Chapter 16 Anatomy and Degenerative Diseases of the Spine
ANATOMY
Spinal Nerves
The spinal cord contains 8 cervical, 12 thoracic, 5 lumbar, 5 sacral, and 1 coccygeal pairs of spinal nerves. These nerves are rather easily identified on CT with intrathecal contrast or high-resolution MR. It is important to appreciate that C1, which is a sensory nerve, exits above the C1–C2 interspace so that the C2 nerve exits between C1 and C2, and so on. Thus, in the cervical spine the nerves running through the cervical neural foramina are the higher numbered cervical root (e.g., the C6 root goes through the C5–C6 foramen). At the cervicothoracic junction, the C8 nerve root exits between C7 and T1. Below this level it is the lower-numbered root going through the respective foramina. Thus, T1 exits between T1 and T2, and T12 exits between T12 and L1. In the lumbar spine the L1 root exits between L1 and L2 and so forth, so that the L5 root exits between L5 and S1. However, a funny thing happened on the way to creation. The bodies in the lumbar region became much longer. The nerve roots in this region leave the thecal sac right under the pedicle (Fig. 16-1), well above the interspace. Paracentral disk herniations in the lumbar region characteristically strike the root in the thecal sac that will exit below the interspace. This is because the disk space is inferior to the same numbered exiting root at that level. Thus, an L4–L5 disk herniation most often compresses the L5 root because the L4 root is already in the foramen. Far lateral herniated disks may compress the upper root; that is, an L4–L5 lateral or foraminal herniation can compress the L4 root. Larger disks can compress many roots in the thecal sac. Furthermore, disk fragments may migrate superiorly and compress the root exiting at the appropriate interspace, that is, an L4–L5 free fragment can compress the L4 root or a combination of both the L4 and L5 nerve roots.
Each spinal nerve is divided into a dorsal or sensory root and a ventral or motor root. The dorsal root ganglion is a distal dilatation of the dorsal root just proximal to its joining with the ventral root to form the spinal nerve (Fig. 16-2).
Vertebrae
The generic vertebra is composed of the cylindrical vertebral body, which contains cancellous bone with marrow and fat, covered by a thin layer of compact cortical bone, and the vertebral arch or posterior elements, which include the pedicles, laminae, superior and inferior facets, transverse processes, and spinous process (Fig. 16-3). The vertebral configuration is modified in the different regions of the spine. The cervical vertebrae have their neural foramina between the transverse processes. The superior and inferior articular facets in the cervical region form the articular pillar (Fig. 16-4). The lower five cervical vertebrae have five joints connecting them. They are the intervertebral disk, two facet (zygapophyseal) joints, and two uncovertebral joints. The uncovertebral joints (neurocentral joints, joints of Luschka) originate from the lateral posterior portion of the vertebral body, articulate with the contiguous vertebral body, and insinuate themselves between the disk and the nerve root canal. The vertebral artery branches from the subclavian artery (V1 portion), enters the foramen transversarium at approximately C6, and travels superiorly (V2 portion) before exiting at C1–C2 (V3 portion) and then enters the cranial compartment (V4 portion).
The first cervical vertebra (atlas) has no body but rather just an anterior arch connected to two lateral masses and a posterior arch (Fig. 16-5). On the upper surface of the posterior arch is a groove over which the vertebral artery courses after it leaves the foramen transversarium of C1. The vertebral arteries pass through the posterior atlanto-occipital membrane and course anterosuperiorly upward through the foramen magnum. As it pierces the dura the vertebral artery may be slightly narrowed, and this caliber change can serve as a marker for the beginning of the intradural V4 segment of the vertebral artery. The first spinal nerve exits here as well.
The second cervical vertebra, the axis, is unique, with a superior extension from its body termed the dens (odontoid process) (Fig. 16-6). The dens represents the lost vertebral body of the atlas. The articulation between the atlas and axis is composed of multiple synovial joints—one medial between the dens and the anterior arch, one on each side between the inferior articular facet of the lateral mass of the atlas and the superior facet of the axis, and multiple ones between the dens and the atlantoaxial ligaments (transverse, cruciate, and alar). Rheumatoid arthritis has a propensity for the atlantoaxial joint with pannus formation, leading to bone erosions and subluxations.
The thoracic vertebrae have an articulation on the transverse process for the rib and no foramen transversarium, whereas the lumbar vertebrae have neither a foramen transversarium nor a specific facet for the rib articulation. The lumbar vertebral articulations are composed of the lumbar disk and two facet joints posteriorly. The lateral recess of the lumbar spine is in the anterolateral portion of the spinal canal, with boundaries consisting of the posterior margin of the vertebral body and disk anteriorly, the medial margin of the pedicle laterally, and the superior articular facet, the medial insertion of the ligamentum flavum, the lamina, and the pars interarticularis posteriorly (Fig. 16-7).
