CHAPTER 41 ANATOMY AND PHYSIOLOGY OF CEREBRAL AND SPINAL CORD CIRCULATION
Of all the mammalian organs, the central nervous system is the most privileged and protected. Claude Bernard developed the now near-axiomatic concept of the maintenance of the “milieu interieur.” It is essential to recognize that the stability of the internal environment of the brain is primordial and that the homeostasis of other organs is subordinated to the vital stability of the neuronal environment in the central nervous system. Before entering into the anatomical and the physiological details that are specific to the brain, it is worth reflecting on the major general systems that protect the body—above all, the central nervous system.
ANATOMY OF THE CEREBRAL CIRCULATION
Arterial Supply
The arterial supply to the human brain consists of four major afferent arterial trunks: two internal carotid and two vertebral arteries. The internal together with the external carotid arteries derive from the common carotid artery. In humans, the carotid arteries are quantitatively more important; each contributes approximately 40% to the total perfusion of the brain. The internal carotid arteries enter the cranial cavity through the os petrosum. The vertebral arteries enter the cranial cavity through the foramen magnum, where, after traversing the anterolateral aspect of the medulla oblongata, they fuse to form the basilar artery at the level of the pontomedullary junction (Fig. 41-1). This artery unites with the two internal carotids to form, at the base of the brain, an equalizing distributor named the circle of Willis (see Fig. 41-1). Having fused with the basilar artery, each internal carotid artery divides into four major branches: the anterior cerebral, the middle cerebral, the anterior choroidal, and the posterior communicating arteries. The latter anastomose with the posterior cerebral arteries, which originate from the basilar artery to complete the circle of Willis (see Fig. 41-1). These major cerebral arteries divide into progressively smaller arteries, which, in turn, enter the brain parenchyma at a right angle to the surface of the brain to supply blood to specific regions. The anterior cerebral arteries irrigate the frontal pole and the medial aspects of both frontal and parietal lobes, the corpus callosum, the anterior limb of the internal capsule, and the most rostral part of the caudate nucleus and the putamen. The middle cerebral arteries supply most of the lateral aspects of the cerebral hemispheres, as well as portions of the caudate nucleus and the putamen. The anterior choroidal arteries supply several structures such as the choroid plexus of the lateral ventricle, the optic tract, the hippocampus, the tail of the caudate nucleus, and the amygdala. The posterior communicating arteries supply the genu of the corpus callosum, part of the posterior limb of the internal capsule, the rostral thalamus, and the wall of the third ventricle. The posterior cerebral arteries supply the inferior and medial aspects of the temporal and the occipital lobes, parts of the hippocampus, and the thalamus (Fig. 41-2). Before giving rise to the posterior cerebral arteries, the basilar artery sends several branches to supply the cerebellum and the brainstem (see Fig. 41-1).
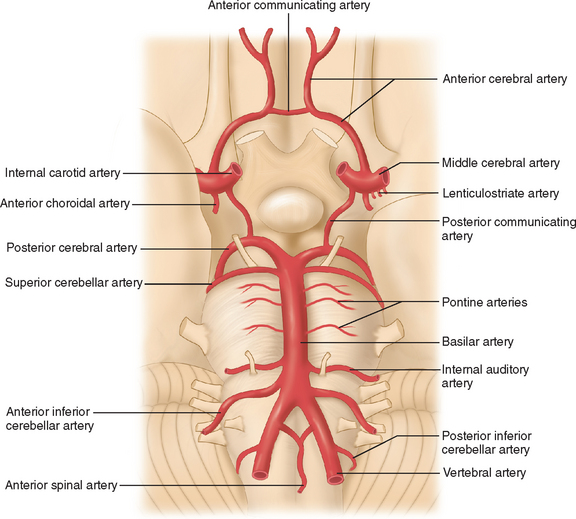
Figure 41-1 Basal view of major cerebral arteries and the circle of Willis.
(From Sokoloff L: Anatomy of the Cerebral Circulation. In Welch KMA, Caplan LR, Reis DJ, et al, eds: Primer on Cerebrovascular Diseases. Academic Press, 1997.)
