disease Astrocytes and Their Links to GFAP Mutations
What critical function (or functions) of astrocytes is disrupted in AxD, and how GFAP mutations cause that disruption, are active areas of research, which is being pursued by analysis of human tissues, by cell free assays, study of primary and stable cell lines, and use of Drosophila and mouse models. All these studies involve comparing the effects of expressing wild type and mutant GFAP. Functions found altered include decreased proteasomal activity, decreased plasma membrane glutamate transport [through GLT-1 (rodents)/EAAT2(human), commonly named (as per HUGO gene nomenclature committee) solute carrier family 1 (glial high affinity glutamate transporter), member 2 (SLC1A2)], increased autophagy, activation of the stress-activated protein kinase/c-Jun N-terminal kinase (JNK) pathway; presence of advancedglycation and lipid peroxidation end products, and activation of an oxidative stress response and iron accumulation (Hagemann et al. 2006, 2009; Tang et al. 2006; Castellani et al. 1997, 1998; Tang et al. 2008; Tian et al. 2010; Wang et al. 2011; Sosunov et al. 2013) . In addition, AxD astrocytes are chronically reactive (Sosunov et al. 2013) , and thus likely to be secreting cytokines such as tumor necrosis factorα and interleukin1β, which can be toxic to other cells. Addressing any of these altered functions could potentially be therapeutic.
A mechanistic linking of GFAP mutations to proteasomal inhibition and stress pathway activation has been proposed by Tang et al. (2006) on the basis of cell culture studies. They found that expression of mutant GFAP inhibits proteasome function, that direct inhibition of proteasome function activates JNK, and that direct activation of JNK inhibits proteasome activity. This prompted the proposal of a positive feedback cycle in which mutant and/or increased GFAP inhibits proteasome activity, which in turn activates JNK, which further inhibits proteasome activity, resulting in yet higher GFAP levels. This is an attractive model because it links the primary defect in AxD, mutant GFAP, to physiological changes. It also could explain why mutations present from conception might not have consequence until later in life—effects might remain subthreshold until some central nervous system (CNS) perturbation increases GFAP above a critical level that sets the positive feedback loop in motion. However, what players in the loop contribute to the clinical manifestations of the disease remains unclear.
Another proposed mechanism linking GFAP mutations to the disease process is inactivation of one or more critical proteins through sequestration in the Rosenthal fibers, a mechanism that has been proposed for other neurodegenerative disorders (Ali et al. 2010) . Although GFAP is a major constituent of Rosenthal fibers, also associated with these aggregates are the small stress proteins HSP27 and αB-crystallin, the 20S proteasome subunit, plectin, p-JNK and p62 (Iwaki et al. 1993; Head et al. 2000; Zatloukal et al. 2002; Tang et al. 2006; Tian et al. 2006) . Proteomic studies of Rosenthal fibers are currently underway to identify other candidate proteins (Heaven and Brenner, manuscript in preparation) . Sequestration of the 20S proteasome has been suggested as a contributor to impaired proteasome activity in AxD, although as discussed below, it is also inhibited by a soluble GFAP oligomer (Tang et al. 2010) .
Both sequestration of αB-crystallin in the Rosenthal fibers and its ability to debundle GFAP filaments (Koyama and Goldman 1999; Perng et al. 1999; Nicholl and Quinlan 1994) led to it being tested therapeutically in a mouse model of AxD. This model consists of mice that both overexpress human GFAP from a transgene and have a knocked-in GFAP R236H mutation, which is the mouse homologue of the particularly pernicious human R239H mutation. Separately, the overexpression and the knock-in each display some characteristics of AxD, including formation of Rosenthal fibers, activation of stress responses and seizure susceptibility, but have normal lifespans. When combined together, however, they produce lethality between about 25 and 35 days of age, presumably from seizures (Hagemann et al. 2006) . In this lethal AxD mouse model, expression of αB-crystallin in astrocytes from a GFAP promoter-driven transgene to further increase the already elevated level present in the disease models resulted in complete rescue of viability, reduction of the stress response and partial restoration of GLT-1 levels (Hagemann et al. 2009) . These marked and general therapeutic effects thus recommend elevation of αB-crystallin for further investigation as an AxD treatment. Indeed, systemic delivery or increased expression of αB-crystallin may have general applicability beyond AxD, as it appears protective in models of neuroinflammatory disease and stroke (Ousman et al. 2007; Arac et al. 2011; Shao et al. 2013) . However, there are concerns about αB-crystallin as a therapy, primarily due to its identification as a negative risk factor in glioblastomas and several other types of cancer (Goplen et al. 2010) . Pharmacological strategies for enhancing αB-crystallin expression would not be tissue specific, and therefore enhancing αB-crystallin expression may be challenging to achieve without unacceptable side effects unless an astrocyte-specific delivery method could be developed, such as with viral vectors (see below).
