Categorize the somatosensory receptors.
Differentiate between phasic and tonic receptors.
Describe the sensory modalities associated with somatosensory system.
Describe the relationship between the receptive field and receptor density.
Differentiate between encapsulated and nonencapsulated receptors.
Compare the types of sensory receptors to rate of conduction.
Overview of Anatomical Receptors
The body’s ability to adapt to its environment and maintain homeostasis depends on the transmission of sensory input by the peripheral nervous system (PNS). The detection of sensory input occurs through specialized receptive nerve endings, known as sensory receptors, found at the terminal ends of afferent (sensory) peripheral nerve fibers. Based on the type of stimulus to which the receptor responds, sensory receptors may be broadly classified as nociceptors, thermoreceptors, chemoreceptors, photoreceptors, and mechanoreceptors. Each type of receptor plays an integral role in collecting sensory input from the external and internal environment and transmitting the information to the central nervous system (CNS) for conscious and unconscious processing. Sensory receptors that detect sensations of pain, temperature, discriminative touch, pressure, visceral distention, and proprioception are known as somatosensory receptors and are broadly distributed within the skin, muscles, joint capsules, and viscera. In comparison, sensory receptors that detect special sensory modalities such as light, smell, taste, and sound are modified neurons or specialized epithelial receptor cells, which exhibit restricted expression to the olfactory epithelium, the retina of the eye, the taste buds of the oral cavity, and the vestibulocochlear apparatus.
This chapter introduces somatosensory stimuli and the types of cutaneous, muscle, joint, and visceral receptors that are responsible for detecting a perceived sensation. Transmission of different somatosensory stimuli follows distinct anatomical sensory pathways to the cerebral cortex and cerebellum. The ascending somatosensory pathways of the body and the head are described in Chapters 12 and 15, respectively. The special sensory pathways associated with vision, hearing, balance, smell, and taste are described in Chapter 15.
General Properties of Somatosensory Receptors
The detection of somatic and visceral input from the environment occurs through specialized receptive nerve endings known as somatosensory receptors, which are formed by the peripheral axon of primary (afferent) sensory neurons ().
The sensory neuron cell body for all somatosensory and visceral receptors is a pseudounipolar neuron located in the dorsal root sensory ganglia or the cranial sensory ganglia associated with CNs V, VII, IX, and X.
Pseudounipolar neurons possess only a single process that emerges from the ganglion cell body and branches into a central process and a peripheral process. A synapse does not occur in the sensory ganglion, and the information detected in the periphery passes to the CNS without interruption.
The central process of a pseudounipolar sensory neuron transmits the impulse toward the CNS. The central process of the sensory neuron enters the dorsal root of the spinal nerve or the sensory root of the cranial nerve, and passes to the spinal cord or brainstem, respectively.
The peripheral axonal process transmits sensory input from the periphery toward the ganglion cell body. The peripheral axon of sensory neurons follows the spinal or cranial nerve to the periphery and terminates as a specific somatosensory receptor that responds to a specific type of stimulus.
As discussed in Chapter 3, peripheral afferent nerve fibers may be classified based on axonal diameter and transmission speed as Type I (Aα), Type II (Aβ), Type III (Aδ), and Type IV (C) fibers.
Type I, II, and III fibers represent myelinated axons of varying diameters. Unmyelinated afferent fibers that exhibit the smallest axonal diameter comprise group IV. The difference in diameter, myelination, and conduction velocity impacts the speed of sensory transmission and central processing.
Individual primary afferent sensory neurons respond to specific stimuli based on the properties of the receptor and the type of nerve fiber transmitting the sensory information.
Morphological variations, such as axonal diameter, the extent of myelination, receptor location, and the presence of a connective tissue sheath surrounding the receptor nerve ending (encapsulation), contribute to the functional properties of different somatosensory receptors and serves as the basis for sensory receptor classification.
Fig. 11.1 Schematic demonstrating the location of cutaneous and visceral somatosensory receptors and the location of the corresponding primary afferent sensory cell body in the dorsal root ganglia. A simplified path of transmission from the periphery to the spinal cord is shown. Cutaneous receptors convey tactile (mechanosensory), nociceptive, and thermal input from the skin to the CNS. Visceral receptors convey mechanical stretch and nociceptive input from the wall of the viscera to the CNS. The neurons for both types of afferent receptors reside in the dorsal root ganglion (shown) or in some cranial sensory ganglia. Cutaneous sensation follows the spinal nerves while visceral sensory input travels initially from the viscera along autonomic pathways before entering the dorsal horn of the spinal cord. CNS, central nervous system. (Modified with permission from TannerThies R. Physiology: An Illustrated Review. © Thieme 2012.)
