Fig. 22.1
Schematic depicting the GFAP gene, the corresponding protein and localization of GFAP mutations identified in patients with Alexander disease
The R416W GFAP mutation is located in the tail domain (Fig. 22.1), which is conserved between all type III intermediate filament proteins and is thought to play a role in stabilizing protofibrillar interactions and filament diameter (Fuchs 1996). Furthermore, R416W GFAP would be expected to affect interactions with other cytoskeletal elements (Quinlan 2001) and be a cause of Alexander disease, as well as a residue of the point mutation in the tail domain of other intermediate filament, desmin, which causes idiopathic dilated cardiomyopathy (Cary and Klymkowsky 1995; Li et al. 1999). Recently, Perng et al. demonstrated that the R416W GFAP mutant disrupted normal filament assembly in vitro, and that protein chaperones such as αB-crystallin and HSP27 were specifically associated with GFAP aggregates in R416W GFAP cells (Perng et al. 2006). In our study, the proportion of abnormal GFAP structures in R416W cells was also significantly higher than that in the wild-type cells, while the velocity of R416W cell migration was not significantly different from the wild-type cells. The time-lapse recording findings revealed that many cells expressing R416W GFAP were able to undergo cell division, although the filamentous structure of these cells was apparently disrupted. These results suggest that intrafilament disruption could cause morphological alteration, and that the residue of point mutation in the tail domain per se may not be a direct determinant of the interaction with extracellular components. Our results suggested that these cells could maintain the fundamental structure of GFAP, and that the alteration of R416W GFAP function in astrocytes depended on other proteins that interact with GFAP, suggesting that the phenotype of mutant GFAP in the tail domain has a variety of clinical features of Alexander disease with varying severity (Brenner et al. 2001; Kinoshita et al. 2003; Li et al. 2005).
The fact that the same mutation can cause different phenotypes suggests that the phenotype is influenced not only by structural and functional changes induced by GFAP mutations, but is also affected by other components, including genetic, environmental, embryological, and GFAP-regulating factors. The current data suggest that a combination of events contribute to Alexander disease. Mitochondrial abnormalities are one consideration, because few reports have described a relationship between Alexander disease and mitochondrial abnormalities. Gingold et al. (1999) reported a female patient who was clinically diagnosed initially with Leigh disease, then later was pathologically confirmed as having Alexander disease due to the finding of a massive deposition of Rosenthal fibers. Schuelke et al. (1999) reported a female patient who showed the phenotype of Alexander disease and an NDUFV1 homozygous mutation, which encodes a component of the mitochondrial complex I. A patient with an R88C GFAP mutation also showed mitochondrial DNA abnormalities, an A-to-G homoplasmic transition at np8291 and a 9-base pair deletion between np8272-8280 (Nobuhara et al. 2004). Plectin, which is a member of the plakin family of cytolinker proteins and is localized at the cytoskeleton-plasma membrane interface, is another potential candidate contributing to Alexander disease development. Tian et al. (2006) reported that the reduced levels of total plectin in response to mutant GFAP expression in astrocytes can promote the abnormal organization and aggregation of GFAP into Rosenthal fibers. Among the minor isoforms of GFAP, GFAP-δ/ε alters the binding of αB-crystallin to GFAP filaments (Perng et al. 2008). GFAP-δ/ε mRNA expression is the result of alternative splicing of intron 7, with variable use of exon 7a, and skipping exons 8 and 9 (Nielsen et al. 2002). GFAP aggregates induced by R239C mutation aggravate the effects of GFAP accumulation in inducing the intracellular stress response (Tang et al. 2008). Most recently, Tang et al. (2010) have reported that GFAP aggregates induced by R239C mutation inhibited the proteasomal system, and that αB-crystallin reinstated proteasomal degradation and inhibited the accumulation of GFAP.
In summary, GFAP point mutations lead to structural changes, and the mechanism of GFAP aggregation depends on the domain in which the point mutation is located and may explain the differences in clinical features observed in these patients. Because many clinical features of Alexander disease of varying severities have been attributed to each mutation, other factors that modify the clinical features of Alexander disease must be present. The identification of the factors will suggest new considerations for reducing the severity of Alexander disease, and may eventually lead to a therapy for the treatment of patients with Alexander disease. A different approach to Alexander disease therapy should be considered according to changes in the GFAP domains.