Presumably the causes of the pathological changes in AxD astrocytes have as their origin a misfolded state of GFAP, but the identity of the toxic species, and whether it is the same immediate cause for each of the pathological changes, is not known. Correlations between the presence of Rosenthal fibers and disturbance of astrocyte functions have led to the tacit assumption amongst most investigators that Rosenthal fibers are the toxic species. However, by analogy with other protein aggregate neurodegenerative disorders such as Alzheimer’s disease, Parkinson’s disease and Huntington’s disease, the aggregate may be benign, and a smaller oligomer (which may be on the pathway to aggregate formation) may cause toxicity (Caughey and Lansbury 2003) . Support for this has been obtained by Tang et al. (2010) with regard to proteasome inhibition. They found that 20S proteasome activity was inhibited more severely by in vitro polymerized R239C mutant GFAP than by wild type GFAP, and that an oligomeric fraction was much more potent than the filament fraction. Native gel separation of the wild type oligomer fraction indicated that it contained primarily monomers and tetramers, whereas the R239C oligomers were primarily tetramers and a more slowly migrating species that could be an octamer. Exposure to αB-crystallin converted the R239C size distribution to that of the wild type, and also reduced proteasome inhibition, suggesting that the larger oligomer is a primary inhibitory species, and that a therapeutic activity of αB-crystallin is to prevent its formation. If the toxic species is indeed an oligomer rather than the Rosenthal fiber aggregate, the possibility exists that treatments targeting dissolution of the Rosenthal fibers could contrarily exacerbate disease by increasing oligomer levels.
Studies in Drosophila have produced indications of what may be primary and what may be secondary pathological effects in AxD. Although Drosophila have neither astrocytes nor GFAP , targeting mutant GFAP expression to Drosophila glia produced many of the phenotypes of AxD, including formation of Rosenthal fiber-like protein aggregates , loss of glia and neurons, susceptibility to seizures , and activation of stress pathways (Wang et al. 2011) . As in the mouse studies, over-expression of αB-crystallin decreased cell death, seizure susceptibility and aggregate formation, indicating that it acts at a fundamental level to reverse the AxD phenotype. On the other hand, although toxicity was decreased by elevating levels of catalase or superoxide dismutase to reduce oxidative stress, or by increasing plasma membrane glutamate transport, there was no effect on the burden of GFAP aggregates, suggesting that these treatments address downstream effects of the primary lesion. Also of therapeutic interest, the Drosophila studies found benefit from two compounds available for human use, vitamin E and 17-(allylamino)-17-demethoxygeldanamycin (17-AAG, an Hsp90 inhibitor that stimulates degradation of select proteins, and is undergoing clinical trials for cancer treatment).
5.3 Treatment Strategies
Thus for treatment strategy, reducing GFAP levels by inhibition of synthesis or increased degradation is likely to be most efficacious, followed by prevention of misfolding by agents such as αB-crystallin, followed by treatment of downstream consequences such as activation of stress pathways and reduced plasma membrane glutamate transport. Both mouse and cell culture studies (Koyama and Goldman 1999; Mignot et al. 2007) (Messing unpublished observations) have found that Rosenthal fibers can disappear with time; thus to the extent that Rosenthal fibers serve as an indicator for the toxic GFAP species, limiting GFAP levels may not only prevent disease progression, but also reverse some symptoms. There is little concern about possible deleterious effects from extreme reduction in GFAP levels, since GFAP knockout mice have minimal neurological deficits. Tolerance of reduced GFAP expression in humans is suggested by the finding that 4 of 2026 healthy subjects were found heterozygous for deletion of the GFAP gene (Shaikh et al. 2009) .