Sensory Reception and Transduction
The process by which receptor stimulation leads to a perceived sensation is similar for all types of sensory receptors ().
Receptor activation by a specific environmental stimulus initiates sensory reception and leads to the conversion of the environmental stimulus into an electrical signal (generator potential) through the process of sensory transduction. If the generator potential is large enough to exceed threshold an action potential will be generated.
Primary sensory afferent fibers of pseudounipolar neurons transmit an action potential along a specific somatosensory pathway to precise areas in somatosensory cortex of CNS for processing and interpretation.
The transmission and efficient processing of somatosensory input require that several attributes about receptor activation and transduction be encoded as part of the process. The stimulus components encoded at the time of sensory reception and stimulus transduction includes the following:
Stimulus intensity threshold: Refers to the minimal amount or intensity required by the receptor to generate an action potential. This threshold is referred to as an adequate stimulus.
Stimulus location or receptive field: Refers to the specific location where the receptor of the sensory neuron elicits a response when stimulated. The ability to detect the precise location of a stimulus depends on the size of the receptive field.
Stimulus duration or adaption: Refers to the rate at which the receptor adapts to the stimulus and the action potential dissipates.
Stimulus (sensory) modality: Refers to the type of sensation produced in response to activation of a receptor and its sensory neuron by a specific type of stimulus.
The following section describes each of the encoded sensory attributes in more detail.
Fig. 11.2 Schematic flowchart demonstrating the four steps of somatosensory processing. Efficient sensory reception, transmission, and perception depend on encoding information about the type of stimulus, the location of the receptive field, the duration of the signal, and the relative intensity of the stimulus. Reception is the first step in the processing of sensation. Sensory input in the form of a stimulus leads to activation of receptors and the conversion of the stimulus into a receptor (generator) potential. A specific receptor will preferentially respond to a specific type of stimulus at a certain threshold. The higher the receptor threshold, the less sensitive the receptor will be to the stimulus. Receptor activation leads to a receptor potential that is graded continuously and dependent on the strength of the stimulus. When the receptor potential exceeds the receptor’s signaling threshold, an action potential results. In neurons, the stimulus intensity is coded by the frequency of the action potential so that a stronger stimulus directly correlates with a faster firing rate. The specific area of tissue that responds to the stimulus, and produces a response, corresponds to the sensory neuron’s receptive field. The size of the receptive field impacts the accuracy in the ability to precisely detect the location of the stimulus. A smaller receptive field leads to more accuracy in detecting the location of the stimulus. The duration of receptor activation corresponds to the length of time the receptor continues to fire with continued stimulation and depends on the adaptive characteristics of the receptor. Based on the type of stimulus, the transmission of the impulse follows specific sensory pathways to distinct areas in the contralateral (opposite side) cortex, where the stimulus is processed and consciously perceived as a specific sensation, or sensory modality. Sensations are mapped directly from receptors to specific regions of the cortex to give a direct association between the stimulus and the sensory neuron receptor. Perception is the individual’s interpretation of the sensation and may vary among individuals due to modulatory effects and cognitive input.
Adequate Stimulus
Each receptor preferentially responds to a specific stimulus and will elicit a response at a specific level or threshold.
The minimal stimulus or lowest threshold necessary to generate an action potential is referred to as the adequate stimulus and represents the type of stimulus to which the receptor is most sensitive.
The type of adequate stimulus for a receptor correlates with the sensory modality encoded by the receptor.
Stimuli such as mechanical deformation, tissue damage, temperature changes, or chemical changes in the environment are adequate to elicit a sensory response and serve as the basis for receptor classification.
Types of somatosensory receptors include mechanoreceptors, nociceptors, thermoreceptors, and chemoreceptors.
The threshold or intensity of the stimulus necessary to generate an action potential varies for different types of receptors and is known as the receptor threshold.