Intervertebral Disks
The annulus fibrosus surrounds the nucleus pulposus (the remnant of the notochord). The nucleus is eccentrically located near the posterior surface of the disk. The lamellae of the annulus are fewer in number, thinner, and more closely packed posteriorly than anteriorly. This anatomic arrangement may account for the propensity for posterior disk herniation. The external fibers of the annulus are connected to the bone of the vertebral bodies by Sharpey’s fibers, which usually cannot be distinguished by imaging. Annular fibers also merge with both anterior and posterior longitudinal ligaments. Important ligaments of the vertebral column are (1) the anterior longitudinal ligament, running along the anterior aspect of the vertebral bodies; (2) the posterior longitudinal ligament, running along the posterior aspect of the vertebral bodies anterior to the thecal sac; (3) the ligamentum flavum, connecting the laminae and extending from the midline laterally to the facets; and (4) the interspinous ligament, joining the superior portion of the spinous process below to the inferior part of the spinous process above and meeting the ligamentum flavum in the midline (Fig. 16-8). There are also small ligaments in the neural foramen, which may play a role in foraminal stenosis.
Blood Supply to the Spinal Cord
The blood supply to the spinal cord depends on the particular location (Fig. 16-9). In the cervical region the anterior spinal artery is formed by branches that originate from the vertebral arteries just before joining the basilar artery. The anterior spinal artery supplies the anterior two thirds of the spinal cord. In addition, paired posterior spinal arteries originate from the vertebral arteries and supply the dorsal portion of the cord (one sixth each). These two arterial systems do not usually have significant anastomoses between them. The anterior spinal artery supplies the anterior column of the central gray matter, the corticospinal, spinothalamic, and other tracts. The paired posterior spinal arteries supply the posterior columns and the posterior horn of the central gray matter. The anterior spinal artery is in the midline, whereas the posterior spinal arteries lie off the midline (see Fig. 16-9B). The anterior and posterior spinal arteries rarely originate at the same level. The caliber of the anterior spinal artery at a particular spinal level is proportional to the metabolic demands of the spinal gray matter.
RADIOLOGIC WORKUP
Myelography (see Chapter 1)
“What presently is the role for myelography?” Although not performed as often as it once was, myelography, almost always combined with CT (myelo-CT), is still a sensitive and useful technique for disk herniation and, more importantly, osteophytic impingement on cervical roots. Myelo-CT is excellent in patients with cervical radiculopathy for visualizing small spurs compressing nerve roots or in cases of cord compression (Fig. 16-10). Other roles include detecting root avulsions, CSF leaks, subarachnoid spread of tumor, small tumor implants on nerve roots, and arachnoiditis. The advantage of myelo-CT is the exquisite bone detail superimposed on the subarachnoid contrast. In the opinion of the authors, myelo-CT is more precise than MR at evaluating the effect of cervical spine uncovertebral joint disease on nerve roots. However, if you are the patient, do you want a spinal tap, with the high likelihood of a spinal headache and the possibility, although remote, of infection or other complications? Some patients report that the postmyelogram headache is worse than the backache before or after surgery. That is an advantage of MR, not to mention it is the method of choice for visualizing the spinal cord and soft tissues.
Patients in whom MR is contraindicated, such as persons with metallic implants or cardiac pacemakers, or those who cannot tolerate MR, can be easily examined by myelo-CT for nonintramedullary pathology. Metallic hardware for spine stabilization, such as pedicle screws and anterior metallic plates, degrades MR to a variable extent, worse at higher field strength (Fig. 16-11). Other potential uses are in cases where ambiguity in the diagnosis exists, such as for demonstrating on avulsed nerve roots or for distinguishing osteophytes from dessicated disks. MR is excellent at depicting compression of the cord and, although it cannot detect a functional spinal cord block with respect to intrathecal contrast, there is no potential for patients with a block deteriorating after spinal tap for myelography.
When you perform a myelogram, be very careful that the contrast agent is instilled in the appropriate compartment. Subdural injections of contrast are not uncommon, and myelograms performed with contrast in the subdural compartment can be misleading (Fig. 16-12). As contrast is instilled into the lumbar region, it is critical to visualize the individual nerve roots of the cauda equina. If these are not demonstrated, you are probably dealing with either a subdural injection or severe arachnoiditis. Another clue to a subdural injection is in the lateral projection, where subdural contrast may collect in the posterior aspect of the thecal sac, as opposed to its normal ventral position, in a patient in the prone position. In the thoracic region, the cord and its surrounding space should be apparent. Failure to separate cord density from surrounding contrast material again suggests subdural injection. The subdural space in the spine is continuous withthat of the brain. On CT, you may see contrast along the clivus, because of subdural injections.