5.3.1 Reduction of GFAP Levels
In theory, GFAP synthesis could be curtailed by inhibiting participating transcriptional activators, but the transcriptional regulation of the gene is poorly understood. Many studies have been performed using cell culture and in the context of developmental activation of the gene. However, Yeo et al. (2013) have shown that cell culture studies of GFAP transcription are poor prognosticators of in vivo regulation; and factors necessary for activating GFAP transcription during development may act indirectly, and have no role in transcription in the adult (Herrera et al. 2012) . Studies examining GFAP transcription in mice have identified AP-1, GATA, NF-1, NF-κB, SP-1 and STAT-3 as contributing to its activity, with others yet to be discovered (Steele-Perkins et al. 2005; Herrmann et al. 2008; Yeo et al. 2013) . None of these is specific to GFAP transcription, making it likely that their inhibition would have unintended consequences. Indeed, general knockout of any of these six transcription factors is embryonic lethal or severely debilitating in mice (Johnson et al. 1993; Steele-Perkins et al. 2005; Fujiwara et al. 1996; Marin et al. 1997; Takeda et al. 1997; Weih et al. 1997) . Perhaps partial inhibition of a combination of these transcription factors would be safe and efficacious, but we are unaware of such an approach having been used for reducing the activity of any gene.
An alternative, gene-specific method for reducing transcription is through use of inhibitory RNA (RNAi) or oligonucleotides. While this approach has been routinely used in cell culture, recently there has been exciting progress in the development of in vivo applications of this technique, and clinical trials for several diseases are now underway (Southwell et al. 2012) . For CNS disorders, systemic delivery faces the usual hurdle of the blood-brain barrier , but recent work on models of Huntington’s disease using intrathecal administration show remarkably long-lasting suppression by relatively short term treatments with antisense oligonucleotides (Kordasiewicz et al. 2012) . In Huntington’s disease there is concern that global suppression would itself cause problems, based on the embryo lethality associated with the null state of huntingtin in the mouse (Duyao et al. 1995; Nasir et al. 1995; Zeitlin et al. 1995) . In the case of AxD, it may be possible to specifically reduce expression of the mutant allele while preserving expression from the wild type allele, although this would be technically challenging since it would require the development of appropriate reagents for each of the more than 80 mutations now associated with disease. However, as noted above, general knock-down of GFAP expression is likely to be safe, recommending AxD as an excellent setting in which to explore the therapeutic value of antisense oligonucleotides.
Another approach already taken to reduce GFAP levels was a screen of small libraries of FDA-approved drugs. This was done using primary cultures of astrocytes prepared from a novel dual-luciferase reporter strain of mice (Cho et al. 2010) . Several compounds were identified that met the criteria designed as hits for this study, but none of them has yet proved useful for reducing GFAP expression in the AxD mouse models (Messing unpublished observations) . It should be noted that the astrocytes used in this screen expressed only wild type GFAP, and not the point mutants that are associated with disease, which will induce a reactive response. Although cultured neonatal astrocytes exhibit some properties of the reactive state (Nakagawa and Schwartz 2004; Cahoy et al. 2008; Zamanian et al. 2012) , they do so only partially, and the transcriptional elements responsible for reactive upregulation of GFAP likely differ from those that control its basal level of expression (Yeo et al. 2013) . In addition, subsequent comparisons of the dual-luciferase reporter with a single luciferase reporter demonstrate that expression from the latter correlates much more closely with GFAP mRNA (Jany et al. 2013) . These two reporters differ in that a 2.2 kb human GFAP promoter fragment was used for the dual luciferase reporter, whereas a 12 kb mouse GFAP promoter was used for the single GFAP reporter. This finding and studies in progress (Brenner unpublished observations) indicate that important transcriptional elements are present in the 2.2–12 kb upstream region. Newer drug screens are now underway that utilize astrocytes derived from mice expressing the R236H point mutant of GFAP along with the more representative single luciferase reporter.
An alternative to inhibiting GFAP synthesis is to increase its degradation. Since inhibition of proteasome activity by mutant GFAP may be the initiating event in astrocyte dysfunction through activation of stress responses (Tang et al. 2010) , stimulating proteasomal activity might not only reduce GFAP levels, but also attenuate the pathological response. On the other hand, the one mutant GFAP examined, R239C, was a poor substrate for proteasomal degradation (Tang et al. 2010), suggesting that increasing proteasomal activity may have little benefit. Also of concern is the finding that proteasomal inhibition unexpectedly decreases GFAP levels in cultured astrocytoma cells and rat brain, presumably due to preservation of a short-lived transcriptional repressor (Middeldorp et al. 2009) . This raises the possibility that proteasome activation could actually increase GFAP levels and exacerbate AxD. Therapies that seek to inhibit proteasome activity are receiving major attention for other disorders, but those that seek to increase this activity are only in the early stages of development (Huang and Chen 2009) .