Collateral Blood Supply of the Brain
Several types of anastomoses are found in the cerebral circulation.1 The following are the most important.
Circle of Willis
As mentioned, the circle of Willis is an anastomosis between the anterior and the posterior circulation via the anterior and the posterior communicating arteries (see Fig. 41-1). Considerable intraspecies and interspecies variability exists in the anatomy of the circle of Willis. In humans, a symmetrical circle is found in only 50% of brains.2 In addition, in most species other than primates, the anterior cerebral communicating artery is absent and the anterior cerebral arteries fuse earlier to form the pericallosal artery.3 This anastomotic ring protects from the disastrous consequences of occlusion of a single supply vessel to the brain. However, under physiological conditions, the blood from the internal carotid and the basilar arteries does not mix because the blood pressure in each arterial trunk is almost identical.
External Carotid-Internal Carotid Anastomoses
In humans, this anastomotic pathway is relatively negligible. The ophthalmic artery is the most significant conduit that is capable of bridging the external and internal circulations. However, in many species such as the dog, cat, and sheep, rich and complex connections exist between the internal and external carotid arteries via a structure termed the rete mirabile.3
Leptomeningeal Anastomoses
The leptomeningeal anastomoses, organized in the subarachnoid space between the arterial boundary zones, represent connections between distal branches of major cerebral arteries (i.e., between the anterior cerebral and middle cerebral arteries and between the middle cerebral and the posterior cerebral arteries) (Fig. 41-3). These arterial boundary zones, also termed “watershed zones,” are especially susceptible to damage following any generalized decrease in blood flow as in the case of severe systemic hypotension.
Venous Drainage
The venous circulation of the central nervous system is particular in that (1) the veins do not run parallel to arteries as in many other organs and (2) the major fraction of blood that drains the brain is collected in the dural sinuses, which represent the final intracranial collecting blood vessels.4 Briefly, there are three groups of valveless vessels that allow for drainage. These are the superficial cortical veins located on the surface of the cortex, the deep or central veins, and the venous sinuses within the dura (Fig. 41-4). The superficial veins convey blood from the cortex and the adjacent white matter and empty into the dural venous sinuses. The deep cerebral veins drain blood in a centripetal direction from the deep white matter, the basal ganglia, and the diencephalon toward the lateral ventricles. Large subependymal veins empty into the internal cerebral and basal veins, which unite and contribute to the formation of the great cerebral vein also known as the “vein of Galen.” The cerebral venous system is also exceptional in that these vessels are endowed with arachnoid villae, which allow cerebrospinal fluid and various metabolites to drain into the systemic circulation.
Blood Supply to the Spinal Cord
A network of irregular anastomosing arteries connects the ventral spinal and dorsolateral arteries. The dorsolateral arteries and their anastomosing branches give rise to radial arteries that supply both gray and white matter in the spinal cord. Each body segment has paired spinal arteries that give rise to a dorsal root artery and a ventral root artery, which follow the nerve connections on each side of the cord. These arteries feed into a spinal arterial ring that surrounds the cord at the level of each intervertebral foramen. The venous architecture of the spinal cord is considerably more variable than the corresponding arterial distribution, and the blood vessels are more tortuous. There tends to be a prominent midline vein that in general follows the course of the posterior median sulcus. Otherwise, the veins follow the same pattern as the intrinsic, radial, and segmental arteries (for details, see Shamji et al5).
