Neurosensory recovery based upon Sunderland classification
Need for surgery
Sunderland
Recovery pattern
Rate of recovery
I
Complete
Fast (days–weeks)
None
II
Complete
Slow (weeks)
None
III
Variable
Slow (weeks–months)
Maybe
IV
Poor
Little/None
Yes
V
None
None
Yes
Table 7.2
Advantages and disadvantages of nerve grafts for the repair of peripheral nerve injury
Nerve transplant | Advantages | Disadvantages |
---|---|---|
Nerve autograft | Provides a suitable environment for nerve regeneration | Limited amount of tissue |
No risk of immunological rejection | Limited number of grafts | |
Simple and safe to obtain | Donor site morbidity and potential loss of function | |
Easy to suture to the injured tissue | ||
Nerve allograft | Unlimited source tissue | Lack of appropriate animal donor tissue |
No donor site trauma for the recipient | Uncertain histocompatibility | |
Ethical and legal concerns | ||
Tissue-engineered material | Fabricated from polymers or biomacromolecules | Degradable biomaterials are expensive |
Unlimited source materials | Antigenicity | |
Easy to produce | Exhibit poor tenacity, making them difficult to suture to the injured nerve | |
No donor site trauma |
7.2 Peripheral Nerve Grafts
Autografts
In patients with larger nerve gaps where the injury must be bridged, use of an autograft remains the most reliable repair technique [3, 5]. Nerve autografts have been studied extensively, and their superiority over epineurial suturing under tension has been reported [6]. By using nerve autografts, the surgeons prepare a structural guidance of the natural material for axonal progression from the proximal to the distal nerve stumps. Donor sites for autograft nerve tissue are represented by functionally less important nerves like superficial cutaneous nerve, posterior interosseous nerve, sural nerves, or medial and lateral antebrachial cutaneous nerves [6, 7].
The three major types of autografts are trunk grafts, cable grafts, and vascularized nerve grafts [3]. Trunk grafts are mixed motor and sensory grafts. Trunk grafts have poor functional results due to their instability and large diameters which inhibits its ability to properly revascularize the center of the graft. Cable grafts are several sections of small nerve grafts aligned in parallel to connect fascicular groups. Vascularized nerve grafts have the advantage that there is no period of ischemia compared to nonvascularized grafts and the necessity for revascularization is avoided [3]. Sensory donor nerves are most often used, with the sural nerve being the most commonly harvested (Fig. 7.1a, b). The choice of autograft is dependent on several factors, that is, the size of the nerve gap, location of proposed nerve repair, and associated donor-site morbidity [8]. Use of autografts is currently restricted to critical nerve gaps of nearly 5 cm length [6].


Fig. 7.1
(a, b) Sensory donor nerves are most often used, with the sural nerve being the most commonly harvested. (a, b) A minimally invasive technique of sural nerve harvesting is begun through a small incision at the level of the lateral malleolus, thereby identifying the nerve and inserting the nerve-harvesting device. An additional small incision, if needed, is placed at the junction of the middle and distal thirds of the lower leg, a landmark at which an anastomosis occurs between the medial and lateral sural cutaneous nerves
The main limitations in the use of nerve autografts are considered to be mismatch of donor nerve size and fascicular inconsistency between the autograft and the distal/proximal stumps of the recipient nerve. Because a mismatch in axonal size and alignment further limits the regeneration capacity of the autografts, the type of nerve autografts chosen, like motor nerves, sensory nerves, or mixed nerves, is also decisive for a successful outcome [6]. A prolonged surgical time together with the potential risk of infection and formation of painful neuroma represents other important drawbacks of nerve autografting [6]. Altogether, the recovery time for the patient can be prolonged, owing to the need for a second surgery. The limitations of autografts forced researchers to develop alternative manufacturing approaches for novel nerve conduits for peripheral nerve repair [6].
Allografts
Nerve allografts have a history that exceeds that of autografts. In 1885, Eduard Albert reported the use of a nerve allograft from an amputated limb to bridge a 3 cm median nerve gap arising from resection of a sarcoma [9]. The use of donor-related or cadaveric nerve allografts is reserved for devastating or segmental nerve injuries. Like all tissue allotransplantation, nerve allografts require systemic immunosuppression; the associated morbidity of immunomodulatory therapy limits the widespread application of nerve allografting. Several techniques (e.g., irradiation, cold preservation, lyophilization) to reduce nerve allograft antigenicity have been published [10].
