Fig. 2.1
The human body in anatomic position
The human body has a line of symmetry that bisects the body into right and left equal halves. This line of symmetry is known as the midline. Structures relatively further from midline than a reference structure are lateral, while structures lying closer to midline are said to be medial to the reference structure (Fig. 2.2).
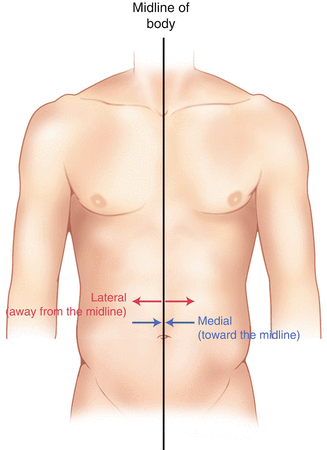
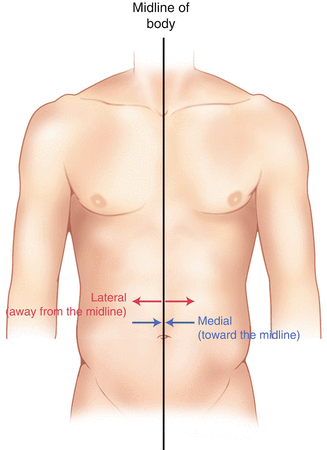
Fig. 2.2
Drawing of a human torso with the anatomic midline shown in black. Arrows indicate the relative terms of medial and lateral
The terms proximal and distal refer to locations that are closer to or further away from the point of attachment of a limb. These terms are often used to describe the position of structures along a limb relative to each other. For example, the elbow is distal to the shoulder but proximal to the wrist.
Superior and inferior are terms that refer to the position of a structure either above or below a reference point, respectively. For example, one can expect to find the nose superior to the chin in most people.
Humans, being bipedal, require some additional terminology than our quadruped friends. In other organisms, the terms anterior and posterior have different meanings than dorsal and ventral. In humans we often speak of the anterior and posterior portions of a limb or the torso. The anterior side is “belly side,” and the posterior is “back side.” These terms are synonymous with ventral and dorsal, respectively (Fig. 2.3). We begin to confuse these terms when considering directionality along the neuraxis.
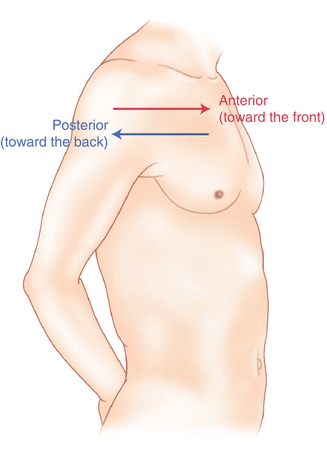
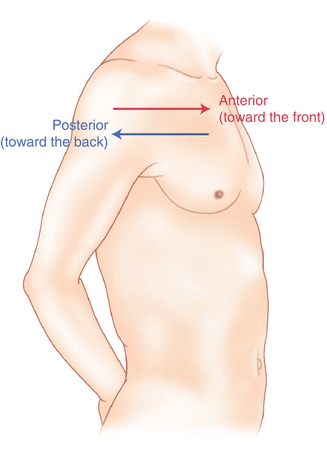
Fig. 2.3
A lateral drawing of the human trunk with arrows indicating the relative terms of anterior and posterior
The curvature of the neuraxis necessitates the introduction of two additional directional terms: rostral and caudal. The term rostral (from the Latin for nose) refers to points located further toward the nose on the neuraxis than the referenced structure. Caudal (from the Latin for tail) refers to points located further toward the end of the spinal cord. The rostral–caudal axis will bend with the neuraxis (most notably as you approach the level of the cerebral cortex). The dorsal–ventral or anterior–posterior plane is perpendicular to the rostral–caudal axis at any given point (Fig. 2.4).
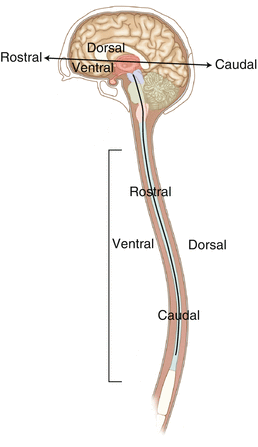
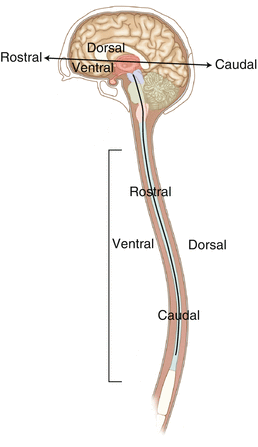
Fig. 2.4
A drawing of the neuraxis illustrating the relative terms rostral, caudal, dorsal, and ventral
Pathways moving from the peripheral nervous system toward the central nervous system are termed afferent pathways. These pathways are sensory. Pathways that travel from the central nervous system out toward the periphery are termed efferent pathways. These pathways carry motor information.
