The NST is the first central relay for afferent inputs controlling cardiovascular functions, including the baroreflex and cardiac reflexes, respiratory and laryngeal reflexes [11], and gastrointestinal motility [9]. Due to its anatomical location, close to the floor of the fourth ventricle, the NST can be easily damaged during surgery over the floor of the fourth ventricle. Its bilateral lesion produces acute hypertension in rats [14], while in bigger animals this lesion can lead to systemic blood pressure (BP) lability, chronic hypertension, and exaggerated BP responses after environmental stress [35, 44].
The RVLM is considered the key area for BP regulation and it has been established as the major sympathetic pressor center, providing sympathetic tonic outflow activation to peripheral vessels of the skeletal muscles and visceral organs, as well as to the coronary vessels and cardiac muscle [6]. This nucleus receives, among others, inhibitory signals from the NST and CVLM and it is located in the ventrolateral medulla, at the entry zone of the IX and X cranial nerves [45]. RVLM lesions can lead to BP collapse and orthostatic hypotension [20]. RVLM chronic compression can result in chronic overstimulation of the sympathetic nervous system, leading to chronic arterial hypertension [25, 32].
The CVLM maintains tonic inhibitory control of the RVLM and mediates the sympathoinhibitory component of the arterial baroreflex. This nucleus is within the ventrolateral bulbo-medullary region and its lesion is related to hypertensive states, similar to those observed in NST lesions [10].
The bulbar region also contains two groups of parasympathetic preganglionic neurons: (1) the DMNV, located in the dorsomedial medulla, close to the NST, at the floor of the fourth ventricle; and (2) the nucleus ambiguus, located in the bulbo-medullary region with a ventrolateral location. The DMNV innervates the local ganglia in the respiratory tract, enteric nervous system, liver, and pancreas, and its lesion can lead to gastrointestinal dysmotility and other visceral disorders [37]. The nucleus ambiguus is the main origin of cardiomotor inhibitory output [27] and it also controls respiratory function. Unilateral lesion of this nucleus produces central hypoventilation, laryngeal stridor, impaired baroreflex cardioinhibition, and respiratory sinus arrhythmia [9].
The PB in the pons is a coordinating center. It receives visceral, thermoreceptive, and nociceptive inputs from the spinal cord and sends this information to the hypothalamus, amygdala, and thalamus [43]. The PB participates in the control of respiratory, cardiovascular, gastrointestinal, and micturition reflexes [13]. The PGM, in the midbrain, has a role in the integration of autonomic and somatic responses to stress, pain modulation, and other adaptive functions. It participates in cardiovascular responses associated with pain modulation, thermoregulation, coordination of micturition reflexes, and respiratory rhythm [9].
Autonomic Tests
The autonomic nervous system regulates vital homeostatic functions such as BP, heart rate, respiration, thermoregulation, gastrointestinal activity, and urinary bladder and sexual functions.
Recent advances have resulted in the development of new quantitative, non-invasive, and reproducible autonomic tests. The main goals of these tests are to easily evaluate the severity and the distribution of autonomic neuropathies, and to monitor their course and response to treatment.
There are tests that evaluate cardiovascular function and tests that quantify sudomotor function [31]. Cardiovascular tests can be divided into cardiovagal and adrenergic tests. The most commonly used cardiovagal tests are: quantification of the heart rate response to deep breathing and to the Valsalva maneuver. Adrenergic function can be measured by the following methods: (1) BP and heart rate responses to the Valsalva maneuver, (2) head-up tilt test, (3) plasma norepinephrine, and (4) quantitave cardiac uptake of MIBG (iodine-123 meta-iodobenzylguanidine).
Tests that analyze sudomotor function are: the quantitative sudomotor axon reflex test (QSART) and the thermoregulatory sweat test (TST) [31]. The QSART evaluates the integrity of the postganglionic sympathetic sudomotor axon and can define the distribution of sweat loss in the forearm, proximal leg, distal leg, and foot. The TST evaluates pre- and postganglionic thermoregulatory sympathetic pathways from the hypothalamus to the eccrine sweat glands. This test can detect patterns of anhydrosis.
Autonomic Dysfunction
Autonomic dysfunction can be classified, according to its extent, as generalized autonomic failure, selective or partial autonomic failure, distal neuropathies, and orthostatic intolerance syndromes [6].
Symptoms that suggest the existence of autonomic failure include: orthostatic hypotension (OH); digestive discomfort; gastrointestinal, sexual, and bladder dysfunction; increase or decrease of sweating; dry mucous membranes; and changes in skin temperature and color [36].