Disk herniation on myelography is diagnosed by displacement of the thecal sac or effacement of a root pouch (Fig. 16-13). It is important to evaluate the myelogram in the anteroposterior (AP), oblique, and lateral planes. A double density can be seen on the lateral radiograph in the paramedian herniated disk. The thecal sac at L5–S1 is convex at its lateral margin as it tapers to the sacrum. Concavity in this region should suggest disk herniation even if the roots do not appear to be effaced. You should appreciate that myelography without CT can be normal in cases of far lateral/foraminal disks and may be insensitive at the L5–S1 level when the cul-de-sac ends at or above this interspace.
On AP myelographic films with an extradural lesion in the cervicothoracic zone, the spinal cord may appear to be enlarged (Fig. 16-14). It is most important to view lesions involving the cord in two planes! Many mistakes have been made by just gunning from the hip off the AP film. This is much less of a problem presently because myelography is usually performed in combination with CT.
Computed Tomography
Just a short word concerning CT: Myelo-CT unambiguously reveals extradural bony lesions compressing the subarachnoid space, roots, and spinal cord. CT without intrathecal contrast is adequate for the lumbar region, where natural contrast exists between epidural fat, disk, and bone (Fig. 16-15). However, there is usually little contrast between the spinal cord and the subarachnoid space in the cervical and thoracic regions, so that intradural processes are suboptimally imaged without intrathecal contrast.
Low Tech: Plain Spine Films
Plain spine films are still performed and are useful, particularly when looking for small fractures in cases of trauma and to check alignment of the vertebral bodies, the position of bone grafts, pedicle screws, cages, plates, and abnormal motion of vertebrae during flexion and extension. Every view contains potential aids in demonstrating disease, but we will briefly focus on a few specific regions and important information to be gained. In the AP and oblique views of the cervical spine, the uncovertebral and facet joints and their relationship to the neural foramina are best demonstrated (Fig. 16-16). There is reasonably good correlation between uncovertebral spurs and myelographic impingement associated with radiculopathy. Alignment of the spinous processes should be assessed for rotational injury to the spine. Subluxations caused by trauma can be detected by noting differences in distance between the spinous process tips of C5–C6 and C6–C7 and are important because the lateral radiograph may not visualize C6–C7. Unilateral facet dislocations produce rotation of the spinous processes in the transverse plane.
Information obtained from the lumbar spine AP and lateral films is similar to that of the thoracic spine; however, the oblique radiographs produce the well-known “Scottie dog,” which provides an excellent view of the pars interarticularis. In the right posterior oblique view the right superior articular facet is the dog’s ear, the right pedicle is the eye, the right transverse process is the nose, the right inferior articular facet is the front leg, the right lamina is the body, the left superior articular facet is the tail, and the left inferior articular facet is the hind leg (see Fig. 16-7). Fractures of the “neck” of the dog indicate spondylolysis (Fig. 16-17; see Fig. 16-7). Spondylolysis is associated with spondylolisthesis (anterior slippage of a vertebral body), both of which are readily detectable on plain films and are discussed later in this chapter.
NORMAL MAGNETIC RESONANCE APPEARANCE OF THE SPINE
The beauty of MR is its ability to provide multiplanar images of both the bone and soft tissues of the spine. In the adult, the normal vertebral marrow generally has intermediate to high signal intensity on T1-weighted imaging (T1WI) and low signal intensity on conventional T2-weighted imaging (T2WI) with bone marrow hypointense to the disk and CSF hyperintense (Fig. 16-18). This appearance is variable, depending on the exact pulse sequence and the age of the patient. On T2WI fast spin-echo images, the normal vertebral marrow is high intensity, making lesion detection in the vertebral body more challenging. Fat saturation or short tau inversion recovery (STIR) sequences are important when vertebral body lesions are suspected and fast spin-echo techniques are being employed. However, the saturation may not be uniform, particularly when large fields of view are employed.
DEGENERATIVE DISEASES
Initially, the nucleus pulposus is soft and gelatinous; however, with aging it is replaced by fibrocartilage, and the distinction between nucleus and annulus fibrosus becomes less well defined. The cartilaginous endplate becomes fissured and more hyalinized. The annulus, which is initially attached to the anterior and posterior longitudinal ligaments, loses its lamellar configuration and develops fissures. The cracks have negative pressure so that gas, primarily nitrogen, comes out of solution and deposits in the intervertebral disk, close to the subchondral bone plate or in other locations. This is termed the vacuum (cleft) phenomenon (Fig. 16-19). These degenerative changes permit disk material to bulge and subsequently to herniate. Remember that disk herniation may also occur in the absence of significant disk degeneration.
Disk calcification commonly occurs in the elderly and is part of the normal aging process. It is also associated with other conditions (Box 16-1). Increased intensity of the disk on T1WI can be seen uncommonly with mild calcification associated with degeneration. As calcification increases, the intensity on T1WI decreases.