Organization of the Nervous System
The nervous system can be divided both anatomically and functionally. Anatomically we divide the nervous system into the central and peripheral nervous system. The central nervous system (CNS) consists of the brain, spinal cord, and the retina. The peripheral nervous system (PNS) consists of all of the nerves that come off of the brain (cranial nerves) and spinal cord (spinal nerves), nerve plexuses, and peripheral nerves innervating the various structures of the body.
Functional divisions of the nervous system include the somatic and autonomic divisions. The somatic nervous system governs voluntary actions and provides motor output through the action of the skeletal muscles. We will spend the majority of time discussing the somatic nervous system, as it is the division that is amenable to neuromonitoring. However, a brief consideration of the autonomic nervous system is warranted.
Autonomic Nervous System
The autonomic nervous system governs “automatic” visceral or vegetative functions and operates generally at the unconscious level. Examples of functions under autonomic control include respiration, heart rate, digestion, and sexual arousal. The autonomic nervous system has two divisions: sympathetic and parasympathetic (Fig. 2.5).
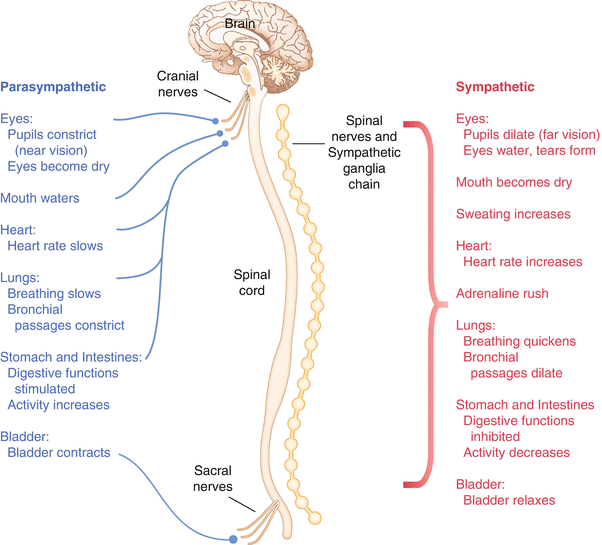
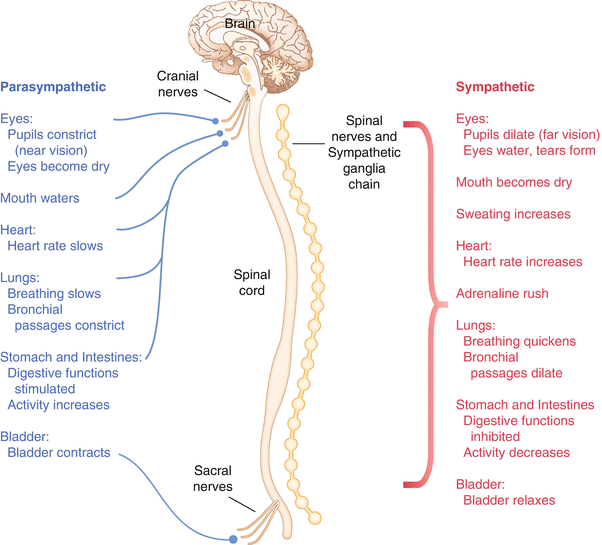
Fig. 2.5
The organization and function of the autonomic nervous system are shown. The parasympathetics are shown in blue on the left originating from cranial nerve nuclei and sacral spinal nerves. On the right, shown in red, are the sympathetics originating from thoracolumbar spinal nerves
The sympathetic nervous system has its anatomic origin from the thoracolumbar segments of the spinal cord, which is why it is sometimes called the thoracolumbar division of the autonomic nervous system. The sympathetics are responsible for the well-known “fight or flight” response. Consider what would happen if you were to encounter a bear in the woods (or any foreboding situation that you can imagine). Your body would prepare to either fight the bear or run from it. To do this you would need increased oxygen delivery to your muscles. The result is an increase in respiration and heart rate. Your pupils would dilate in order to increase visual acuity, and your hair would stand on end so that you may appear more ferocious to the bear (this last part may be an evolutionary leftover).
The parasympathetic division is anatomically located on either side of the sympathetic division and is alternately called the cranial–sacral division. The prefix “para” meaning “alongside” will remind you of the location. The parasympathetic division of the autonomic nervous system regulates visceral functions at rest. The phrase “rest and digest” summarizes the function of the parasympathetic system. You will study the cranial nerves in another section, but if a cranial nerve has an autonomic function, you can be sure it’s parasympathetic. Again, remember that the parasympathetics have cranial or sacral origins. The vagus nerve (CNX), for example, is the largest parasympathetic nerve in the body.
Somatic Nervous System
The somatic nervous system governs voluntary movement as well as sensory processing of sensory information from external stimuli (such as light, sound, and touch). The somatic nervous system is that division that is tested during intraoperative monitoring. The somatic nervous system works through the activation of skeletal muscles and exteroceptors.
When discussing the somatic nervous system, it is common to specify between sensory and motor pathways. Sensory pathways are those that are activated by sensory stimuli in the environment and transmit this information to the central nervous system. There are many different sensory stimuli in our environment from light and sound to pain and temperature and many modalities in between. In order to be interpreted by the nervous system, these sensory modalities must be changed into electrical impulses. The process by which sensory stimuli are converted to electrical impulses for use by the nervous system is called transduction.
