Fig. 2.1
Retinal layers as they appear on spectral domain optical coherence tomography (Reprinted with permission from Staurenghi et al. [16])
Layer 1: Retinal Pigment Epithelium
Histologically, the retinal pigment epithelium (RPE) is composed of a single layer of low cuboidal epithelium. This layer has a neuroectodermal embryologic origin [3, 13, 14], with abundant melanosomes (melanin-containing granules) within the cells’ cytoplasm [15]. With OCT, the RPE layer appears hyperreflective [16].
The pigment epithelium forms the outer layer of the entire neural retina and extends from the optic disk forward through the ora serrata to be continuous with the epithelium of the ciliary body [2, 4]. There are about four million epithelial cells in each human retina [17]. The greatest density of these cells is in the fovea (5000/mm2) where these cells are also smaller and appear more condensed to each other. From the surface view of the retina, the retinal pigment epithelial cells appear tightly packed with regular hexagonal arrangement. This is due to the presence of tight junctions between cells. These tight junctions form a band that appears histologically as a membrane called Verhoeff’s membrane [18]. Their number is reduced with age, especially at the periphery where they reach 2000/mm2 in people above 40 years of age [19].
The basal surface of the RPE is separated from the underlying choroid capillaries by a membrane called Bruch’s membrane [20]. Bruch’s membrane is an elastic membrane that is formed of five layers: the choriocapillaris basement membrane, the outer collagenous layer, the central elastic layer, the inner collagenous layer, and the basement membrane of the RPE [5]. As the RPE, Bruch’s membrane also appears hyperreflective with the OCT. In low-resolution OCT, the RPE and Bruch’s membrane appear as one thick zone, but with high-resolution (spectral-domain) OCT, two hyperreflective zones separated by a thin hyporeflective band can be identified. As it appears on the OCT, the whole zone has been recently referred to as the RPE/Bruch’s complex (zone 14) (Fig. 2.1) [16].
The presence of the underlying Bruch’s membrane and the tightly packed epithelial cells create a barrier between the choriocapillaris and the outer retina. This barrier, usually referred to as the outer blood-retina barrier, works with the inner blood-retina barrier to control the microenvironment of the retina and to protect the retina from the immune system and systemic infections. These barriers are selective and allow for small molecules such as O2 and CO2 to diffuse freely [21].
The apical surface of the cuboidal epithelium is in contact with the photoreceptors (cone and rod processes) within the subretinal space. Here, the cuboidal epithelial cells possess microvilli that project in between processes of rods and cones [19]. The subretinal space is the extracellular space between the RPE microvilli and the photoreceptors. Every single epithelial cell faces 40 photoreceptors [19]. This arrangement of the RPE microvilli and the photoreceptor processes appears with retinal OCT as a hyperreflective zone on the apical side of the RPE. This has been believed to be the reflection of Verhoeff’s membrane, which represents the tight junction between RPE cells. It has been reported recently that this hyperreflective zone is in fact the outer tips of the photoreceptors with microvilli of the RPE [16, 22] and was named the interdigitation zone (zone 13) (Fig. 2.1) [22].
The connection between photoreceptors and microvilli in the interdigitation zone is important in maintaining integrity of the retina and also in the turnover process of rod and cone molecules. In fact, the granular appearance of the RPE cells is believed to be due to lipofuscin granules, which are produced from the phagocytosis of rod and cone debris into the cytoplasm of the pigment cells of this layer [23].
Retinal epithelial cells have high metabolic activity and that explains the abundance of mitochondria, endoplasmic reticulum, and free ribosomes [24, 25]. There appears to be active transport of molecules across the basal and apical surfaces of pigment epithelial cells with the outer choroid layer capillaries and the inner photoreceptor layer, respectively. For example, retinol is an essential vitamin for the visual cycle. This vitamin is absorbed from the choriocapillaris by the retinal epithelium and then supplied to the photoreceptors. The RPE is also supportive to the photoreceptors of the retina; loss of the epithelial layer usually results in atrophy of the photoreceptors [5, 25].
Another proposed function of the retinal epithelium is absorption of light that goes through the retinal layers and prevents it from being reflected back to the photosensitive cells, thus preventing photoreceptor cells from being restimulated. It is believed that this process helps in improving the image quality [25]. In addition, the layer also absorbs the energy from the reflected light and prevents it from overheating the eye. However, very strong light initially causes damage to this layer and consequently damage to the retina [26].