References
Brenner M, Johnson AB, Boespflug-Tanguy O, Rodriguez D, Goldman JE, Messing A (2001) Mutations in GFAP, encoding glial fibrillary acidic protein, are associated with Alexander disease. Nat Genet 27:277–286CrossRef
Cary RB, Klymkowsky MW (1995) Disruption of intermediate filament organization leads to structural defects at the intersomite junction in xenopus myotomal muscle. Development 121:1041–1052PubMed
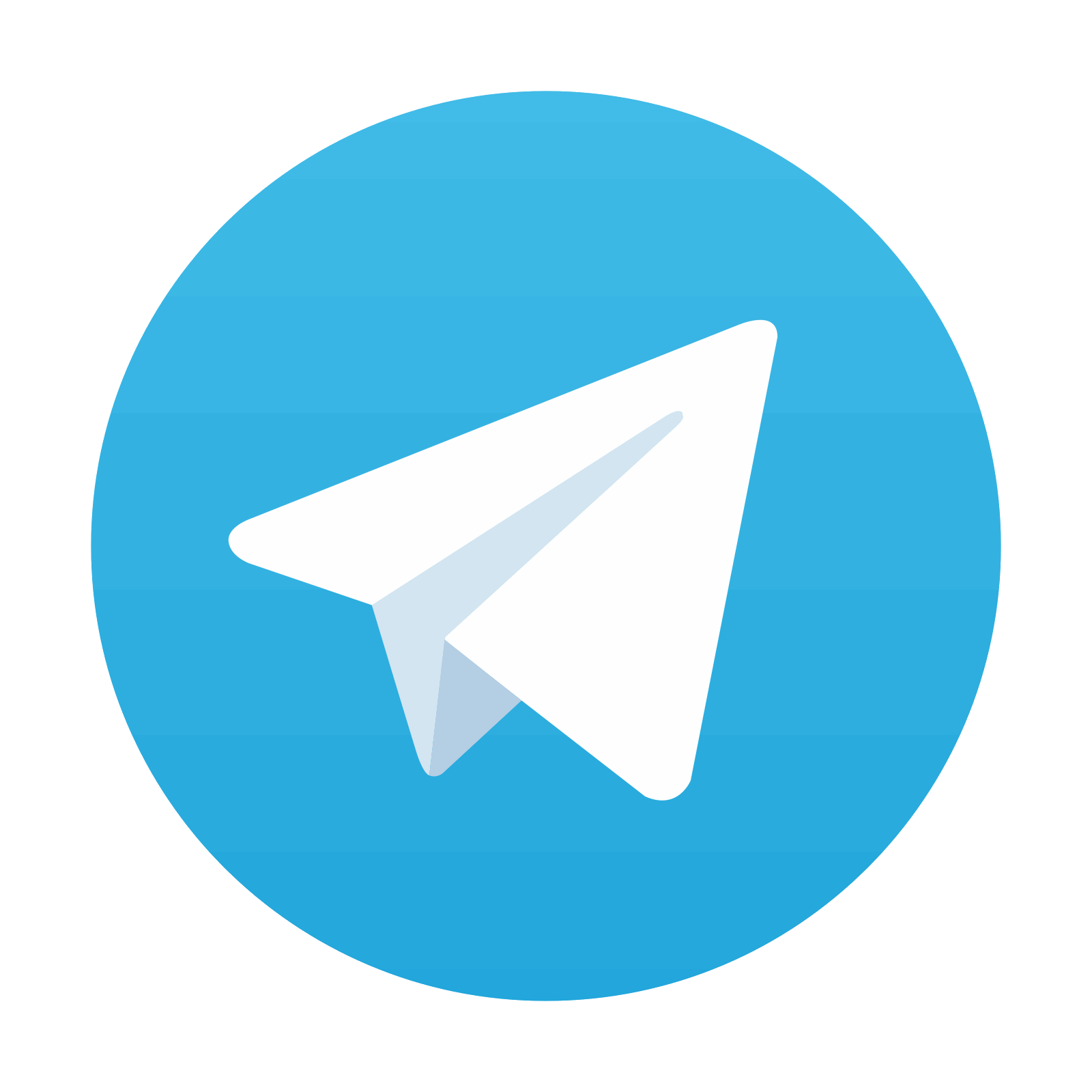
Stay updated, free articles. Join our Telegram channel

Full access? Get Clinical Tree
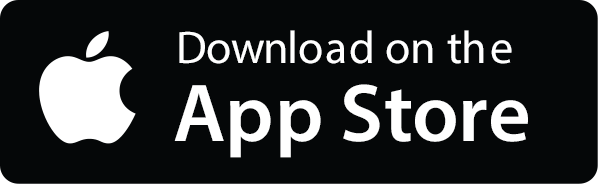
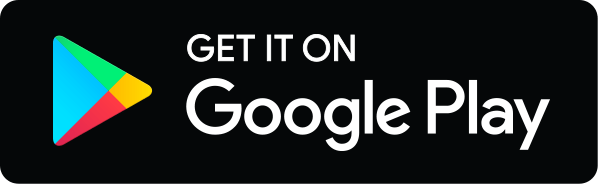