All sensory neurons have specialized endings called exteroceptors that are specific for the sensory modality they mediate. For example, exteroceptors in the retina are called photoreceptors. Hair cells are the sensory cells of the auditory system. Sensory neurons that mediate various types of mechanical stimuli in the skin have different names like Pacinian corpuscles for deep touch and pressure or Meissner’s corpuscles for light touch (Fig. 2.6). Proprioception, knowing where your limbs are in space, is mediated by stretch receptors located in the muscles.
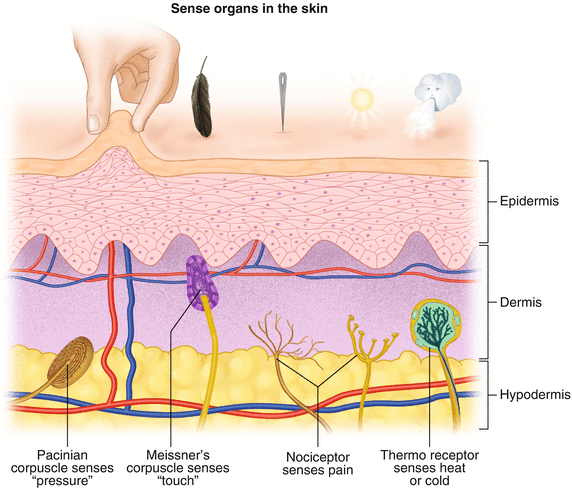
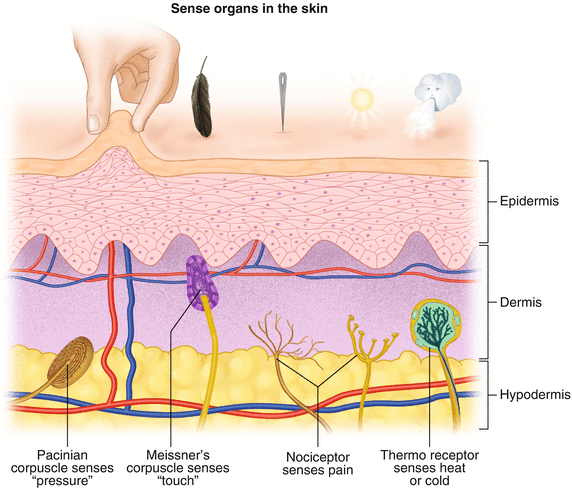
Fig. 2.6
Various sensory organs of the skin along with their function
We are able to stimulate and record from both sensory and motor pathways in order to assess their function either in the clinic or during surgery. We will continue our discussion of neuroanatomy with a focus on sensory and motor tracks of the somatic nervous system. In subsequent chapters you will learn how we can stimulate and record from these anatomical substrates for the purposes of intraoperative monitoring.
Brain Anatomy
The human brain is arguably the most complex structure in existence. The brain governs all of human behavior. Vegetative functions are controlled by the lower brain structures of the brainstem, while reasoning and other distinctly human behaviors are a result of processing in higher brain centers such as the cerebral cortex. An understanding of the overall structural organization of the brain with a focus on sensorimotor processing is required for the IOM clinician to properly plan, carry out, and interpret a monitoring session. In this section we will discuss the gross anatomical organization of the brain including topographical organization. We will consider some important neural pathways in a subsequent section.
The Skull
The brain is protected by a bony structure called the cranium. The cranium, along with the mandible, makes up the skull (Fig. 2.7). The uppermost part of the skull is known as the skull cap or calvarium. The skull serves as a protective case for the brain. Bones of the skull are joined together by special immobile joints known as sutures. In infants, the skull bones are separated by cartilaginous areas known as fontanelles (Fig. 2.8). The fontanelles allow for expansion of the skull to accommodate the growth of the brain. The fontanelles are mostly ossified by 2 years of age.