Information concerning the stimulus intensity is conveyed to the CNS as the frequency of receptor firing and the number of receptors recruited. An intense stimulus causes a rapid firing rate of the receptor, and in some cases, may also initiate action potentials in several adjacent receptors.
Receptor threshold and intensity serve as a mechanism for further classification. Low threshold (high sensitivity) receptors require only a small or weak stimulus, whereas high threshold (low sensitivity) receptors need intense stimuli to respond.
Receptive Fields
The ability to discriminate between different points of receptor stimulation is an essential attribute of sensory feedback that is influenced by the location of the receptor, the receptor density of the area, and the size of the receptive field ().
The receptive field of a sensory neuron represents the area of tissue that, when stimulated, leads to receptor activation in the region and elicits a characteristic sensation. Therefore, the receptive field provides information about the location of the stimulus and the sensory modality.
The neurons associated with the receptors of a specific receptive field are associated with a specific sensory modality and transmit sensory input along particular ascending sensory pathways to terminate in specific regions in somatosensory cortex.
The size of the receptive field correlates with the receptor density, the level of receptor sensitivity, and the ability to precisely localize the response. A small receptive field allows more precise localization of stimulus.
The location of the receptor correlates with receptor field size and receptor density.
Cutaneous receptors that are superficially located exhibit a higher distribution density within a specific area and a more localized or discriminative sensation.
In comparison, receptors found in deeper tissue regions such as muscle tissue and joints are widely dispersed, exhibit a broader receptive field, and a less localized sensation ().
The size of the receptive field and the receptor density vary between different regions of the body and reflect the functional requirements of the region.
Areas of the body which exhibit an increase in receptor density and small receptive fields include the face, lips, tongue, oral mucosa, fingertips, and hands. Functionally these areas perform numerous tasks that require a high level of discriminative tactile and proprioceptive input.
Fig. 11.3 Schematic diagram illustrating the difference in the receptive field size supplied by different cortical modules in the upper limb of a primate. Individual receptive fields are depicted by individual red circles with the receptive field size corresponding to the size of the circle. Each receptive field is associated with one cortical module. The receptive field of a sensory neuron represents the area of the body that, when stimulated, leads to receptor activation, and produces a characteristic sensation. Information from sensory receptors in a specific receptive field follows a three-neuron relay chain to terminate in specific regions of the somatosensory cortex for processing. Based on the type of sensory input and location of the receptive field, specific cortical neurons that are arranged in functional columns (cortical modules) receive the information. Due to the topographical organization of the cortex, receptive fields in proximity of the body terminate in adjoining areas of the cortex. The size of the receptive field correlates with the functional requirements of specific regions of the body. Small receptive fields correspond to areas in the body, such as the fingertips, which require fine tactile discrimination and high resolution. One cortical module receives input from a small receptive field. Small receptive fields provide greater acuity and a more localized response. In areas that do not require high specificity, one cortical module supplies a large receptive field. Larger receptive fields such as those depicted in the palm (metacarpal) and forearm region allow for the detection of stimuli over a wider area, but the ability to precisely localize the stimulus is diminished. Because one skin area may be innervated by several neurons, many of the receptive fields overlap. (Reproduced with permission from Schuenke M, Schulte E, Schumacher U. THIEME Atlas of Anatomy Second Edition, Vol 3. ©Thieme 2016. Illustrations by Markus Voll and Karl Wesker.)
Fig. 11.4 Schematic diagram of cutaneous tactile receptor density, location, and corresponding receptive field size in the skin of the fingertips. Touch receptors vary in density between different areas of the body and within different tissue layers. The four major types of cutaneous (tactile) mechanoreceptors, which include the Merkel’s cell, Meissner’s corpuscle, Ruffini’s corpuscle, and Pacinian corpuscle, are differentially distributed between the superficial and deep layers of the skin. The superficially located Merkel’s cell and Meissner’s corpuscle exhibit higher receptor density and smaller receptive fields in the fingertips when compared to the Pacinian and Ruffini’s corpuscles. The Pacinian and Ruffini’s corpuscles also reside in the fingertip but are found in the deep layers of the dermis and hypodermis. These receptors exhibit wider receptive fields and less density. The ability to accurately discern the precise location of a stimulus correlates with the receptor density, receptive field size, as well as the location and function of the receptor. For regions such as the fingertips, which require high resolution and tactile discrimination, the receptive fields are smaller and contain a higher number of superficial receptors that function in fine discriminative touch. The receptors found in deeper regions convey different mechanosensory inputs such as stretch, vibration, and transient pressure, and therefore, exhibit slightly larger receptive fields with fewer receptors scattered throughout the receptive field. The localization of the sensation is less precise and more diffuse.