Nerve allografts have demonstrated clinical utility in repairing extensive peripheral nerve injuries where there is a paucity of donor nerve material. Allografts used in peripheral nerve injuries are commercially processed to be cell- and protein-free [3]. This allows the nerve allograft to serve as a scaffold that is repopulated by Schwann cells and host axons over time [3]. The use of allografts presents limitations including especially risk of cross contamination, immune rejection, secondary infection, and limited supply [6]. Therefore, the use of allografts requires systemic immunosuppressive therapy, but long-term immune suppression is not a desirable treatment due to increased risk of infection and decrease of healing rate, and it occasionally results in tumor formation and other systemic effects [6]. In order to overcome some of these limitations, nerve allografts can be processed by repeated irradiation, freeze–thaw cycles, and decellularization with detergents [11].
7.3 Allogeneic Decellularized Nerve Transplantation
Since 2007, decellularized nerve grafts are in clinical use. Since 2013, this alternative is also available in German-speaking countries; however, only a few clinics in Germany gathered experience in this field (Fig. 7.2).




Fig. 7.2
Allogeneic decellularized nerve transplantation. (a) Surgical preparation of the median nerve. (b) Bridging the nerve gap with a decellularized allogeneic nerve. (c) Suturing the decellularized qallogeneic nerve with the median nerve
The allogeneic transplants, which are generated from human donor nerve, combine many advantages due to its macrostructure and the three-dimensional microstructure. First clinical observations indicated in broken sensitive nerves good results to a defect distance of 3 cm. In the ten cases described, the 2-point discrimination (2PD) was 6 mm or better.
The largest prospective study on the use of allogeneic nerve grafts was published in 2011 by Brooks et al. under the name “RANGER study.” The RANGER study registry was initiated in 2007 to study the use of processed nerve allografts (AxoGen® nerve allograft (AxoGen Inc., Alachua, FL)) in contemporary clinical practice [12]. Twelve sites with 25 surgeons contributed data from 132 individual nerve injuries. Data was analyzed to determine the safety and efficacy of the nerve allograft. Sufficient data for efficacy analysis were reported in 76 injuries (49 sensory, 18 mixed, and 9 motor nerves). The mean age was 41 ± 17 (18–86) years. The mean graft length was 22 ± 11 (5–50) mm. Subgroup analysis was performed to determine the relationship to factors known to influence outcomes of nerve repair such as nerve type, gap length, patient age, time to repair, age of injury, and mechanism of injury. Meaningful recovery was reported in 87% of the repairs reporting quantitative data. No graft-related adverse experiences were reported, and a 5% revision rate was observed. Processed nerve allografts performed well and were found to be safe and effective in sensory, mixed, and motor nerve defects between 5 and 50 mm. The outcomes for safety and meaningful recovery observed in this study compare favorably to those reported in the literature for nerve autograft and are higher than those reported for nerve conduits.
Xenografts
A nerve xenograft is obtained from a member of a species other than that of the recipient. A research group developed an experimental animal model to study the potential transplantation of nerve xenografts using the newer immunosuppressive agents RS-61443 and FK-506. They transplanted 2 cm sciatic nerve xenografts obtained from golden Syrian hamsters into a 0.5 cm gap in the sciatic nerve of Lewis rats. The functional recovery in the test animals was found to be not as good as those in the control autografts [13].
Another research team used acellular nerve xenografts and seeded them with bone marrow stromal cells [14]. When the allograft and the xenograft were compared with electrophysiological studies, it was observed that the xenografts were as effective as the allografts in regenerating the nerves. Allograft and xenografts have certain disadvantages such as disease transmission and immunogenicity.
7.4 Nerve Conduits
7.4.1 Biological Nerve Conduits
The use of a conduit as a vehicle for moderation and modulation of the cellular and molecular ambience for nerve regeneration has been widely investigated [15].