GFAP degradation could also be augmented by stimulating autophagy. Increased numbers of autophagic vacuoles are already present in AxD patients, in the R236H mouse model and in transfected cells expressing R239C GFAP (Tang et al. 2008) . However, no increase in protein degradation was observed in the cell culture systems, raising the possibility that the increase may be compensation for a partial inhibition of autophagic flux or proteasomal activity, with the net result of reestablishing the normal steady state rate of degradation. Nevertheless, inhibiting autophagy pharmacologically with 3-methyadenosine or genetically with knockout of the Atg5 gene led to accumulation of GFAP expressed from a CMV promoter, whereas activating autophagy by starvation, rapamycin, or decreasing mammalian target of rapamycin (mTOR) levels reduced GFAP levels, indicating that GFAP is an autophagy substrate and that increasing utilization of this pathway could be beneficial. Another player affecting autophagy is p38, whose activation was found to increase autophagy markers and reduce GFAP levels. Activation of p38 could occur through stimulation of the mitogen-activated protein kinase (MAPK) stress pathway already mentioned. As with proteasomal activation, a caveat for therapeutic efficacy of increased autophagy is that it might also increase GFAP levels through removal of a transcriptional repressor (Middeldorp et al. 2009) . No attempt has been made to treat AxD by stimulating autophagy, although this is being investigated for other neurodegenerative disorders (Hochfeld et al. 2013) .
Another possible route to reducing GFAP levels was discovered while pursuing the role in AxD of Nrf2, a transcriptional activator that regulates a large repertoire of genes associated with the response to toxic injury and oxidative stress. Activation of this pathway was first suggested by microarray analysis of olfactory bulb from the GFAP over-expressing mouse model of AxD, and subsequently confirmed by studies on tissues from human patients (Hagemann et al. 2005) . Enhancing expression of Nrf2 showed clear evidence for protection in mouse models of amyotrophic lateral sclerosis (ALS) and α-synucleinopathy (Vargas et al. 2008; Gan et al. 2012) . Most recently, beneficial effects of forcing over-expression of Nrf2 for the mouse models of AxD have been observed, with striking reductions in the expression of GFAP itself and other indicators of pathology in several areas of the CNS (LaPash Daniels et al. 2012) . Surprisingly, however, placing the AxD models in the Nrf2-null background did not make the phenotype worse, and even reduced evidence of gliosis in the hippocampus (Hagemann et al. 2012) . A similar pattern of protection from over-expression, but minimal effect from the null state, has also been observed with the ALS models (Guo et al. 2013; Vargas et al. 2013) . The beneficial effects of increasing Nrf2 are of particular interest, since a drug that activates this pathway, BG-12, has recently been approved for use in multiple sclerosis (Scannevin et al. 2012; Linker et al. 2011; Stangel and Linker 2013) . A clinical trial of BG-12 in AxD patients is therefore worth consideration.
5.3.2 Other Treatment Targets and Patient Testing
A decrease in GLT-1/EAA-2 as found in AxD also occurs in other neurodegenerative disorders , and may be associated with the astrocytic reactive response (Tian et al. 2010) . GLT-1/EAAT-2 is the major glutamate transporter in astrocytes, and deficiency in glutamate transport causes seizures and excitotoxic death of both neurons and oligodendrocytes (Tanaka et al. 1997) . Presumably with the rationale of restoring EAAT-2 levels, Sechi and colleagues treated a 39 year old late onset AxD patient with ceftriaxone, an antibiotic identified as a potential therapeutic for ALS from a drug screen seeking enhancers of GLT-1 expression (Rothstein et al. 2005) . The patient showed apparent improvement in some of her symptoms over a 20-month period (Sechi et al. 2010) . More recently, this same group provided 4-year follow up data (~ 2 additional years of treatment) on the same patient, and claim continued improvement, particularly with respect to gait ataxia, dysarthria, and palatal myoclonus (for which blinded assessments were performed) (Sechi et al. 2013) . However, these studies do not take into account the spontaneous remissions that have occasionally been reported in AxD patients, particularly those with bulbar signs (Messing et al. 2012b; Namekawa et al. 2012) . Whether any other patients have successfully been treated with this drug is not known.