Sensory Adaptation
Most receptors may adjust to prolonged stimulation by decreasing the rate at which it generates an action potential. The process by which a receptor becomes less sensitive to a continued stimulus is known as sensory adaptation and plays an important role in the ability of the sensory system to monitor, adjust, and react to continual changes in the environment.
The rate of receptor adaption reflects the duration of how long the receptor encodes the stimulus. This property varies between different types of receptors and allows receptors to be classified as phasic or tonic receptors. Rapid adapting receptors, or phasic receptors, respond quickly to stimuli; however, the sensation rapidly dissipates as the ability of the receptor to generate an action potential diminishes.
Rapidly adapting receptors detect moving or vibrating stimuli and provide information about changes in the stimuli intensity. Rapidly adapting receptors include types of tactile and proprioceptive mechanoreceptors found in skin, muscles, and joints. Several receptors involved in detecting visceral sensation also function as phasic or rapidly adapting receptors.
Slowly adapting or tonic receptors provide input about the continued presence and intensity of the stimulus by sustaining an action potential for the duration of the stimulus.
Slow adapting receptors respond to static stimuli, such as constant pressure, and include types of tactile mechanoreceptors, some proprioceptive mechanoreceptors, as well as some nociceptors and visceral receptors.
Most tissues, including those in the oral cavity, contain both rapid and slow adapting receptors. The presence of both receptor types alters the rate at which the tissue adapts and responds to sensory input and is an important mechanism to detect changes in the environment and prevent sensory overload.
Stimulus (Sensory) Modalities
In the body and head, the cortical processing of sensory input arising from receptors in the skin, muscle, connective tissue, joints, and viscera leads to the perception of somatic sensations or sensory modalities.
The modalities of somatic sensations include touch, proprioception, pain, and temperature. Each modality represents a characteristic sensation of a specific stimuli and correlates with a specific type of receptor, its associated afferent fiber, and primary afferent neuron ().
Sensory modalities may be separated into submodalities which further describes the quality of the perceived sensation. Submodalities of touch, proprioception, thermal sensations, and pain are described below and correspond to the stimulus that preferentially activates the receptor.
Touch or tactile sensation refers to the awareness and ability to localize the point of mechanical stimulation. Submodalities of tactile sensations include crude (light) touch, fine discriminative touch, pressure, vibration, and stretch.
Activation of tactile receptors occurs through the mechanical displacement of the receptor. The different submodalities of tactile sensation reflect different levels of distortion of the skin and correlate with the stimulation of different types of tactile receptors.
Crude and discriminative touch: Tactile responses may be described as crude and nondiscriminative touch or as fine, discriminative touch. This difference correlates with the type of receptor and sensory neuron, the receptor location, density, and receptive field size.
Crude touch receptors only convey the sense of touch and not the location of the stimulus. Crude touch receptors are found throughout the body, including the cornea, dental pulp, mucosa, and viscera.
In comparison, fine, discriminative tactile receptors enable the ability to distinguish the location of an applied stimulus to the skin by one or two points. This type of fine, discriminative touch is also known as two-point discrimination. The minimal distance that may be detected separately varies between different parts of the body. Areas of the body that functionally require precise tactile discrimination exhibit high receptor density, smaller receptive fields, and smaller distances between two points.
Fine, discriminative tactile receptors also function to discern the shape, size, and texture of an object by means of touch in the absence of visual input through a process known as stereognosis. The ability to perform stereognosis depends on both intact tactile sensory pathways and higher cortical input involving memory of the object.
Disruption in discriminative sensory feedback in the regions of the fingertips, oral cavity, hands, and tongue leads to difficulties in coordinating motor responses and an inability to recognize the size, shape, and texture of an object without visual input (astereognosis).