A combination of physical, biological, and chemical factors has made the study of nerve tubes a complex process, rising tremendous interest in the fields of medicine. The ideal tubular material has not yet been established. Several materials, either of biologic origin or synthetically fabricated, have been applied for these purposes. The ideal conduit would be made of a low-cost, biologically inert material that is biocompatible; flexible; thin; transparent; inhibitor of inflammatory processes such fibrosis, neuromas, gliomas, swelling, ischemia, and adhesions; and facilitator of the processes that contribute to regeneration, accumulating factors that promote nerve growth [15]. Biological conduits such as autologous veins, arteries, muscle, and heterogeneous collagen tubes denatured skeletal muscle or muscle basal lamina, veins, and polyglycolic acid (PGA)–collagen tubes [16]. Biomaterials such as artery, vein, and muscle have been widely used to repair relatively short nerve defects. These materials can provide support for the nerve in the short term and degrade to innocuous products after complete nerve regeneration.
Table 7.3 summarizes the types and the performance of a variety of conduit materials.
Table 7.3
Design criteria for nerve guidance conduits
Ideal properties | Description |
---|---|
Biocompatibility | Material should not harm the surrounding tissues |
Protein modification/release | Laminin/fibronectin coating for increased cellular adhesion; controlled/sustained growth factor release |
Degradation/porosity | Degradation rate should complement nerve regeneration rate; conduit should allow nutrient diffusion and limit scar tissue infiltration |
Anisotropy | An internal scaffold or film should provide directional guidance |
Physical fit | Conduit should have a large enough internal diameter to not “squeeze” the regenerating nerve; wall thickness limited |
Electrically conducting | Capable of propagating electrical signals |
Support cells | Schwann cells/stem cells capable of delivering neurotrophic factors to the site of regeneration |
In the 1980s, preclinical research by Glasby and colleagues demonstrated that autografts of skeletal muscle which had been deeply frozen in liquid nitrogen and subsequently thawed can provide a valuable matrix for the regenerating nerve, when oriented coaxially with respect to the nerve tissue. In eight patients, this type of grafts was used to repair injured digital nerves. Assessment from 3 to 11 months after operation showed recovery to MRC (Medical Research Council) sensory category S3+ in all patients [17]. Lundborg reported about different methods of frozen muscle grafts and other conduits bridging nerve gaps [18].
There are several advantages, however, in using vein conduits for nerve reconstruction [19]. The tissue composition of veins is similar to that of nerve tissue. Furthermore, muscle–vein-combined graft conduits have been broadly devised and effectively employed for repair of segmental nerve injuries [20]. Manoli et al. conducted a retrospective clinical trial in order to compare regeneration results after digital nerve reconstruction with muscle-in-vein conduits, nerve autografts, or direct suture [21]. In a total of 46 patients with 53 digital nerve injuries with a segmental nerve injury ranging between 1 and 6 cm, no statistically significant differences between all three groups could be found. The authors also emphasized that after harvesting a nerve graft, reduction of sensibility at the donor site occurred in 10 of 14 cases but only in one case after harvesting a muscle-in-vein conduit.
7.4.2 Synthetic Nerve Conduits
They include nondegradable and degradable nerve conduits (Fig. 7.3). Synthetic polymers, though often less biocompatible relative to biopolymers, offer opportunities for tailored degradation and control of mechanical strength, porosity, and microstructure properties [22].


Fig. 7.3
Examples for nerve conduit designs. (a) Acellular nerve repair material and its application for the repair of 2 cm ulnar nerve defect in the fourth finger. (b) Acellular nerve repair material and its application for the repair of 2 cm radial nerve defect in the thumb
In the meantime, the material choice for nerve conduits shifted toward the use of more biocompatible synthetic polymers. Biodegradable polyesters, such as polyglycolic acid (PGA), polylactic acid (PLA), poly(ε-caprolactone) (PCL), poly(lactic acid-co-glycolic acid) (PLGA), polyurethanes (PUs), and nonbiodegradable polymers such as methacrylate-based hydrogels, silicone, polystyrene, and polytetrafluoroethylene), were used as nerve conduit materials and intensively studied in preclinical models [6].
7.4.3 Technique of Tubulization (Fig. 7.4)
Surgery on the peripheral nerve requires microsurgical techniques. Following debridement and neurolysis if applicable, the nerve stumps are located with one or two u-sutures of 8/0 to 10/0 nylon and inserted into the moistened tube with an overlap of 2–3 mm (Fig. 7.4a, c).