Microcirculation
The main arteries enter the subarachnoid space and divide many times before penetrating the substance of the brain to form smaller arterioles and capillaries. The smallest pial arteries enter the brain parenchyma at a right angle to the surface of the brain. These pial arteries are formed by endothelial and smooth muscle cell layers as well as an outer layer of cells, termed the adventitia, which contains collagen, fibroblasts, and perivascular nerves. The penetrating arterioles are surrounded by an invagination of the pia matter, creating a perivascular space (Virchow-Robin space) that is contiguous with the subarachnoid space. As the arterioles penetrate deeper into the brain, this space disappears and the vascular basement membrane comes into direct contact with the astrocytic end-feet (intracerebral arterioles and capillaries). Capillaries are formed by one layer of endothelial cells and show a marked heterogeneous distribution that is correlated with synaptic density and local energy metabolism.6,7 In most regions of the brain, endothelial cells are unique in that they lack fenestrations and are interconnected by specific intercellular junctions, known as tight junctions. These morphological features, in conjunction with metabolic activity that is essentially limited to cerebral endothelial cells, constitute the blood-brain barrier that excludes large molecules, neurotransmitters, and toxins by both forming a physical barrier and lacking the typical transport mechanisms that operate in blood vessels most in other regions of the body. It should be emphasized, however, that the endothelial cell is more than the anatomical interface between cerebrovascular muscle and the blood; it plays an important functional role in the regulation of cerebral blood flow.8 Indeed, the cerebrovascular endothelium is able to produce a variety of vasodilatatory and constrictory agents. Intraparenchymal blood vessels are almost completely ensheathed by astrocyte processes. Recent reports indicate that astrocytes are involved in local regulation of blood supply in response to neuronal activation.9,10 In the central nervous system, pericytes are found and are closely apposed to the abluminal surface of the capillaries of which they cover, on average, 25%. The function of pericytes is not well known, but some studies suggest that they may influence the diameter of capillaries because they display contractile properties.11
One distinguishing feature of the cerebrovascular bed is the presence of a rich and complex innervation.12 Large intracranial and pial vessels are densely innervated by perivascular nerves that originate from autonomic and sensory ganglia (extrinsic innervation) and contain many agents that can potentially modify vascular tone. Intracerebral arterioles and capillaries are contacted by neural processes that originate from local interneurons or from central pathways (intrinsic innervation). These processes also contain many vasoactive substances. These neurally produced transmitters and modulators are likely to participate in the control of the microvascular tone and, thereby, local cerebral blood flow.
Physiology of the Cerebral Circulation
Pressure-Flow Relationships
If the cerebral vascular bed was a system of nondistensible pipes, cerebral blood flow (F) would be, according to Ohm’s law, a simple function derived from the perfusion pressure (the difference between arterial inflow and downstream pressure ΔP) divided by the resistance to flow along these pipes (F = ΔP/R). Resistance to flow is determined by the caliber of the vascular segment, its length, and the nature of the fluid that flows along it. By analogy to Poiseuille’s equation that applies to a rigid system of tubing perfused by a newtonian fluid:
where F is flow, r is vascular radius, ΔP is the pressure gradient between inflow and outflow, η is viscosity, and L is length. Although this equation cannot be totally applicable to the cerebrovascular bed, because it is not a rigid system, its effective length is not known, and blood is a non-newtonian fluid, Poiseuille’s law can reasonably describe the fundamental relationships between cerebral blood flow, perfusion pressure, and resistance. Of great interest, this equation emphasizes that cerebral blood flow is related to the fourth power of vessel radius; thus even minor changes in arterial diameter have a significant impact on cerebral blood flow.