Vibration: Vibratory sense refers to the tactile sensation of a mechanical vibratory movement occurring in the skin, viscera, muscle, joints, or the periosteum of bone. Specific tactile receptors respond to either low- or high-frequency vibrations generated by mechanical stimulation. Low-frequency vibrations produce the sensation of flutter, stroking, or movement, while high-frequency vibrations create the sense of vibration and deep pressure.
Pressure: Tactile pressure, or deep touch sensations, refers to the perception of a maintained distortion, such as an indentation, or a compressive force applied to the skin, muscle, or viscera. The sensations of deep touch and vibration are important in mediating stability, balance, and bodily movements associated with proprioception.
Proprioception refers to the awareness of movement and position of the body, limbs, and joints, along with the sensation of muscle force and tension. The sense of position and movement arises from the mechanical displacement of specific receptors found in tendons, joints, capsules, and muscles.
Thermal sensations range from hot to warm, or cool to cold. The ability to detect temperature changes is important for homeostatic thermoregulation and as a protective response to excessive temperatures that may cause tissue damage. Each temperature range has a threshold that will trigger the activation of a receptor known as a thermoreceptor.
Pain is a subjective sensation often associated with tissue injury that may involve both physical and emotional discomfort or agony. Different types of receptors, known as nociceptors, respond to noxious stimuli and generate two types of nociceptive pain sensations. Submodalities of nociceptive pain include fast pain and slow pain.
Fast pain, also known as first onset (first) pain, is associated with a well-localized, sharp, pricking, stinging, or stabbing sensation that is of short duration. This type of pain often occurs from mechanical stimuli such as cutting, pinching, or pinpricks, but may also be associated with insect bites, or thermal stimuli such as intense heat. First pain is transmitted rapidly through lightly myelinated nerve fibers to the cortex for conscious processing.
Slow pain, or second pain, is a dull, burning, or aching sensation that is diffuse, poorly localized, and of longer duration. Burning pain is generally caused by inflammation, while aching pain arises from the viscera, or deep subcutaneous structures such as bone, muscles, joints, and connective tissue. Mechanical, thermal, and chemical stimuli associated with swelling, tearing, rupture, ischemia, or inflammation may elicit slow pain. The transmission of second pain occurs slowly through small, unmyelinated nerve fibers. The fibers follow specific tracts that typically terminate diffusely in regions other than the somatosensory cortex.
Somatosensory Receptor Classification
Somatosensory receptors may be classified based on function, structure, and location.
Functional Classification
The type of adequate stimuli to which a receptor responds and elicits a characteristic sensation serves as the basis for receptor classification. Somatosensory receptors may be classified into four functional types: mechanoreceptors, thermoreceptors, nociceptors, and chemoreceptors (see ).
Mechanoreceptors: Respond to mechanical deformation of the skin, muscles, tendons, joint capsules, and viscera. Myelinated and unmyelinated afferent nerve fibers of mechanoreceptors terminate to form three classes of mechanoreceptors: tactile mechanoreceptors, visceral mechanoreceptors, and proprioceptors.
A specialized type of visceral mechanoreceptor, known as a baroreceptor, monitors arterial blood pressure and acts as pressure receptor. Baroreceptors exhibit a more restricted expression to the cardiovascular system and reside in the wall of the aorta and carotid artery.
The nerve fibers associated with the detection of innocuous mechanical stimuli from skin, muscle, tendons, and joints are large diameter, heavily myelinated axons known as large diameter, heavily myelinated axons of Type Ia (Aα) fibers and Type II (Aβ) fibers. Both types of axons rapidly transmit mechanoreceptive input from several cutaneous mechanoreceptors and proprioceptors to the CNS for processing.
Mechanoreceptive signals associated with light (crude) touch and pressure detected from the skin, peritoneum (serosa), mucosa, blood vessels, and organs are conveyed by smaller, lightly myelinated Type III (Aδ) and unmyelinated Type IV (C) fibers.
Thermoreceptors respond to changes in temperature occurring at the receptive nerve endings found throughout the body. Thermoreceptors, which are important in the homeostatic maintenance of our internal body temperature, are sensitive to warm, hot, cool, and cold stimuli. Thermoreceptors rapidly adapt to temperature changes that vary from the normal skin temperature of 34–36 °C (93–98 °F). Thermal changes within a certain range may be classified as innocuous, while temperature changes above or below those levels are considered noxious stimuli.