Despite this apparent success, there are several uncertainties concerning these findings with ceftriaxone. Although selected for its ability to elevate GLT-1 in test systems, the AxD patient treated had had no history of seizures, and in some models ceftriaxone has neuroprotective effects without any change in GLT-1 expression (Melzer et al. 2008) . One hypothesis is that ceftriaxone might activate the Nrf2 pathway (Lewerenz et al. 2009; Nizzardo et al. 2011) , one of the stress pathways shown to have beneficial effects in AxD as discussed above (LaPash Daniels et al. 2012) . However, in our own studies with ceftriaxone administration to mouse models of AxD we have been unable to find effects on GLT-1 expression, protection from lethality, or activation of protective stress pathways (Messing unpublished observations) . Seizures are a known side effect from ceftriaxone, raising the questions of whether it is suitable for use in patients who already have seizures as one of their symptoms.
Ishigaki and colleagues treated a 9 year old type II AxD patient with thyrotropin releasing hormone (TRH) based on its efficacy in treating cerebellar defects in mice (Ishigaki et al. 2006) . Intravenous administration followed by oral dosing mitigated some of the symptoms in this child, although the effectiveness diminished over the 4 month period in which she was observed. More recently, in the context of a broad review on AxD, Yoshida and colleagues briefly mention that two other patients have been treated with TRH, without benefit (Yoshida et al. 2011) . No other details were provided about these additional patients.
The spice curcumin has been tested in a cell culture system for its effects on aggregate formation. The rationale was that curcumin had been shown in other systems to increase levels of αB-crystallin and HSP27 in cells, to inhibit or activate proteasome activity depending on its concentration, to activate autophagy, and to reduce protein aggregate formation. In the AxD study, the effect of curcumin was tested in cells transfected with an R236C expression vector. Promising data were obtained indicating that curcumin could indeed increase both αB-crystallin and HSP27 levels in a glioma cell line and decrease GFAP expression. On the other hand, the absence of clear dose-response effects, a very narrow effective dose window, and variability of outcomes with time after treatment provide notes of caution. Additional mechanistic studies in cell culture and testing in animal models will be of interest. The safety of using curcumin has recently been questioned by Foxley et al. (2013) , who found that CNS lupus erythematosus was aggravated by curcumin in a mouse model.
5.3.3 Treatment Delivery Methods
Whether treatment is by drugs or gene therapy, the therapeutic agent would either need to be delivered intracerebrally, or be able to cross the blood-barrier if given systemically. Systemic administration is certainly preferred for ease of delivery and safety concerns, especially since treatments may need to be chronic. Recent reports for recombinant adeno-associated virus (rAAV) suggest this may be feasible not only for drug treatments, but also for gene therapy. Foust et al. (2009) report that a single intravenous injection via the tail vein of an adult mouse resulted in transfection of about 64 % of astrocytes in the lumbar region of the spinal cord, while producing minimal neuronal labeling. They also observed, but did not quantify, extensive transgene activity in astrocytes throughout the brain. In contrast to the vascular route of delivery, this group found that direct injection of the rAAV9 into the striatum and dentate gyrus resulted primarily in neuronal labeling, suggesting that when delivered intravenously, astrocytes are labeled via their endfeet association with endothelial cells. This infection pathway might limit the percentage of astrocytes that can be genetically engineered as not all astrocytes have contact with blood vessels. On the other hand, those astrocytes which do associate with blood vessels (perivascular) are a prime site for Rosenthal fibers, and there is also a suggestion that reactive astrocytes are more prone to AAV transfection than resting astrocytes (Klein et al. 2008) . Thus rAAV infection could have some specificity for those astrocytes affected by AxD. In contrast to the results of Foust et al. (2009) , von Jonquieres et al. (2013) observed extensive astrocytic expression of rAAV1/2 in astrocytes delivered to P0 mice by striatal injection, with cell specificity being conferred by use of a GFAP promoter. In the immediate region of the injection site approximately half the astrocytes expressed the GFP transgene, and this number increased to approximately 65 % when P90 mice were injected. It is unclear whether this different result for intracerebral delivery is attributable to the different AAV serotype or some other variable.