OH can explain some of the symptoms that neurosurgical patients develop before and after brainstem surgery. Light-headedness, blurred vision, cognitive difficulties, headaches, tremulousness, and palpitations can be related to OH.
As previously mentioned, autonomic nuclei can be damaged during brainstem surgery. Surgery over the floor of the fourth ventricle can easily injure the NST, which may elicit baroreflex failure. Baroreflex failure can lead to acute severe arterial hypertension, bradycardia, and cardiorespiratory arrest, as well as chronic cardiovascular instability and OH. If the injury is produced within the region of the ventrolateral surface of the pontomedullary junction, as in acoustic schwannoma surgery, the RVLM may be damaged and patients may develop severe postoperative OH. On the other hand, if the RVLM is stimulated by a pulsatile arterial loop or a neural structure, sympathetic hyperstimulation and subsequent chronic arterial hypertension may be elicited [26].
Which Neurosurgical Pathologies Can Affect CAN Structures?
Brainstem lesions associated with dysautonomia include tumors, multiple sclerosis [42], stroke [1, 7], syringobulbia [21], Chiari malformation [47], and vascular compression [15].
Tumors
The brainstem can be affected by intrinsic tumors (including cavernous malformations) and extrinsic compressing posterior fossa tumors.
The authors are currently carrying out a study of brainstem tumors (intrinsic and extrinsic); 43 patients have been studied. Intrinsic and extrinsic gliomas, ependymomas, medulloblastomas, cavernous malformations, epidermoid cysts, cerebellopontine angle schwannomas, choroid plexus papillomas, chondrosarcomas, pituitary adenomas, lymphomas, chordomas, arachnoid cysts, and meningiomas have been collected (Fig. 2).


Fig. 2
(a) Patient with a low-grade glioma compressing the dorsal surface of the mesencephalon, presenting orthostatic hypotension and postural orthostatic tachycardia; (b) patient with a bulbar intrinsic lesion presenting sympathetic hyperactivity, autonomic instability, orthostatic hypotension, and postural orthostatic tachycardia; (c) patient operated for a bulbo-medullary cavernous malformation, diagnosed with autonomic instability and orthostatic hypotension; (d) patient with a cerebello-pontine meningioma with ventrolateral pontine compression, presenting sympathetic instability and hyperactivity; (e) pediatric patient operated for a pilocytic astrocytoma with bulbar distortion, presenting arterial hypertension and orthostatic hypotension; (f) patient with an epidermoid cyst causing compression of all brainstem levels, presenting autonomic instability, sympathetic hyperactivity, and orthostatic hypotension
In Fig. 1 we show six cases of patients from our study with brainstem compression caused by tumors and presenting autonomic dysfunction. Relevant data were collected after our preliminary analysis: 92.9% of the patients tested showed some grade of autonomic dysfunction. The most frequent diagnoses were: autonomic instability (57.1%), sympathetic hyperactivity (35.7%), and OH (31%).
The first case of a brainstem tumor causing autonomic dysfunction was reported by Wagner et al., who described a tumor located in the floor of the fourth ventricle [22]. The first authors to describe the relation between the removal of tumors from the floor of the fourth ventricle and physiological abnormalities were Baker et al., in 1964 [2], who stated: “the floor of the fourth ventricle is considered no man’s land, and from an anatomical and physiological standpoint it is a very important region”. Baker and colleagues reported their experience with 11 subependymomas, which they classified into three types: (1) those that can be removed completely without any physiological changes, (2) others that cannot be approached because respiratory paralysis or vasomotor instability develops with manipulation, and (3) those that can be completely removed but with a residue of severe long-lasting physiological abnormalities. The autonomic abnormalities collected were: respiratory paralysis, OH, postural tachycardia, and digestive disorders.
Cameron and Doig described two patients with systemic arterial hypertension that improved after posterior fossa tumor surgical resection [8]. They included the posterior fossa tumors in the differential diagnosis of pheochromocytoma. Evans et al. published the first pediatric case of astrocytoma near the floor of the fourth ventricle, mimicking the features of pheochromocytoma. These clinical findings disappeared after tumor removal [16]. Cushing syndrome has also been included in the differential diagnosis of posterior fossa tumors [51].
Riedel et al. were the first to describe OH after the surgery of posterior fossa tumors: a foramen magnum meningioma and an ependymoma of the floor of the fourth ventricle [40]. Wörner et al. also showed another foramen magnum meningioma, with compression of the ventrolateral medulla oblongata, in which resection solved the chronic arterial hypertension [50].