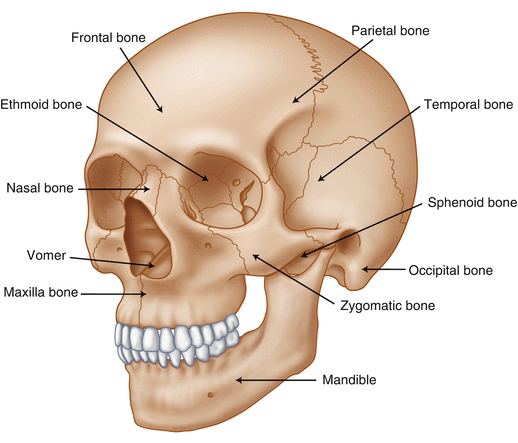
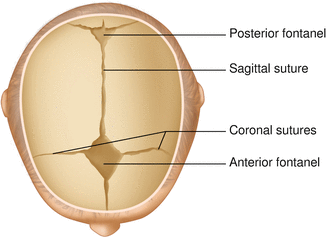
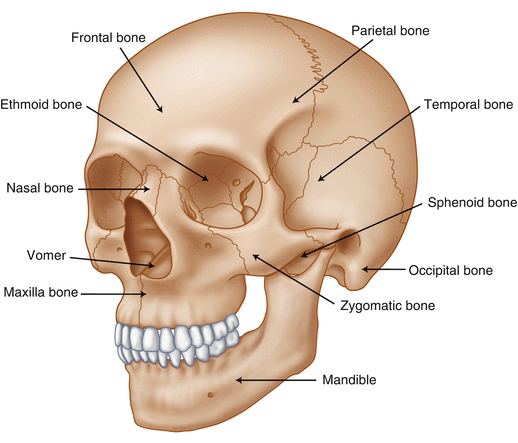
Fig. 2.7
The cranial bones including the frontal, parietal, temporal, and occipital bones along with the mandible comprise the complete human skull
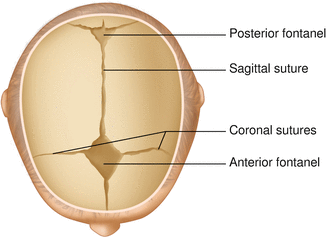
Fig. 2.8
Superior view of the infant skull showing the anterior and posterior fontanelles along with the development of the sagittal and coronal sutures
The Meninges
The brain and spinal cord are further protected by a system of membranes called meninges. The meninges consist of three layers that are anatomically continuous: the dura mater, the arachnoid mater, and the pia mater. The term dura mater is from the Latin literally meaning “tough mother,” while pia mater means “soft mother.” The word arachnoid implies a spider weblike quality. The function of the meninges is to protect the brain and contain cerebrospinal fluid (Fig. 2.9).
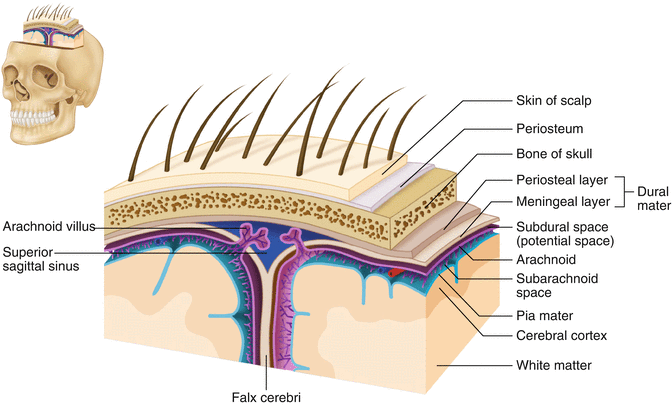
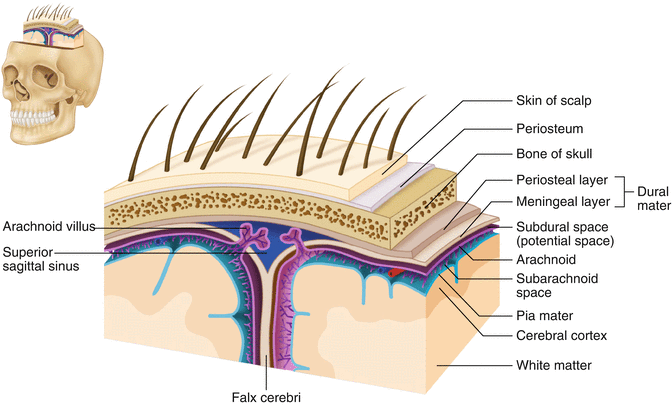
Fig. 2.9
The organization of the meninges and meningeal spaces of the brain
The dura mater is the fibrous outermost layer of the meningeal membranes. This layer contains larger blood vessels as well as sensory nerve fibers. Among the blood vessels present in the dura mater are large venous sinuses that return blood and cerebrospinal fluid from the brain back to the heart. There are two dural extensions that you should be familiar with. The falx cerebri separates the cerebral hemispheres and the tentorium cerebelli separates the occipital lobe from the cerebellum (Fig. 2.10).
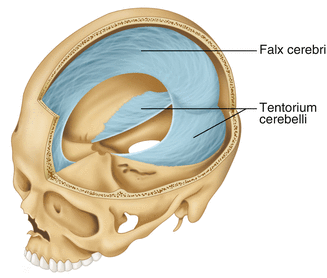
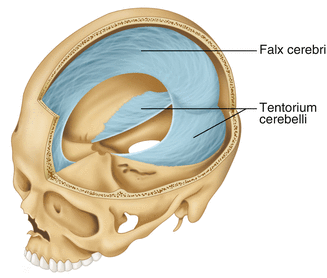
Fig. 2.10
The specialized dural infoldings: falx cerebri and tentorium cerebelli
The arachnoid mater is thinner than the dura and resembles a loose fitting sac for the brain. Thin filaments known as arachnoid trabeculae extend from the arachnoid to the pia mater.
The pia mater is the thinnest layer of the meninges and closely adheres to the surface of the brain and spinal cord following each gyrus and sulcus. The pia has an extensive capillary network that nourishes the surface of the brain and spinal cord.