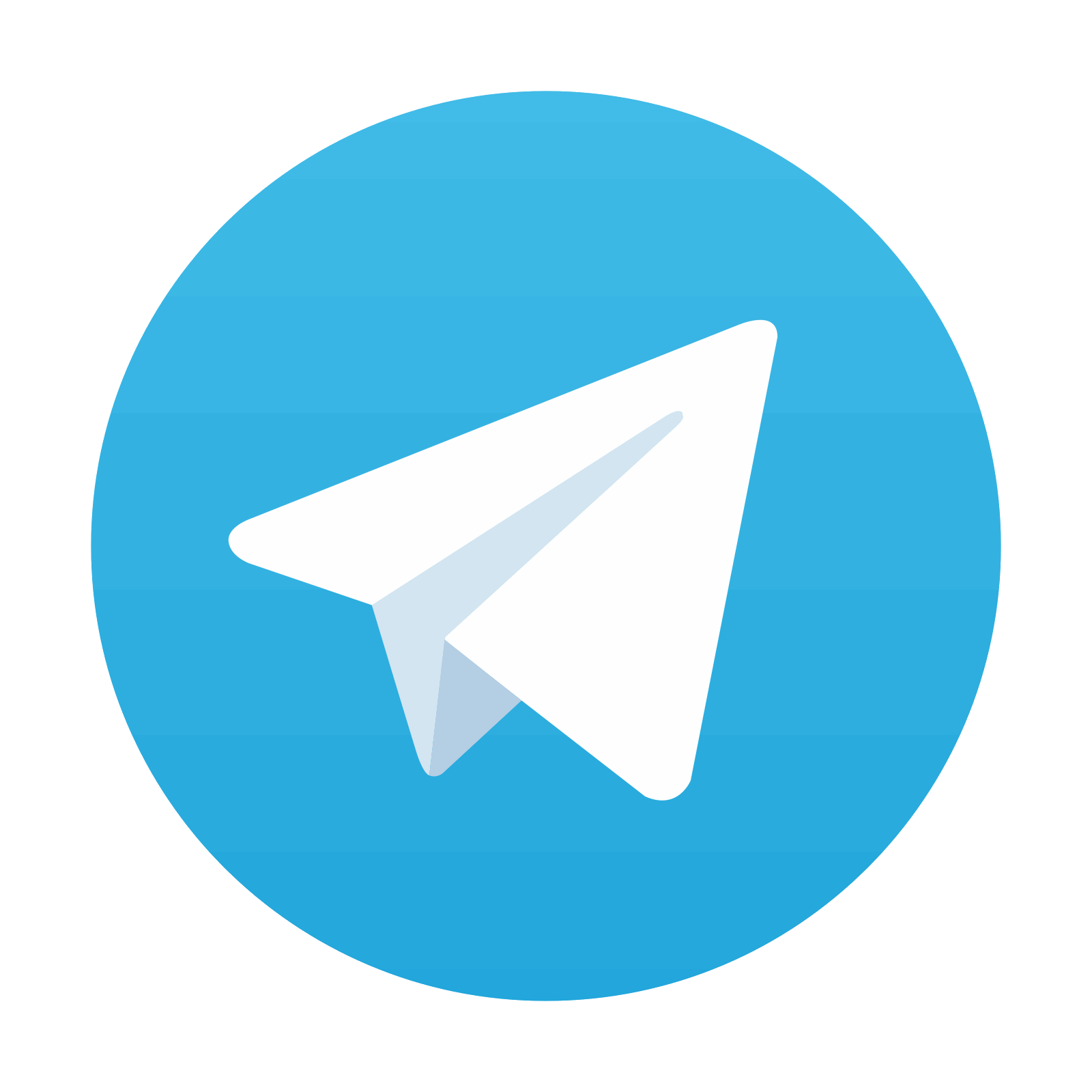
Stay updated, free articles. Join our Telegram channel

Full access? Get Clinical Tree
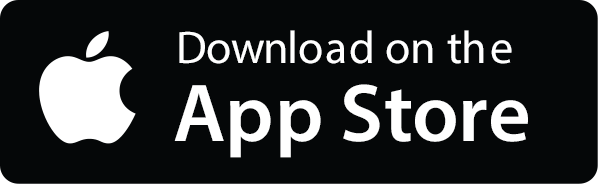
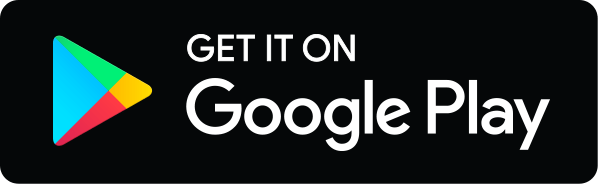