Layer 2: Photoreceptor Layer
This layer contains processes of cone and rod cells that receive light signals and convert them into electric signals. Each of these processes has an outer segment and inner segment. The plasma membrane of the outer segment is arranged into hundreds of flat disks [27]. The flat disks contain photopigments that are attached to their plasma membrane. Such arrangement increases the surface area of the plasma membrane and consequently the amount of photopigments. The lamellated disks with their photopigments are disposed between microvilli of the retinal pigment epithelium to form the interdigitation zone that has been described [16]. There are no junctional complexes between microvilli and the plasma membrane of the photoreceptor disks creating the subretinal space [28]. It is a loose potential space that is liable to become a true space under certain pathological conditions when the retina becomes detached from the underlying RPE [29].
The inner segment is divided into two parts: the outer zone is packed with mitochondria making it hyperreflective under OCT examination (ellipsoid zone), and the inner zone contains smooth and rough endoplasmic reticulum and appears hyporeflective with retinal OCT (myoid zone) (Fig. 2.1) [5, 27, 30].
Therefore, the photoreceptor layer appears as three distinct zones on the OCT imaging: a hyporeflective zone corresponds to the outer segment (zone 12), a hyperreflective zone corresponds to the ellipsoid zone of the inner segment (zone 11), and a hyporeflective zone corresponds to the myoid zone of the inner segment (zone 10) [16, 18].
Layer 3: External Limiting Membrane
The external limiting membrane appears on the OCT as a thin hyperreflective membrane that runs perpendicular to the direction of cell bodies of cone and rods and their process (zone 9) [16, 31, 32]. The inner segments of rod and cone cells pass through the external limiting membrane to reach their cell bodies in the next layer, which is the outer nuclear layer. It is not a true membrane; the intercellular junction between the processes of the cone and rods and Müller cells appears as a membrane on radial section of the retina [33, 34]. Integrity of this membrane is important in protection of photoreceptors [35], and in maintaining visual acuity in health and disease [36, 37].
Layer 4: Outer Nuclear Layer
The outer nuclear layer is formed of the cone and rod cell bodies containing their nuclei. These cells are the photosensitive cells of the retina. This layer is thickest at the macula and around the fovea centralis, where these cells form a multicellular layer [38].
Cone cells are primarily responsible for color vision with high resolution during daylight or under good lighting. The number of cones in the human retina is 4.6 million [39]. Cone cells are distributed in the entire retina but their concentration increases gradually toward the center of the retina to become highest in the area with the greatest visual acuity (the fovea), where the concentration reaches 199,000 cones/mm2. Cone concentration reduces gradually away from the fovea to become 2500 cells/mm2 near the ora serrata [38]. Also, cone density is higher in the nasal retina by 40–50 % compared to the temporal retina [39]. According to their lighting sensitivity, cone cells are classified into three types: blue, red, and green ones. The most common cells are the red cones forming 63 % of all cone cells, followed by the green cones (32 %) and the blue cone cells (5 %).
Rod cells are responsible for night vision as they are sensitive to a high range of illumination including light with low intensity. Their number is greater than cones, with a total number of 92 million. Rod density is variable throughout the retina. However, density reduces toward the center of the retina to become absent at the area of the fovea [38, 39]. The highest density of rods is a ring area 3–5 mm away from the fovea where their concentration may reach 150,000 cells/mm2. Concentration of rods also reduces to 30,000/mm2 toward the periphery of the retina [38].
Cells forming the outer nuclear layer, rods and cones, have a similar structure characterized by long cells with vertical orientation relative to the retinal layers. Each cell of the rods and cones has two processes that travel radially into two different directions. One of these processes is the photoreceptor portion that forms the previously described zones on OCT, which are myoid (zone 10), ellipsoid (zone 11), outer segment layer (zone 12), and part of interdigitation zone 13. The other process is the photoreceptor axons, which travel vertically to reach the outer plexiform layer forming a membrane called Henle’s fiber layer. The fiber layer of Henle is prominent at the area of the macula and can be recognized using OCT as a pale fibrous band apical to cone and rod cells [40, 41]. With OCT, the outer nuclear layer and Henle’s fiber layer appear as one hyporeflective zone (zone 8), where Henle’s fiber layer occupies the inner half and the rod and cone cell bodies occupy the outer half of the zone [16]. Thickness of this zone can be measured using OCT and frequently correlated to the degree of visual acuity in a number of conditions, especially at the area of the macula [42, 43]. At the macula, the zone is liable for extravasation of fluid and protein causing edema of the macula, which significantly affects visual acuity. Macular edema can be detected with OCT imaging and commonly seen in patients with diabetes [44] and as a side effect of fingolimod use in multiple sclerosis patients [45].
