Mark Sherer and Angelle M. Sander (eds.)Clinical Handbooks in NeuropsychologyHandbook on the Neuropsychology of Traumatic Brain Injury201410.1007/978-1-4939-0784-7_2
© Springer Science+Business Media, LLC 2014
Cognitive and Behavioral Outcomes from Traumatic Brain Injury
(1)
Wayne State University School of Medicine, Rehabilitation Institute of Michigan, Detroit, MI, USA
Abstract
Outcome following traumatic brain injury (TBI) depends on many factors, including severity of injury. Research has demonstrated a dose–response relationship between TBI severity and cognitive outcomes, with more severe deficits associated with increasingly severe injuries. Additionally, changes in psychological functioning, behavior, and participation in the community may be sequelae of TBI. This chapter focuses on recovery and outcomes from TBI, including mild, mild-complicated, and moderate-to-severe, as well as mitigating factors, such as demographics and premorbid factors, psychological comorbidities, type of injury, repeated concussions, and financial incentives. Outcome prediction is essential for planning rehabilitation goals, patient and family education about long-term changes, and identifying necessity of future assistance. This task is made challenging by the additional influence of factors such as premorbid functioning, type and severity of injury, and other situational factors.
Keywords
OutcomesCognitionBehaviorPsychosocialParticipationMitigating factorsA variety of factors affect recovery from traumatic brain injury (TBI). The extent of cognitive recovery from TBI largely depends on the severity of the injury, as illustrated by the well-established dose–response relationship between TBI severity and cognitive outcome [1, 2]. This finding demonstrates that neuropsychological performance correlates with severity of injury with the most severe deficits following severe injuries. Furthermore, changes in behavior, psychological functioning, and participation in the community may also occur subsequent to TBI. This chapter will address recovery and outcomes following TBI, including mild, mild-complicated, and moderate-to-severe, as well as mitigating factors.
Mild TBI
Mild TBI (mTBI) has traditionally been defined by a Glasgow Coma Scale (GCS) score of 13–15, loss of consciousness (LOC) less than 30 min, and posttraumatic amnesia (PTA) less than 24 h [3]. Brief LOC may also be associated with mTBI; however, its usefulness in predicting symptoms subsequent to mTBI is questionable. Research has shown that LOC is not associated with total number of symptoms or duration of symptoms among athletes who sustained concussions [4]. Some persons report experiencing symptoms consistent with post-concussion syndrome (PCS), which can be grouped into three clusters: somatic, affective, and cognitive [5]. Somatic symptoms typically include headache, fatigue, and dizziness. The affective cluster often includes irritability, anxiety, depressed mood, and sleep difficulties. The most commonly reported cognitive changes include slowed processing speed, poor attention, and difficulty with short-term memory [5–7].
It is noteworthy, however, that PCS symptoms are not specific to mTBI [8, 9] and some individuals have questioned the construct validity of this diagnosis, including the authors of the Diagnostic and Statistical Manual of Mental Disorders—IV-Text Revision [10]. mTBI is listed as a diagnosis under the category of Criteria Sets and Axes Provided for Further Study, due to the limited empirical evidence for this disorder as a distinct entity. Furthermore, in prospective studies, the presence of mTBI did not predict PCS symptoms at 5 days post-injury, rather the presence of a depressive or anxiety disorder and female gender were most strongly related to PCS symptom reporting [6, 8, 11]. Rates of PCS symptoms have been found to be roughly equivalent among mTBI patients and trauma controls, which indicates that mTBI is not specifically predictive of PCS [12]. Other studies have found similar rates of PCS symptoms among normal controls and outpatients [13, 14]. Furthermore, PCS symptoms have not been found to be related to objectively measured cognitive functioning [12]. Rather subjective complaints of deteriorating cognitive functioning are likely related to non-brain injury factors [15, 16]. For studies that have noted PCS symptoms, symptoms resolved within 16 days of injury [4] and poor medical health, mental health, and marital status at baseline (being widowed, divorced, or separated) were more predictive of complaints such as fatigue at 12 months than was injury [17]. Persons with history of mTBI may attribute fatigue, as well as other PCS symptoms, to their injuries but the symptoms may actually be associated with premorbid factors, psychological status, or other health-related factors. Thus, PCS symptoms appear to reflect psychological distress related to the events of the injury, pre-injury psychological distress, or non-clinical factors and are likely unrelated to the actual head injury especially when they occur after the first month.
Mild cognitive deficits may occur within the first few days after mTBI, often including difficulty with recall, slowed processing speed, and reduced attention. These symptoms may be due to the actual brain injury, but other causes such as injury-related pain and psychological distress cannot be ruled out [8]. Research has suggested that athletes sustaining concussions demonstrate full recovery on cognitive measures within 7–10 days (e.g., return to personal baseline) [18, 19]. In community samples of persons with mTBI deficits in verbal learning and attention as compared to controls were evident up to 1–6 months post-injury in some individuals, but many individuals did not show any cognitive impairment during this time period [20, 21]. Although subtle neuropsychological changes may be evident following mTBI, research has shown that these changes are often resolved within 3 months [8, 19].