Warm (innocuous) receptors respond to temperatures between 25 °C (77 °F) and 45 °C (113 °F) with maximal stimulation occurring 10 °C above normal skin temperatures. Temperatures above 45 °C (113 °F) are often perceived as pain (heat) due to the activation of a type of noxious high threshold (hot) receptors known as a thermo-nociceptor.
Cold (innocuous) receptors are more numerous than heat receptors and normally respond to decreases in temperature below the normal skin temperature range of 34 to 36 °C (93–98 °F). Maximal firing occurs about 10 °C below normal skin temperature of 25 °C (77 °F), while skin temperatures below 15 °C (59 °F) stimulate noxious cold receptors. When these thermo-nociceptors become activated, the sensation is one of pain.
Thermoreceptors are the free nerve endings of lightly myelinated Type III, (Aδ), or unmyelinated, Type IV (C fibers) nerve fibers. Typically, the lightly myelinated Aδ fibers convey cool temperature sensations and exhibit faster conduction velocities, whereas unmyelinated C fibers transmit warm sensations at slower conduction speeds. As a result, the time for the body to respond is faster for a cold stimulus and slower for a warm stimulus.
Chemoreceptors associated with the somatosensory system rapidly respond to chemicals released in response to changes in pH, oxygen, and carbon dioxide levels and function as part of unconscious visceral reflex pathways. Chemical stimuli associated with inflammatory mediators produced in response to inflammation from tissue damage are detected by polymodal nociceptors and are often perceived as dull, burning pain.
Nociceptor s respond to noxious stimuli characterized as extreme pressure, temperature, and chemical irritants that may cause tissue damage, inflammation, or decreased blood flow (ischemia). Activation of nociceptors occurs in response to intense, high threshold stimuli as part of a protective response against further tissue damage and nociceptors are ubiquitously expressed in most tissue, including the skin, connective tissue, joint capsules, muscles, tendons, viscera and serosa, and the periosteum of the bone.
Nociceptors represent the unencapsulated free nerve ending of either lightly myelinated (Type III, Aδ) fibers or unmyelinated axons (Type IV, C fibers). Noxious stimuli transmitted by lightly myelinated Aδ fibers pass rapidly to the CNS and generate a fast-onset, localized, sharp pain sensation. Unmyelinated C fibers conduct noxious stimuli slowly toward the CNS, resulting in the sensation of slow, burning, or aching pain.
Nociceptors are classified based on their sensitivity into noxious mechanical, thermal, and/or chemical stimuli caused by tissue damage and inflammation. There are four classes of nociceptive receptors.
Mechano sensitive nociceptors represent the receptive nerve endings of nociceptive-specific neurons that respond to extreme pressure, such as cutting or pinching. Lightly myelinated Type III Aδ fibers transmit the information from mechano-sensitive nociceptive nerve endings to the CNS for processing.
Thermo sensitive nociceptors represent high threshold thermal receptors that respond preferentially to intense heat and cold. Lightly myelinated Type III Aδ and unmyelinated Type IV C fibers convey intense heat (above 45 °C) and intense cold (below 15 °C) to the CNS.
Polymodal nociceptors respond to noxious chemical, mechanical, or intense thermal stimuli. The transmission of nociceptive input from polymodal nociceptors occurs through unmyelinated Type IV C fibers and is perceived as diffuse, dull pain. Polymodal C fibers comprise approximately 70% of all fibers carrying nociceptive input.
Mechano insensitive nociceptors, also known as silent nociceptors, represent the last class of nociceptive nerve endings. Mechano insensitive receptors are high threshold receptors that are usually “silent” or unresponsive to mechanical and thermal stimulation. However, in response to tissue inflammation, the mechano insensitive nociceptors become sensitized to the noxious chemical mediators produced during the inflammatory response. Sensitization lowers the activation threshold of the receptor causing it to become stimulated at a lower threshold.