Layer 5: Outer Plexiform Layer
It is a complex layer of synapses between different processes of cone and rod cells and other interneurons. The photoreceptor axons end in a cone pedicles or rod spherules that have synaptic contacts with other cells in the outer plexiform layer, such as the bipolar and horizontal cells [30]. It is considered the initial step in the processing of light signals where the first synapse of these signals takes place [46]. This layer conducts signals from the outer nuclear layer to the inner nuclear layer [46, 47]. The layer contains cone pedicles and rod spherules synapsing on dendrites of bipolar cells, horizontal cells, and interplexiform cells, and the whole zone appears on OCT as a hyperreflective layer (zone 7) [16, 48, 49].
Layer 6: The Inner Nuclear Layer
This layer contains the cell bodies and nuclei of different types of interneurons: the horizontal cells, the bipolar cells, the amacrine cells, the Müller cells, and the interplexiform cells. These cells form complex connections via their processes in the inner and outer plexiform layers; it is considered the interconnection between the photoreceptors and the ganglion cells. The nearest cells to the outer plexiform layers are the horizontal cells. The amacrine cells are present near the inner plexiform layer, while other cells are located in between [50–52].
The layer appears with the OCT as a hyporeflective zone (zone 6) [16]. Its morphology can be affected by a number of diseases. However, there is no atrophy of the inner nuclear layer in multiple sclerosis or any other central pathology leading to retrograde axonal degeneration [53–55]. Focal thickening of the internuclear layer due to microcyst formation has been reported in MS and a large range of other neurological and ophthalmological diseases [56].
Bipolar Cells
The cell bodies and nuclei of bipolar cells are located in the inner nuclear layer. Each cell body has two processes: one is directed internally toward the inner plexiform layers and the other externally toward the outer plexiform layer. The externally directed process has dendrites that come in contact with rod spherules and cone pedicles and horizontal cell dendrites in the outer plexiform layer. Usually bipolar cell processes have more than one dendrite that is connected to a variable number of cells. Bipolar cells that contact cone pedicles are called cone bipolar cells, while those that contact rod spherules are called rod bipolar cells. Usually rod bipolar cells contact as many as 70 rod cells, whereas rod bipolar cells make contact with fewer cone cells [46, 57].
The internally directed process has dendrites that contact ganglion cell dendrites and amacrine cells in the inner plexiform layer. Simply, bipolar cells convert the analogue signals from photoreceptors to a digital signal, which is transmitted to the ganglion cells [57].
Cone bipolar cells are classified into three types: midget bipolar cells, blue bipolar cells, and diffuse bipolar cells. Midget bipolar cells are small cells that directly connect cone cells to ganglion cells; each midget cell connects one cone cell to a ganglion cell. Midget cells are believed to be responsible for high special resolution. Blue cells are larger than midget cells and they mediate transmission of short-wave signals. The diffuse cone bipolar cells are connected to several cone cells, up to ten cones, and they probably mediate luminosity rather than color. Rod bipolar cells receive signals from many rod cells and transmit the signal to ganglion cells indirectly by contacting dendrites of amacrine cells [46, 57, 58].
Horizontal Cells
Horizontal cell bodies and nuclei are located in the outer zone of the inner nuclear layer. Each cell has many dendrites directed toward the outer plexiform layer where these dendrites make synaptic contacts with rods and cones and with bipolar cells dendrites. These cells also have connections between each other in the outer plexiform layer via gap junctions at the tips of their dendrites. Horizontal cells are inhibitory to other cells in the outer plexiform layer. Inhibition is thought to be an important mechanism in improving contrast and spatial resolution by inhibiting illumination from the periphery of the visual field [51, 59, 60].
Amacrine Cells
Amacrine cell bodies are located either in the inner nuclear layer with other bipolar cells or in the ganglion cell layer where they are called displaced amacrine cells [61, 62]. Some amacrine cells have been described in between dendrites of the inner plexiform layer [61]. Amacrine cells have dendrites that are directed toward the inner plexiform layer and their input is thought to be inhibitory in nature [63]. These dendrites function also as axons and transmit signals in two directions. The amacrine cells form extensive connection with axons of bipolar cells and with dendrites, axons, and cell bodies of ganglion cells and also with other amacrine cells [46].