Fig. 7.4
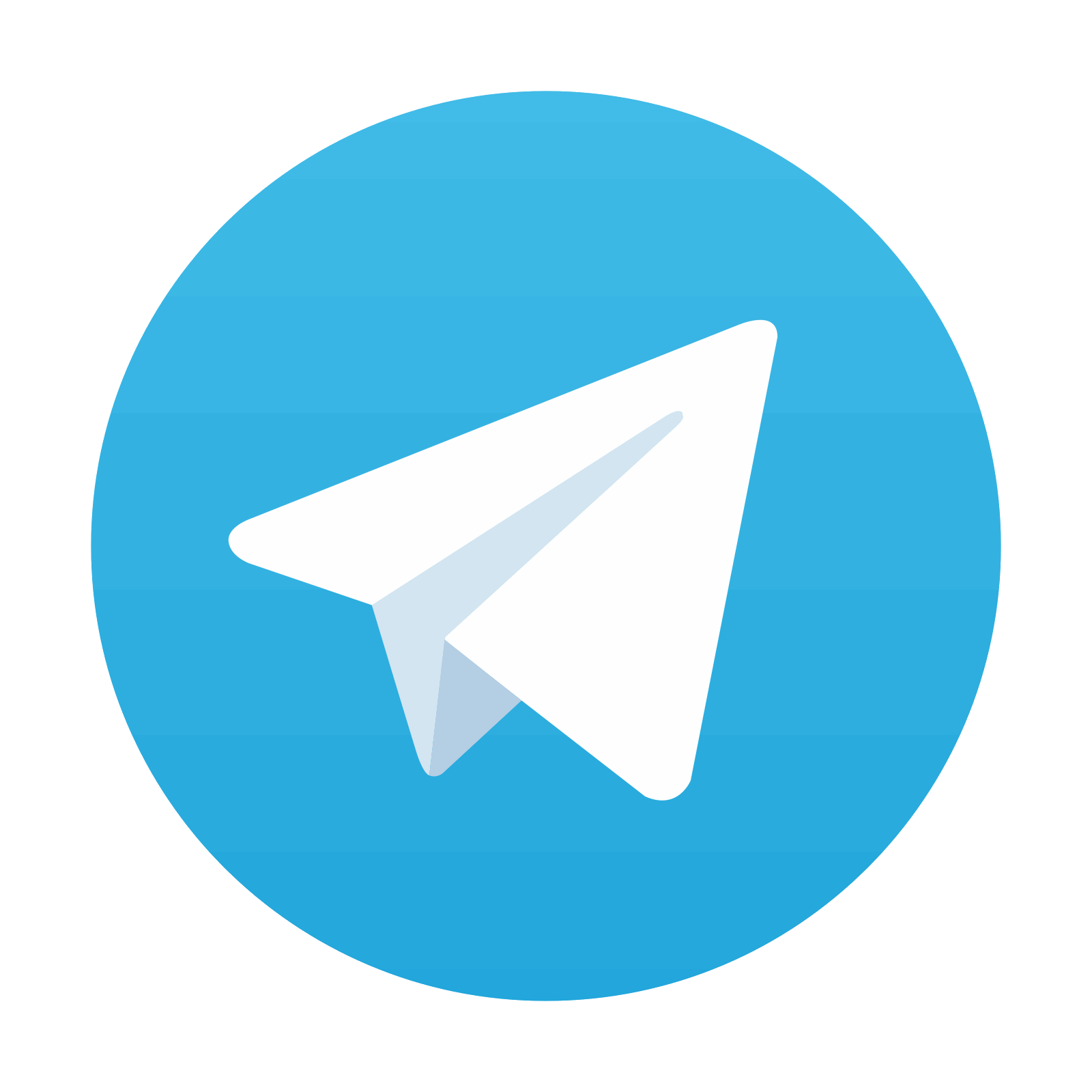
Schematic representation of the tubulization technique. (a–c) Nerve stumps are located with one or two u-sutures of nylon and inserted into the moistened tube with an overlap of 2–3 mm. (b, d) After finishing each coaptation, the lumen has to be rinsed with