There is a normally occurring space between the arachnoid and pia mater. This subarachnoid space is filled with cerebrospinal fluid. Bleeding into this space as a result of trauma or the spontaneous rupture of a blood vessel can enlarge this space causing compression on the brain. This is known as a subarachnoid hemorrhage and requires surgical intervention to decompress the neural tissue. The dura and arachnoid are normally attached closely. Occasionally bleeding resulting from trauma or disease will occur opening up a space between the dura and arachnoid that does not normally exist. This potential space is called the subdural space, and the resulting bleed or clot would be known as a subdural hemorrhage or subdural hematoma, respectively.
The Ventricles
There are a series of canals within the brain whose function is to circulate cerebrospinal fluid. These are known as the cerebral ventricles (Fig. 2.11). There are two paired (left and right) lateral ventricles, a third ventricle, and a fourth ventricle. The ventricles communicate with each other via foraminal openings and are continuous with the central canal of the spinal cord. The left and right ventricles communicate with the third ventricle through the intraventricular foramina (of Monro). The third ventricle communicates with the fourth through the cerebral aqueduct (also known as the aqueduct of Sylvius). Cerebrospinal fluid returns to the subarachnoid space and venous circulation from the fourth ventricle via the midline foramen of Magendie and two paired foramina of Luschka.
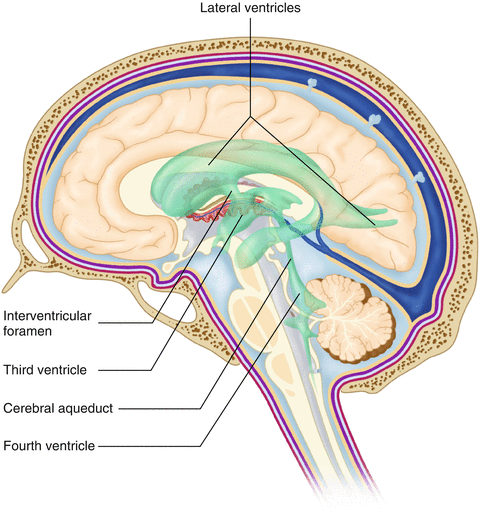
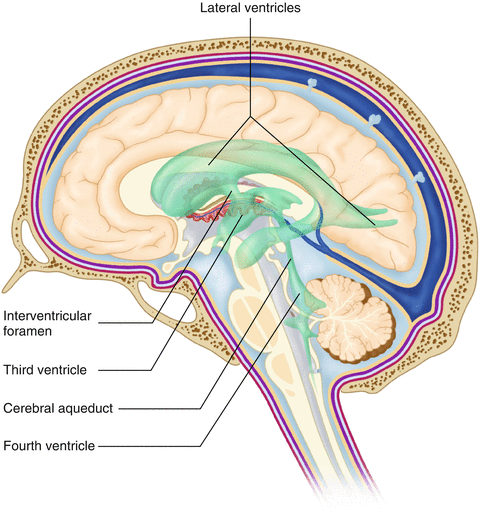
Fig. 2.11
The organization of the cerebral ventricles
The cerebrospinal fluid (CSF) bathes and cushions the brain and spinal cord and is contained within the dura mater. The CSF is produced by tufts of tiny capillaries called choroid plexus that are found within the ventricles (Fig. 2.12). CSF is simply filtrated blood plasma. CSF comes from the blood and must eventually return to the blood. The presence of microorganisms or white blood cells in CSF indicates infection within the central nervous system. CSF can be sampled for diagnostic purposes by lumbar puncture.
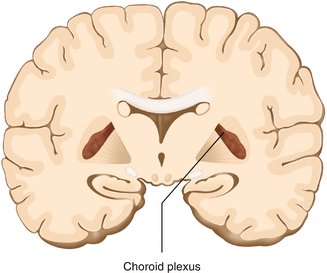
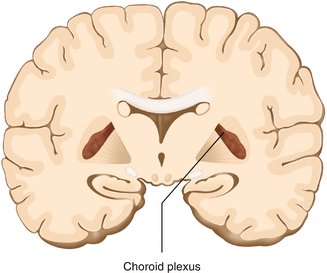
Fig. 2.12
The choroid plexus lines the ventricles making CSF
The Cerebral Lobes
The cerebral cortex can be divided into four paired functional lobes: frontal, parietal, temporal, and occipital (Fig. 2.13). The cortex is the substrate for all higher order sensorimotor processing and cognitive functioning. If you recall the adage “structure subserves function,” it won’t be a surprise that the wrinkled structure of the cerebral cortex serves to increase surface area without necessitating an obnoxiously large skull to house the brain! Think of this as folding a piece of paper up to fit into your pocket. The bumps along the brain are called gyri (the singular form is gyrus), and the grooves separating the gyri are known as sulci (the singular form is sulcus). Each gyrus and sulcus of the brain has its own name. We will just consider a few.