There are as many as 30 types of amacrine cells, but they are generally classified according to the degree of their dendritic connections within the inner plexiform layer [64]. The narrow-field amacrine cells, such as amacrine II cells, connect rod bipolar cells to ganglion cells and therefore may be involved in the scotopic vision (rod pathway), while large-field starburst amacrine cells are for motion detection and light adaptation during different times of the day (circadian rhythm) [59]. According to the inhibitory neurotransmitter released, there are different types of amacrine cells: GABAergic, glycinergic [65], or neither of them. Amacrine cells have been found to release dopamine [63] and acetylcholine in the retina [65, 66].
Layer 7: Inner Plexiform Layer
This layer is another processing layer of the retina that can be visualized using the OCT as a hyperreflective band (zone 5) [16]. The layer is divided into laminae, which can be up to six laminae with different connection levels. Three main laminae are usually described according to the type of contact: (1) the outer layer contains connection between bipolar cells, ganglion cells, and some amacrine cells; (2) the middle layer contains similar contact but with displaced amacrine cells; and (3) the inner layer contains synapses between bipolar and displaced amacrine cells [46, 47]. The layer contains synapses between dendrites of cells in the inner nuclear layer and the dendrites of ganglion cells. Therefore, this layer can be affected by pathologies of the surrounding layers. Thickness of the layer can be assessed by OCT [67] and can reflect pathological changes such as glaucoma [68, 69] and diabetes [70].
Layer 8: Ganglion Cell Layer
This layer contains the cell bodies and nuclei of mainly retinal ganglion cells and, less commonly, displaced amacrine cells in addition to few astrocytes. There are around one million ganglion cells in each retina, with more ganglion cells at the peripheral nasal retina compared to the peripheral temporal retina [71]. Ganglion cells are not present at the center of the fovea, but their number is greatest around the fovea where they form up to nine layers of ganglion cells. Ganglion cell density may reach 38,000/mm2 at the center of the retina [71]. However, there is only one layer of ganglion cells in most of the peripheral retina.
Ganglion cells are the final cells in the process of transmitting visual signals from the retina to the brain. They are multipolar cells with their dendrites directed toward the outer layers, while their axons form the nerve fiber layer. Dendrites of ganglion cells are connected to bipolar cells and amacrine cells in the inner plexiform layer. Each ganglion cell dendrites make indirect contact with a large number of other photoreceptor cells [46, 58].
There are more than 20 types of ganglion cells within the human retina. The two main types that form more than 80 % of the ganglion cells are the midget ganglion cells and the parasol ganglion cells [72]. Midget ganglion cells are small cells with a small number of dendrites. They receive signals from midget bipolar cells with a ratio of 1 to 1 at the fovea. This ratio increases as we move out from the fovea. In comparison, the parasol cells are larger with much wider dendritic connection. Axons of midget and parasol cells terminate at the lateral geniculate nucleus of the brain [73]. Another morphological and physiological classification of ganglion cells in vertebrates includes alpha, beta, and gamma ganglion cells. Alpha ganglion cells have large cell bodies, large axons, and large dendritic fields, while beta cells have smaller cell bodies, smaller axons, and smaller dendritic fields. Gamma cells are smaller heterogeneous cells that are distributed throughout the retina with low conduction velocity [74].
Ganglion cells can be stimulated directly by light without necessarily being stimulated by input from the photoreceptors. Ganglion cells contain photopigments called melanopins, which are present in a subset of ganglion cells called intrinsically photosensitive ganglion cells [75]. These cells form only 0.3 % of all ganglion cells in the retina and they combine direct stimulation by brightness of the surroundings with signals received from rod and cone cells. Axons of these ganglion cells project to the diencephalon and midbrain. These projections are believed to play a role in certain functions of the eye, such as the circadian rhythm and the papillary reflex. This may explain the preservation of the circadian rhythm, photosensitivity, and pupillary reflex in patients with severe loss of rod and cone photoreceptors [76].
The distance from the cell body of the retina to the brain requires an effective mechanism to transport metabolites and neurotransmitter vesicles to the axon terminals. Transportation of molecules travels through the optic disk and any interference of this transportation process beyond the optic disk usually results in optic disk swelling (papilloedema), which may lead to loss of optic nerve axons if left untreated (optic atrophy) [46].