Cerebral perfusion pressure is the difference between intra-arterial pressure where the vessels enter the subarachnoid space and pressure in the thin-walled veins in the subarachnoid space. Venous pressure changes in parallel to intracranial pressure and is normally 2 to 5 mm Hg higher than intracranial pressure. Under physiological conditions, the intracranial pressure is determined by the volume of three compartments: brain parenchyma, quantity of cerebrospinal fluid, and intravascular blood pool. Because of the rigidity of the cranium, increases in the size of one component of the intracranial contents must be accompanied by removal of an equivalent amount of another otherwise intracranial pressure will increase.13
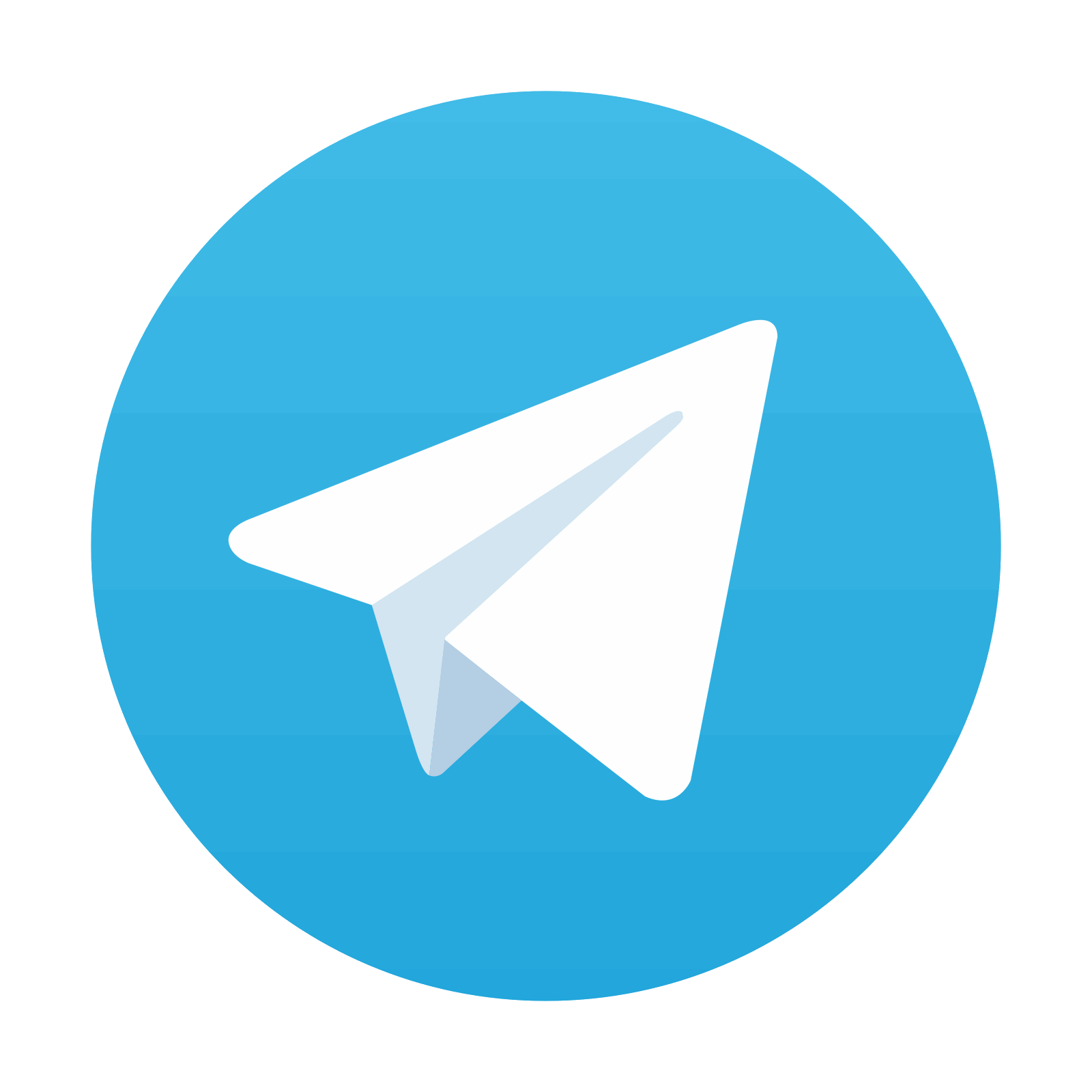
Stay updated, free articles. Join our Telegram channel

Full access? Get Clinical Tree
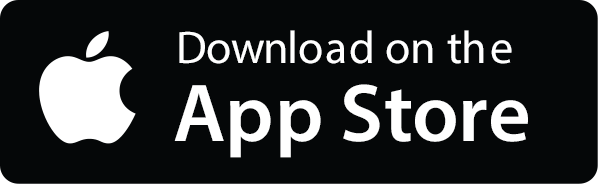
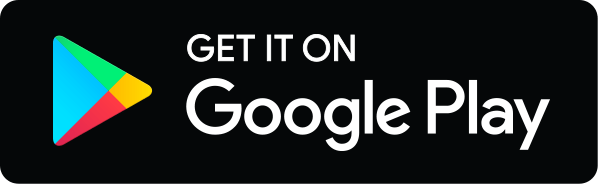