normal saline or electrolyte solution using a small cannula to remove any remaining blood clots
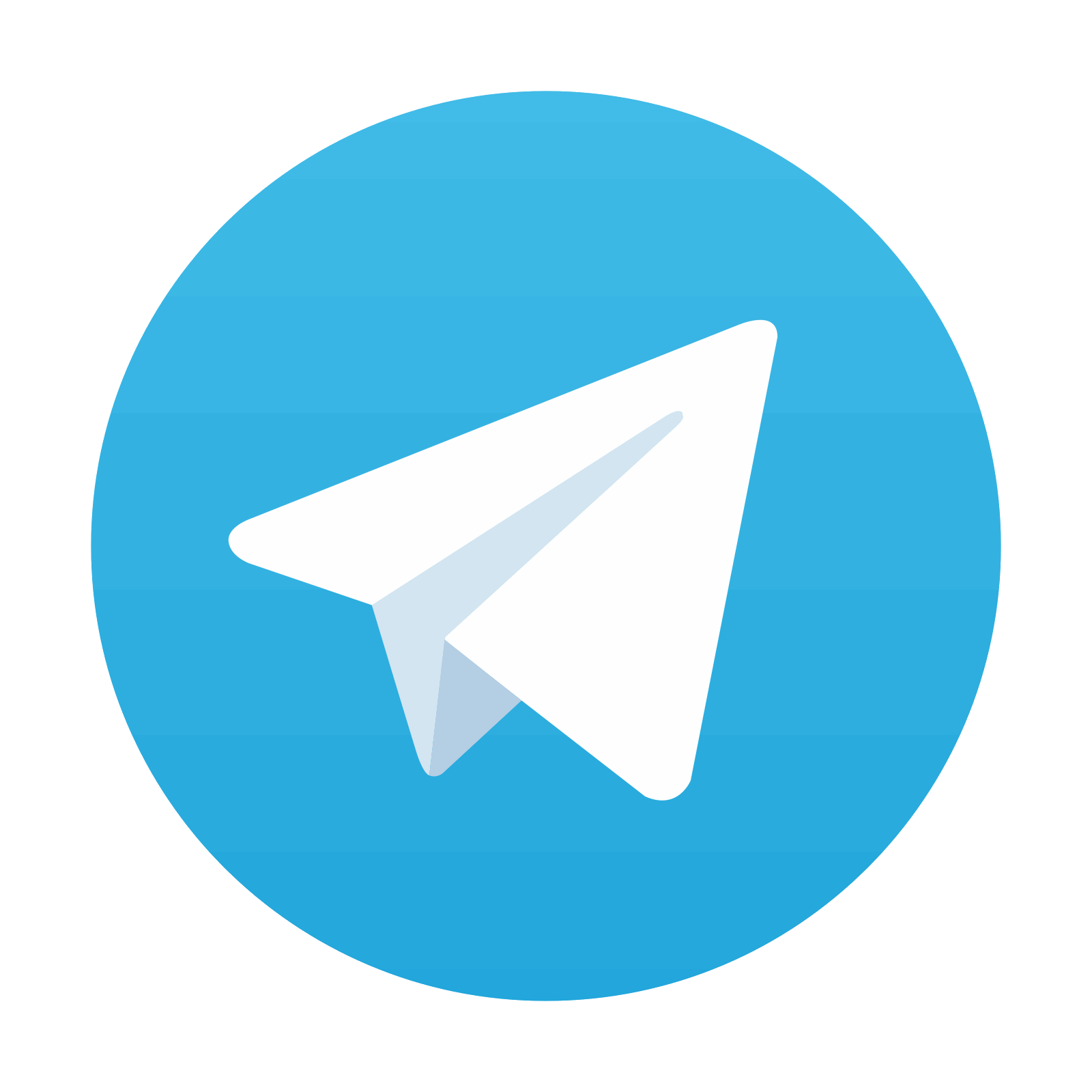
Stay updated, free articles. Join our Telegram channel

Full access? Get Clinical Tree
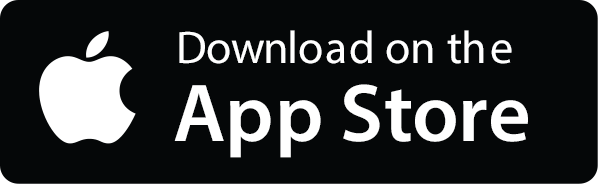
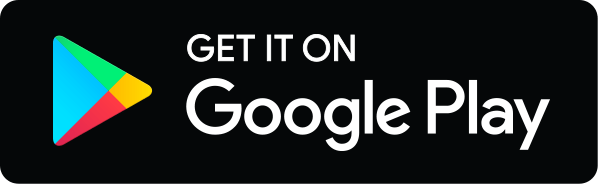