The ganglion cell layer thickness (zone 4) can be assessed using OCT, especially at the macular area [16, 77]. The degree of atrophy in the layer correlates to clinical outcome measures of visual function in a number of diseases including multiple sclerosis [77], glaucoma [78], and optic neuritis [79].
For comparison, Table 2.1 summarizes the average size of retinal cells in relationship to the resolution of current OCT technology (3–7 μm) [25, 38, 80–83].
Table 2.1
Overview of the size of retinal cell soma in relationship to the 3–7 μm axial resolution of current clinical routine OCT technology
Cell | Diameter of the soma |
---|---|
RGC | 5–20 μm [80] |
Amacrine cell | 12–16 μm [81] |
Horizontal cell | 10 μm [82] |
Bipolar cell | 8 μm [83] |
Cone | 3.3 μm (center of retina) and 10 μm (periphery of the retina) [38] |
Rod | 3.0–5.5 μm [38] |
Pigment epithelium | Width 14 μm at the macula and 60 μm at the periphery (height is variable) [25] |
Layer 9: Nerve Fiber Layer
The nerve fiber layer is formed by the ganglion cell axons that travel horizontal to the retina layers. Axons converge within the layer from the entire retina to the area of the optic disk where other layers of the retina are diminished. The nerve fiber layer is thin at the periphery and becomes thicker as more fibers converge in the layer toward the optic disk [60]. Axons from the medial or nasal half of the retina usually converge radially to the area of the optic disk, which lies medial to the macula. Axons of ganglion cells of the macula, which is located just lateral to the optic disk, travel directly in a fasciculus called the papillomacular fasciculus or bundle to the optic disk. Axons from peripheral parts of the temporal retina pass above and below the macula to reach the optic disk. The axons of the papillomacular bundle (PMB) are smaller in diameter compared to axons elsewhere in the retina. The average axonal diameter of primates’ PMB, as demonstrated by electron microscopy studies, was 0.4 μm [84]. The small size of axons possibly makes the PMB particularly vulnerable, explaining the frequently seen temporal thinning of the nerve fiber layer in MS. Selective loss of small axons of the optic nerve in MS with the consequent degeneration of parvocellular cells has been reported previously. The study also demonstrated another evidence that the PMB projects mainly to the parvocellular cells [85].
Axons of the nerve fiber layer are unmyelinated as the refractile characteristic of myelin may interfere with vision. However, these axons are ensheathed by the surrounding processes of glial cells such as astrocytes and Müller cells, which are present in the nerve fiber layer [51, 86]. Few axons may possess myelin at the optic retina as a developmental anomaly, but most retinal axons acquire myelin sheath once they pass an area at the optic disk called lamina cribrosa [87].
The high reflectivity of the layer makes it apparent as a distinct zone even with low-resolution OCT (zone 3) [16, 88]. Therefore, the nerve fiber layer has been visualized and examined early during the development of OCT [88]. The nerve fiber layer is one of the important layers of the retina that can be affected by a wide spectrum of retinal diseases [89]. As the nerve fiber layer represents axons of the ganglion cells, these axons are affected greatly by the pathology of the ganglion cells. Thickness of the nerve fiber layer, especially around the optic nerve head (peripapillary), can reflect ganglion cell survival and consequently visual function [77].
Layer 10: Internal Limiting Membrane
The most interior layer of the retina is the internal limiting membrane. The membrane is formed by the expanded terminal end feet of Müller cells and astrocytes. The membrane continues anteriorly to the ora serrata and blends with the membrane that covers the inner surface of the ciliary body. Selective fluid exchange between the retina and the vitreous body takes place through the membrane. The membrane also controls migration of cells and other particles to the vitreous body [46].
Structure and thickness of the membrane vary at different parts of the retina. Thickness of the membrane is about 70 nm at the optic disk and the periphery of the retina, whereas thickness at the macula may reach 400–500 nm. The membrane is attached to the vitreous body of the eye by an inner meshwork of fibrils. The degree of attachment with the vitreous body also varies at different regions of the retina, being loose with few fibrils at the fovea centralis [90]. However, due to the connection between this membrane and the vitreous body, the retina is significantly affected by changes in the shape of the vitreous body.