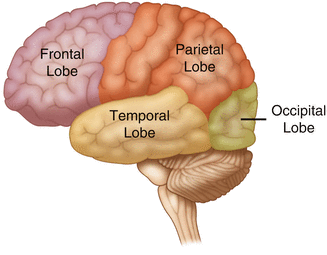
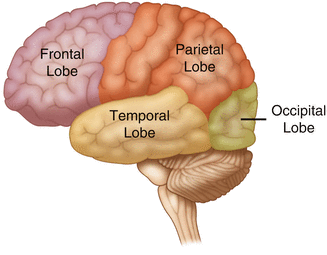
Fig. 2.13
The anatomic lobes of the cerebral cortex
The frontal lobe is responsible for higher order executive functioning such as personality, inhibition, and long-term memory. From the perspective of the intraoperative monitoring clinician, the frontal lobe is important because it governs voluntary motor function. Premotor and supplementary motor areas of the frontal lobe are not completely understood but are generally accepted to participate in planning of movement and coordination of sensory and motor information. Direct voluntary motor control, however, begins on one particular gyrus of the frontal lobe known as the precentral gyrus. The precentral gyrus gets its name from its location anterior to the central sulcus. The central sulcus separates the frontal from the parietal lobes of the cerebral cortex. The precentral gyrus is also known as the primary motor cortex. Neurons of the precentral gyrus project directly to the spinal cord to influence voluntary movement.
Logic tells us that if there is a precentral gyrus then there must be a postcentral gyrus. This gyrus is located in the parietal lobe just posterior to the central sulcus and is also known as the primary sensory cortex. Neurons in the postcentral gyrus receive input from the somatic sensory pathways (Fig. 2.14).
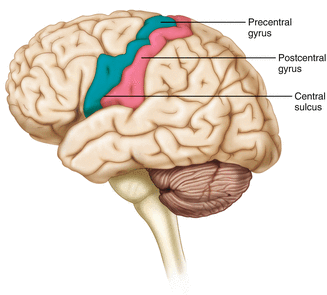
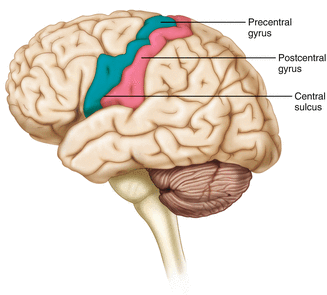
Fig. 2.14
The central sulcus shown with the precentral gyrus (primary motor cortex) and postcentral gyrus (primary sensory cortex)
The lateral sulcus (also known as the Sylvian fissure) separates the frontal and parietal lobes from the temporal lobe. The temporal lobes are responsible for the formation of short-term memories as well as speech and language comprehension.
The occipital lobes are the most posterior lobes of the cerebral cortex. The occipital lobes are the site of visual processing. The calcarine sulcus separates the parietal and occipital lobes. This sulcus runs deep and is not completely visible from the cortical surface.
Topographical Organization
A common theme running through the nervous system is topographical organization. One of the main examples of topographical organization within the brain is the homunculus. The primary motor and primary sensory cortex are functionally organized such that a visual representation of the body may be reconstructed on them (Fig. 2.15). There are slight differences between the motor homunculus and sensory homunculus, but overall they are quite similar. You will notice that the hands and feet of the homunculus are quite large. This is because there is a proportionately large amount of surface area dedicated to these structures on the cerebral cortex. The hand and face