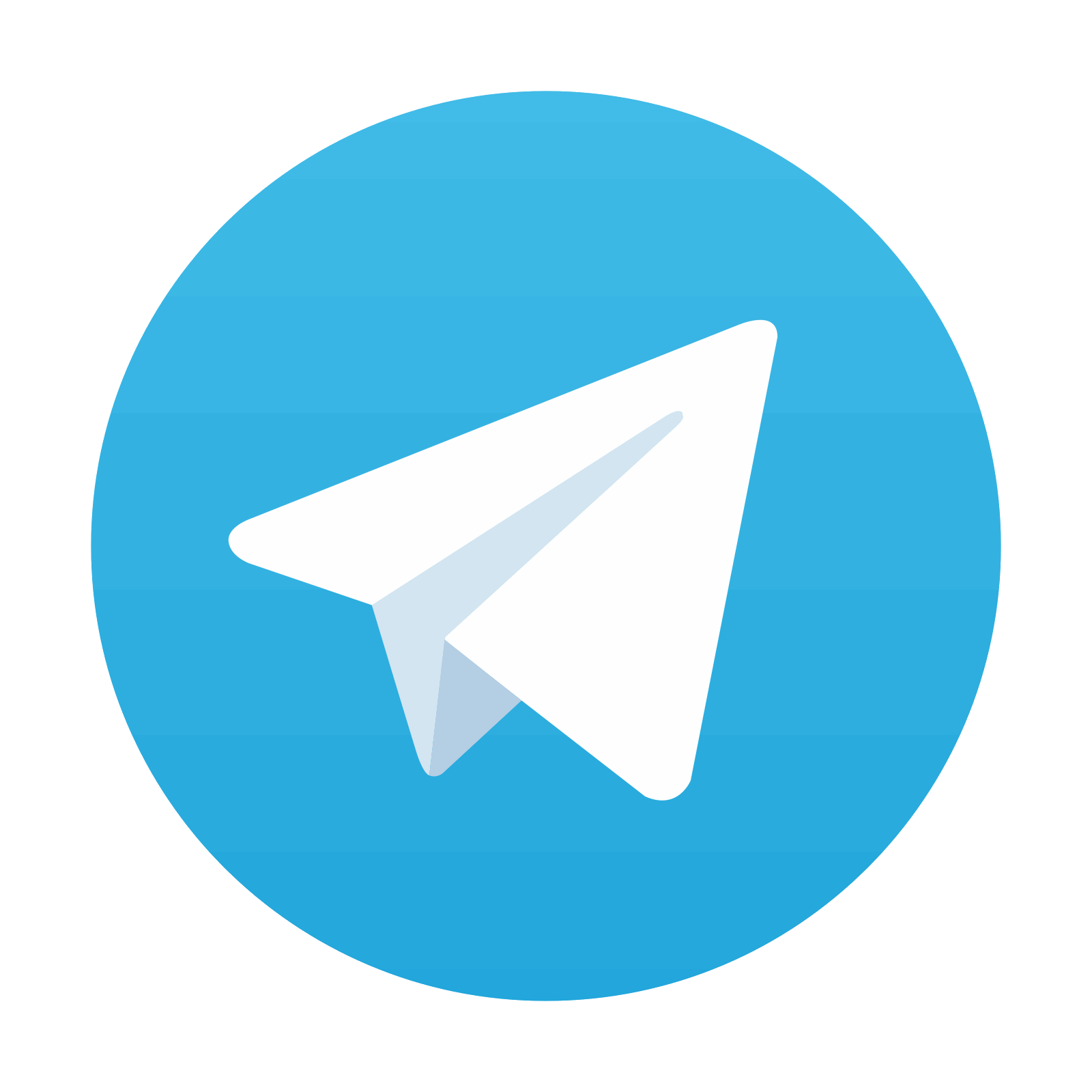
Stay updated, free articles. Join our Telegram channel

Full access? Get Clinical Tree
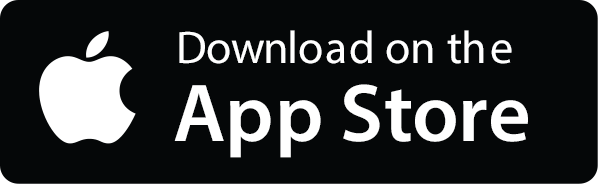
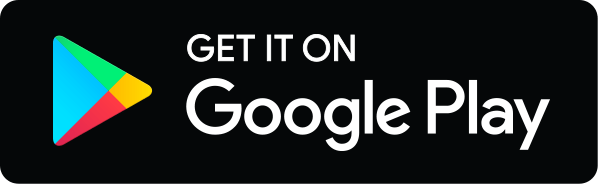