The membrane appears hyperreflective on OCT [16] and it frequently cannot be differentiated from the underlying nerve fiber layers. Occasionally a hyporeflective narrow zone can be seen on normal OCT between the ILM and the vitreous called the preretinal space (zone 2) [16]. This space is a potential space for vitreous preretinal hemorrhage. Anterior to the preretinal space is the vitreous body, which is called posterior cortical vitreous near the center of the retina (zone 1) [16].
Retinal Glial Cells
The extensive distribution of neuroglia within layers of the retina fills the extracellular space and provides physical support to the retina. These cells appear to be important in maintaining the ionic environment around neural cells, glycogen storage, reuptake of released neurotransmitters, removal of debris, and insulating axons and neurons.
There are three types of retinal glial cells: radial glial cells that are also called Müller cells, astrocytes, and microglia. The most abundant cells in the retina among glial cells are Müller cells [91].
Müller cell bodies and nuclei are present in the inner nuclear layer. These cells extend radially and cross the layers of the retina from the most inner layer to the photoreceptive layer to form the cytoarchitectural support of the retina. The outer process extends to terminate as microvilli between the cone and rod processes. At this level, radial glial cells form the external limiting membrane, which is formed by dense zonula adherens. The inner process extends to the inner surface of the retina forming a flattened terminal foot plate. The terminal foot plate fuses with those foot plates from other Müller cells, and probably with astrocytes, forming the internal limiting membrane [46].
Müller cells are scattered between rods and cones and between other neuronal cells in the retina. They are also scattered between blood vessels and capillaries and cover them. Their processes send lateral lamellae forming a sheath-like structure that separates retinal cells except the areas of synapses. These lamellae contact blood vessels, especially capillaries, and their basal laminae fuse with those of the vascular smooth muscle in the media of vessels or of the endothelial lining of capillaries [46, 50, 92]. Müller cells are important in maintaining the extracellular environment necessary for the proper function of retinal neurons [16, 93].
Retinal astrocytes do not belong embryologically to the retina as they migrate along the optic nerve through the optic disk. This may explain the restricted position of these cells to the nerve fiber of the retina [94]. They are scattered in the entire retina except the fovea. These cells have similar morphology to astrocytes of the central nervous system in terms of flattened cell body with numerous processes and can be readily marked with astrocytes marker glial fibrillary acidic protein [95]. The astrocytes form extensive processes that ensheath axons of the ganglion cells and they also contribute in supporting retinal capillaries by forming glia limitans [92].
Microglia migrate to the retina from the circulation and distribute in small numbers in different parts of the neural retina. They are mainly present within the inner plexiform layer. They have multiple processes that extend horizontally to retinal layers. Their function in the retina is primarily similar to their function in the central nervous system as they are able to phagocytose debris [46].
The Macula Lutea
The macula lutea appears yellow in color and its diameter measures about 5 mm [96]. At the center of the macula is a reddish depression, of a diameter of 1.5 mm, called the fovea. The area of the macula is responsible for most of the photobic vision. The yellow color is due to the presence of the yellow carotenoid pigments known as xanthophylls [46]. The fovea appears shiny when examined with the ophthalmoscope (foveal reflex). It has the highest density of cone cells (199,000/mm2) [39], where cone cells are elongated with smaller diameter compared to cone cells in other parts of the retina. These are arranged in close proximity to each other and serve the function of the retina of obtaining high degree of spatial discrimination [39].
The previously described layers of the retina are modified in the macula, especially at the fovea. According to structure and modification of layers, four concentric zones can be recognized at the macula [43].
The perifoveal zone is the outermost part of the macula that delineates between the central retina and the peripheral retina. It is considered the transitional zone of retinal layer modification. The peripheral retina extends outside the perifovea and it is anatomically characterized by the presence of one layer of ganglion cells [97, 98]. At this zone, the ganglion cell layer starts to become multicellular. The perifovea is rich in blood vessels that start to diminish gradually when moving toward the central zones. In addition, the perifovea has a high rod-to-cone ratio of about 30–130:1. This ratio starts to reduce gradually toward the center [61].