areas are represented laterally, while the lower extremities and genitalia are represented medially. Correlating homuncular topography with cerebral blood supply and recorded electrical potentials will be important for intraoperative monitoring. You will soon learn that the middle cerebral artery supplies the lateral portions of the cerebral hemispheres and the anterior cerebral artery supplies the medial portion of the hemispheres. Deficits resulting from an MCA territory infarct will present clinically in the upper extremity and face. Deficits resulting from an ACA infarct will present clinically in the lower extremity. The homunculus has practical use when determining sites to stimulate and record from the brain for intraoperative monitoring.
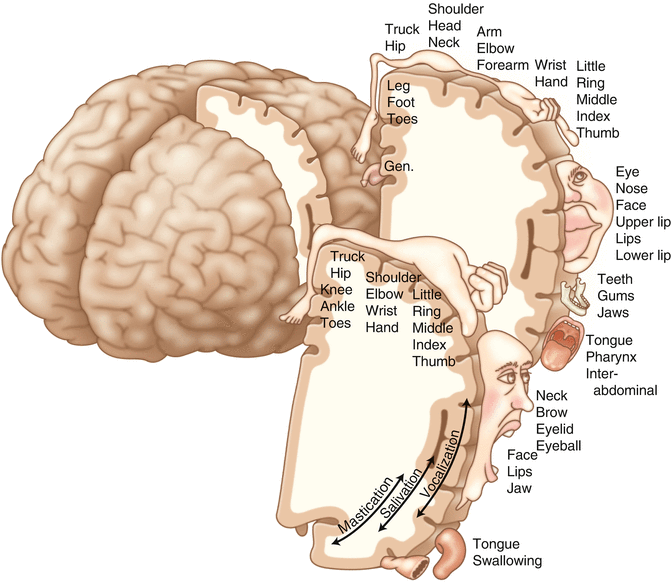
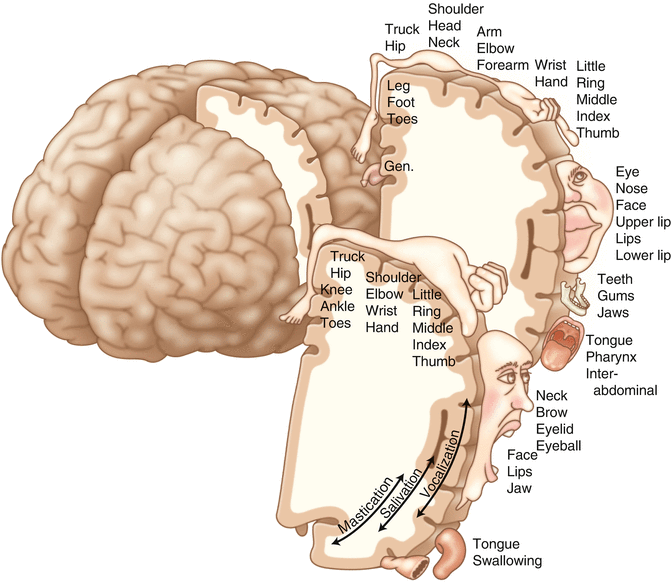
Fig. 2.15
Drawing of the motor and sensory homunculus. Notice that the face and upper extremity are represented laterally and the lower extremity and genitalia are represented medially
The Diencephalon
The diencephalon is a region of the neuraxis, rostral to the midbrain, composed of a group of deep brain structures including the thalamus and hypothalamus (Fig. 2.16). The hypothalamus is often called the master endocrine gland of the body. The thalamus is a bilateral structure composed of several functionally distinct nuclei (Fig. 2.17). Sensory information on its way to the cortex is first processed in the thalamus. The thalamus generates potentials that can be recorded from the scalp during intraoperative monitoring. Blood supply to the thalamus is from the posterior cerebral circulation making thalamic generated evoked potentials useful for monitoring posterior ischemia.
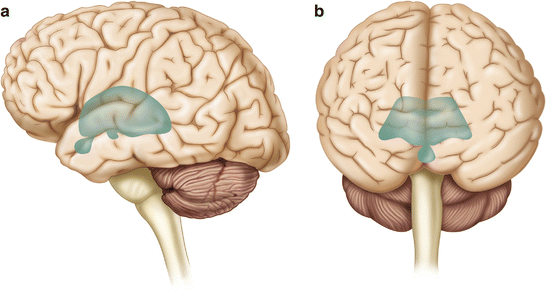
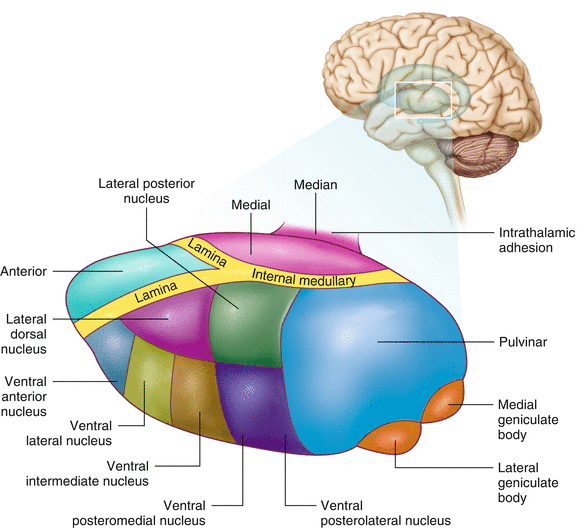
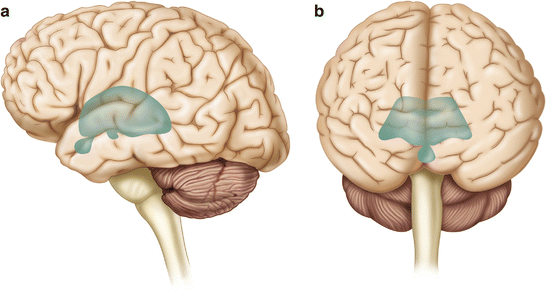
Fig. 2.16
Lateral (a) and anteroposterior (b) views of the diencephalon (shaded) within the neuraxis
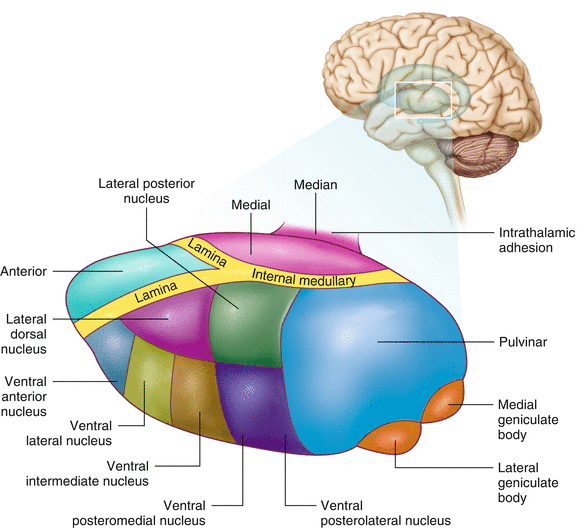
Fig. 2.17
Organization of the human thalamus
The Brainstem
The brainstem is composed of the midbrain, pons, and medulla oblongata and is continuous with the spinal cord (Fig. 2.18). The brainstem contains sensory and motor tracts passing information to and from the higher brain centers as well as cranial nerve nuclei. The cranial nerve nuclei contain the cell bodies whose axons form the cranial nerves, which supply sensory and motor innervation to the head, neck, and face. Vegetative functions are controlled by the brainstem including the heart rate, respiration, and aspects of the sleep–wake cycle. Intraoperative monitoring of various brainstem potentials can protect these vital structures and functions during surgery. We will discuss some of the brainstem structures in more detail in subsequent sections.