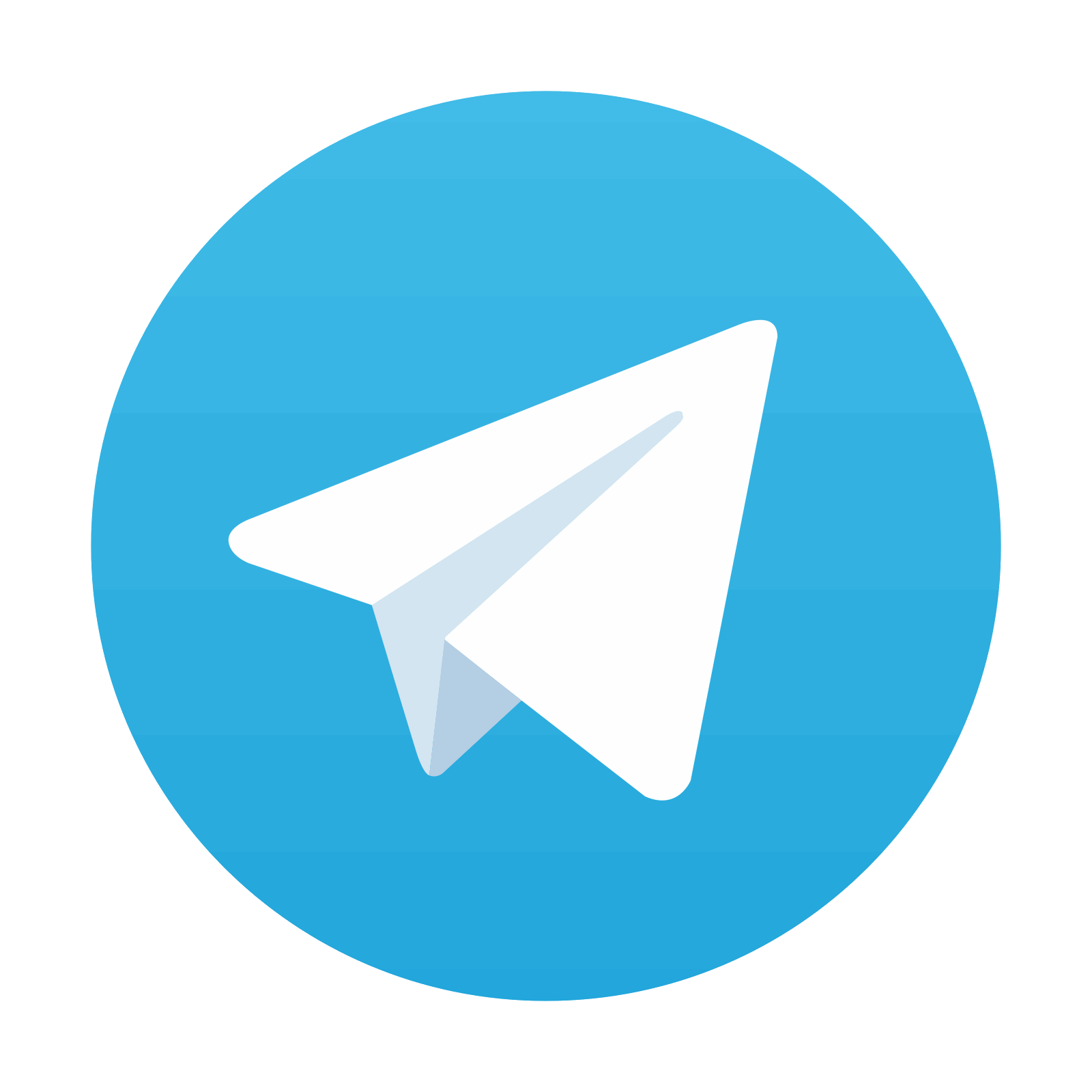
Stay updated, free articles. Join our Telegram channel

Full access? Get Clinical Tree
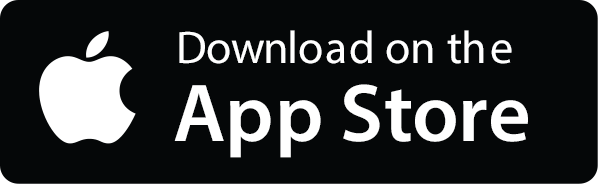
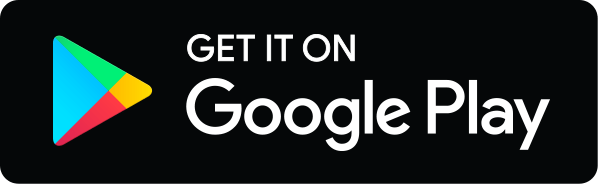