A decade later, Hsu et al. [22], in 1984, published the first autonomic study in patients with a brainstem tumor. They described three patients with posterior fossa tumors and brainstem compression. They carried out an autonomic evaluation in one of the patients with a diagnosis of dorsal medulla oblongata astrocytoma with pontine and cervical medulla invasion. The study revealed OH caused by baroreflex dysfunction. Yamashita et al. described similar findings in autonomic tests in a patient with a diagnosis of bulbar lymphoma with OH [52]. These authors postulated damage of the NST or the DMNV as the origin of the dysfunction.
Rouchoux et al. reported, for the first time, the possible relation between hydrocephalus and brainstem lesions [41]. Subsequently, in 2010, Gomez-Esteban published a case of an epidermoid cyst associated with hydrocephalus that caused OH. The autonomic dysfunction was resolved after the placement of a ventriculoperitoneal shunt [19].
Monge Argilés et al. published a case-control study of 14 patients with brainstem lesions (ischemic strokes, vascular malformations, and neoplastic and post-traumatic lesions) and 25 control patients. The authors demonstrated a decrease in heart rate variability in the patients with brainstem lesions [32].
More recently, Idiaquez et al. described a woman with syncopal episodes with a diagnosis of a cavernous malformation of the dorsal and posterior medulla oblongata. After a partial resection of the tumor, the patient experienced a worsening of the OH. The authors proposed a lesion of the catecholaminergic transtegmental tract as the possible origin of the disease [24]. Ideguchi et al. reported a case of sympathetic hyperactivity with severe arterial hypertension and tachycardia that developed after surgery for a hemangioblastoma with bulbar and pontine compresssion. The authors postulated bilateral NST damage as the origin of the autonomic dysfunction [23].
From our preliminary study, we can report the case of a 9-year-old male patient with a recurrent fourth ventricle anaplastic ependymoma who developed severe arterial hypertension and BP lability during and after surgery. A punctual bilateral lesion located within the mid-dorsal medulla oblongata, caused by both infiltration and surgical resection, was observed on postoperative magnetic resonance imaging (MRI) (Fig. 3). On autonomic evaluation, we observed an increase in sympathetic outflow with tachycardia, together with OH caused by baroreceptor reflex dysfunction. We postulate that a bilateral injury to both nuclei of the solitary tract may have caused central dysautonomia.


Fig. 3
(a) Axial, sagittal, and coronal preoperative magnetic resonance imaging (MRI) views, showing new recurrence of anaplastic ependymoma with infiltration of the floor of the fourth ventricle. (b) Axial view of the upper medulla oblongata on anatomical atlas [12]. Black circle shows the possible location of the injury. (c) MRI showing postoperative distortion/injury of the medulla oblongata. Black and white arrows show the area of injury. This MRI was performed on the same day as the autonomic tests
Vascular Compression
In 1979, Jannetta et al. observed that brainstem compression caused by arterial loops could be responsible for some cases of essential neurogenic arterial hypertension [25]. The authors found that compression of the left ventrolateral surface of the medulla oblongata, specifically over the retro-olivary sulcus, close to the exit of the IX and X cranial nerves, may elicit activation of the RVLM, evoking a subsequent increase in sympathetic tone and BP elevation. The authors showed the results in five patients, with a significant improvement of the hypertension after vascular microdecompression surgery.
In the following years, several studies in animals concluded that chronic compression of RVLM elicits arterial hypertension [26, 33, 45]. The proposed theories for the arterial pulsatile beat effect on sympathetic pathways have focused on: (1) direct hyperstimulation of the RVLM and, (2) mechanical damage of the afferent pathways of the IX and X nerves that may cause deafferentation of the baroreceptor reflex arch with subsequent sympathetic hyperactivity due to RVLM liberation [34, 48].
Neuroimaging studies have revealed controversial results. Naraghi et al., in their study of 24 patients with a diagnosis of arterial hypertension, found 20 patients with vascular compression of the left RVLM [34]. Morimoto et al. observed that 78% of their hypertensive patients showed compression, compared with 34.5% of normal patients [33]. On the other hand, Watters did not find any statistically significant difference between cases and controls [49]. Finally, Levy et al. (2001), in their review, concluded: “current MRI technology is not adequate to image the microvasculature of the brainstem” [30].