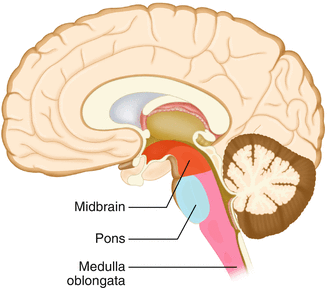
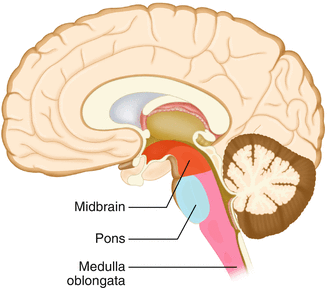
Fig. 2.18
Lateral view of the brainstem (midbrain, pons, and medulla oblongata) within the neuraxis
The Cranial Nerves
The cranial nerves emerge from the brain and innervate structures of the head and neck (Fig. 2.19). There are 12 cranial nerves that are motor, sensory, or mixed nerves. Some of the cranial nerves even have autonomic function, specifically parasympathetic function. Each nerve has a unique name but may also be designated by its number. The numerical designation takes the form of “CN” followed by the Roman numeral assigned to the nerve. For example, the facial nerve is also designated CNVII. You should be familiar with both the names and numerical designations of all 12 cranial nerves.
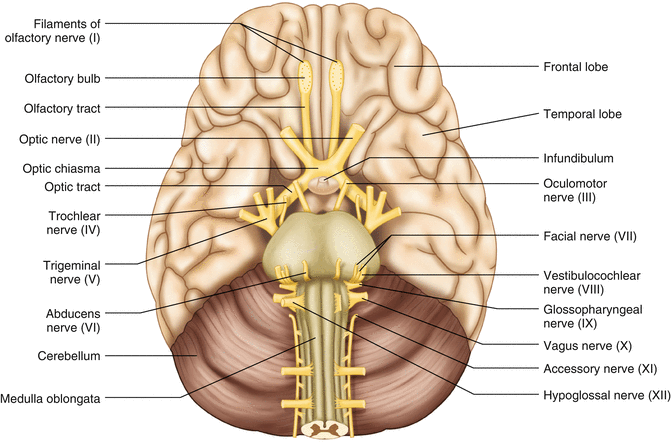
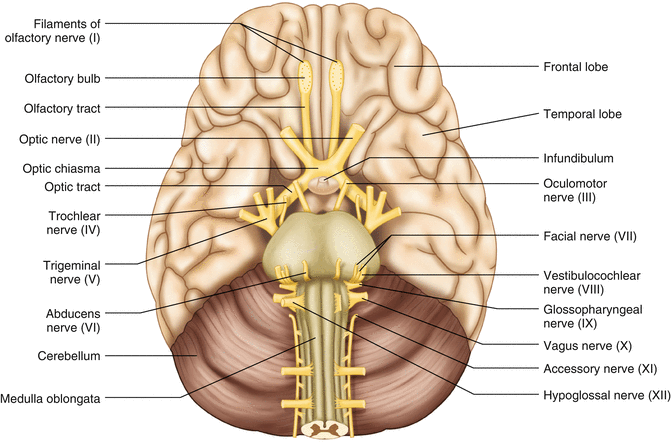
Fig. 2.19
Ventral view of the brain showing the 12 cranial nerves
Table 2.1 lists all of the cranial nerves as well as information about each nerve that you should know. There is only one cranial nerve that cannot be monitored and that is CNI, the olfactory nerve, which mediates the sense of smell.
Table 2.1
The cranial nerves
Number | Name | Modality | Function | How monitored |
---|---|---|---|---|
I | Olfactory | Sensory | Smell | N/A |
II | Optic | Sensory | Vision | VEP |
III | Oculomotor | Motor | Eye movement, pupillary constriction | EMG from extraocular muscles (except trochlea and lateral rectus) |
IV | Trochlear | Motor | Eye movement | EMG from superior oblique |
V | Trigeminal | Both | Sensation to face, motor to muscles of mastication | EMG from masseter or temporalis |
VI | Abducens | Motor | Eye movement | EMG from lateral rectus |
VII | Facial | Both | Motor to muscles of facial expression, autonomic input to salivary glands, taste to anterior 2/3 of tongue | EMG from muscles of facial expression |
VIII | Vestibulocochlear | Sensory | Hearing and balance | BAEP |
IX | Glossopharyngeal | Both | Sensation to tonsils and pharynx, motor to stylopharyngeus, taste to posterior 2/3 of tongue, input to parotid gland
![]() Stay updated, free articles. Join our Telegram channel![]() Full access? Get Clinical Tree![]() ![]() ![]() |