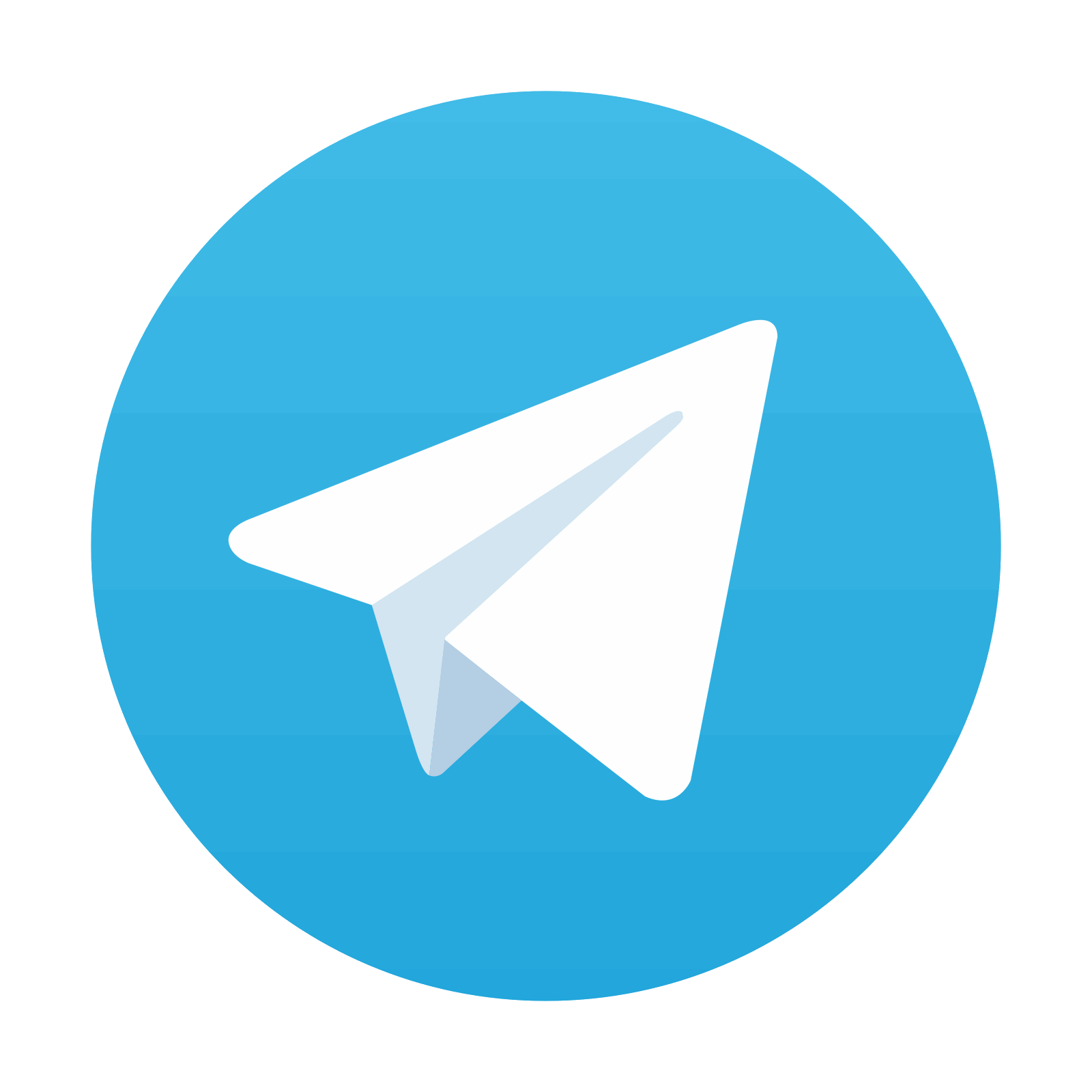
Stay updated, free articles. Join our Telegram channel

Full access? Get Clinical Tree
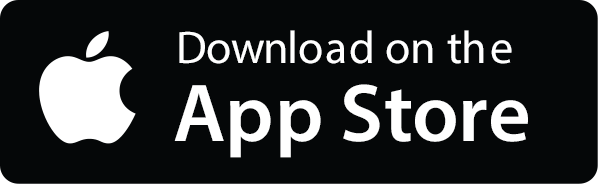
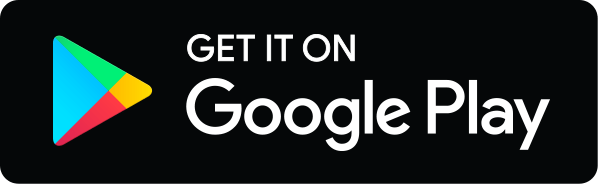