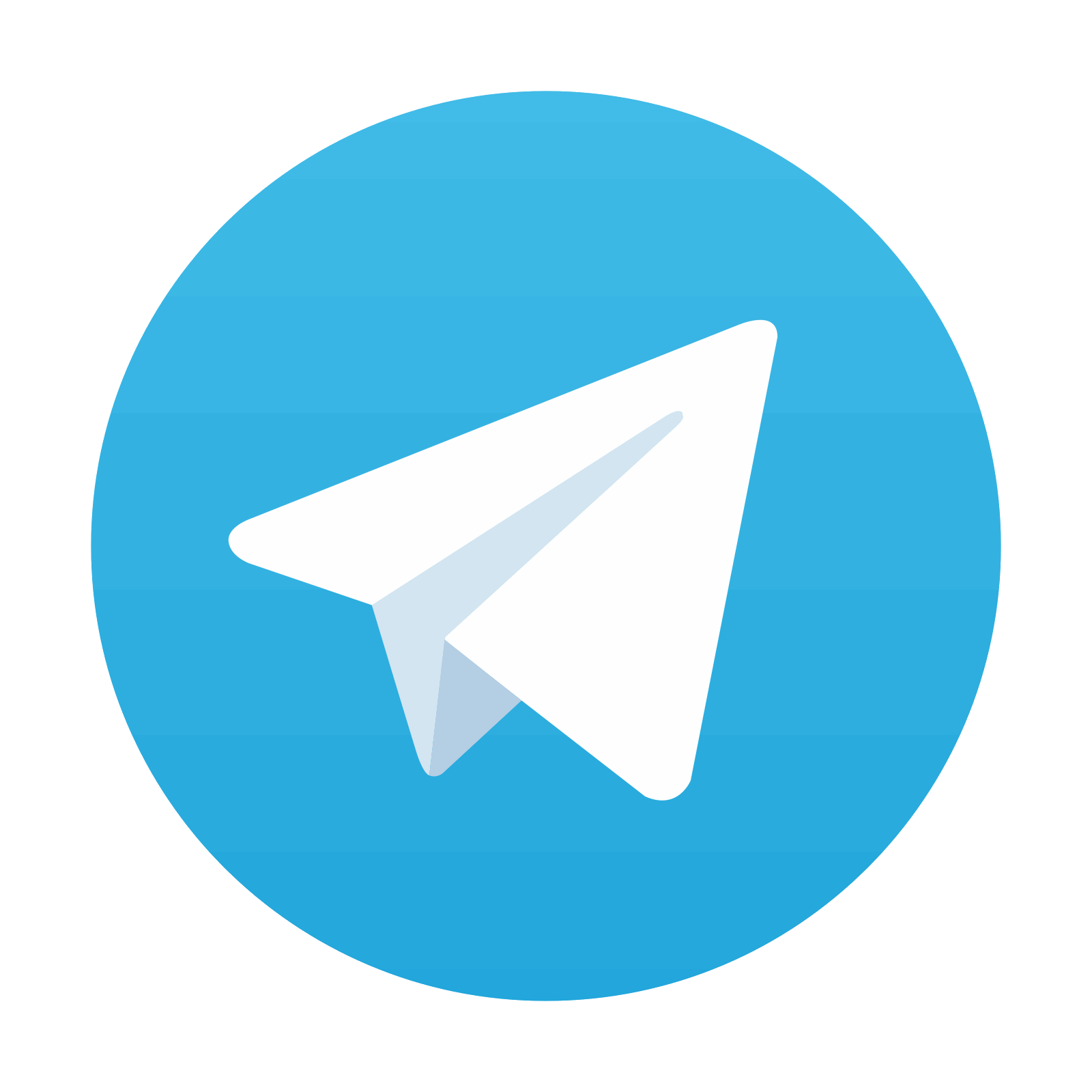
Stay updated, free articles. Join our Telegram channel

Full access? Get Clinical Tree
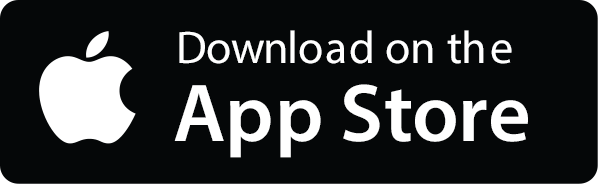
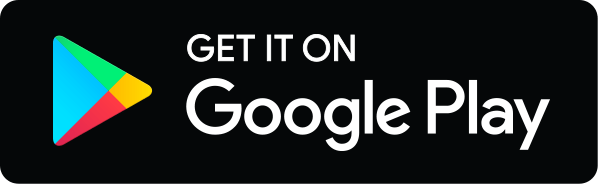