Regarding functional outcomes, assessed with the GOS, research has shown that persons who have sustained mTBI have good outcomes in both the short and long terms [8]. Poor outcomes and lingering PCS symptoms appear to be unrelated to brain injury and likely related to psychological variables or litigation. For example, subjective cognitive and somatic complaints may be related to depression or anxiety [8, 22]. The literature also indicates a tendency for persons sustaining injuries to recall themselves as being healthier and happier prior to the injury. For example, persons who had sustained concussions 6 months previously underestimated their pre-concussion symptoms by 97 % [23]. This tendency has been referred to as the “good old days” bias, such that people overestimate the actual degree of change that has occurred since injury by underestimating premorbid symptoms [24–26]. Iverson et al. [25] found that pre-injury ratings of persons with mTBI were higher than ratings of control participants. As PCS symptoms are nonspecific, a person may associate negative events and feelings to an injury rather than normal emotional fluctuations, events, etc. The effects of litigation on recovery from mTBI are discussed later in this chapter in the mitigating factors section. Thus, consistent findings indicate full recovery following an uncomplicated mTBI in the vast majority of injured persons.
Repeated mTBI
Research has also focused on the cumulative effects of sustaining repeated mTBIs, especially among athletes. Whereas some correlational studies have suggested a dose–response relationship between number of injuries and poorer neuropsychological status, a meta-analysis on multiple mTBIs by Belanger et al. [27] indicated that the literature revealed no overall significant effect on neurocognitive functioning or symptom complaints. Their results showed that sustaining multiple mTBIs has been associated with cognitive deficits in executive functioning and memory, but the effect size was small and the clinical significance was unclear. The authors concluded that sustaining two or more mTBIs has little relationship with cognitive performance several months later.
Some researchers have speculated that repeated trauma to the brain may accelerate degenerative processes later in life, such as mild cognitive impairment, Parkinson’s, Alzheimer’s, or some other type of neurodegenerative disease [28]. Findings concerning whether the length of recovery following subsequent mTBIs is longer than the first mTBI have been inconclusive [27, 28]. Among professional boxers, a review of the literature by Loosemore et al. [29] revealed that 10–20 % develop measurable long-term brain injury as indicated by clinical, radiologic, or histopathologic examination, but they found that amateur boxing was not associated with neurocognitive deterioration. A recent study on diffuse axonal injury (DAI) as measured by MRI showed no significant differences in frequencies of microhemorrhages between amateur boxers and controls [30]. A possible explanation for this discrepancy is that a head guard is not permitted in professional boxing, but is required in amateur boxing. Although the concepts of dementia pugilistica and chronic traumatic encephalopathy have been discussed in the media, there are very few well-controlled studies published in the neurology literature on these conditions and the studies have not controlled for other factors that could contribute to cognitive impairment such as premorbid learning disabilities, ADHD, and substance abuse.
The risk of “second-impact syndrome” has been identified, especially among athletes. This syndrome may occur when someone sustains a second concussion prior to complete healing from a previous concussion, usually within a few days to a week, which has been thought to lead to acute brain swelling and the possibility of fatality [19], but the probability of such an injury occurring is extremely low. Randolph and Kirkwood’s [31] review of the risks of sport-related concussion included an examination of the Catastrophic Sports Injury Database for 10 years of American football at all levels. These authors found that there was only one case of diffuse cerebral swelling at all levels of play over this time period, even though it was estimated that 40 % of players were returned to play when symptomatic [32]. Given the overall high rate of concussion, one would expect that if second impact syndrome was a common medical event, one would see more than one case in a 10-year span in this database. Additionally, given the return to play guidelines currently in place in the sports community, individuals are at a lower risk for this kind of event given that it already has a very low base rate. Research has indicated that when diffuse cerebral swelling is seen, it is most often in children and adolescents and represents a calcium subchannel mutation that is related to familial hemiplegic migraine [33, 34].
Complicated mTBI
Complicated mTBI is a classification of TBI for cases in which GCS scores were between 13 and 15 with evidence of an intracranial bleed or lesion [35, 36]. Research has shown that a subgroup of persons with GCS scores in this range accompanied by intracranial bleeds had poorer outcomes, including neuropsychological status, than those who had sustained an uncomplicated injury [35]. Similarly, persons with GCS of 15 after TBI who required surgery after developing intracranial hematoma were also at risk for worse outcomes, such that only 65 % had a good outcome [37]. Research has shown an increased level of disability among persons with complicated mTBI at 6 months and 1 year as compared to those sustaining uncomplicated injuries [35, 36, 38, 39]. Persons sustaining complicated mTBIs have also been found to have significantly worse cognitive performances than control participants at 1 month post-injury, whereas those with uncomplicated mTBI had performances comparable to controls [40]. Furthermore, based on group data, persons sustaining complicated mTBIs have not been found to experience complete recovery of cognitive function to unimpaired levels by 1 year post-injury, which contrasts the 3 month recovery of neuropsychological status that is typical with mTBI [36].
Outcomes at 1 year post-injury among persons with complicated mTBI are actually more consistent with the outcomes following moderate TBI [36]. Functional outcomes were equivalent for those with complicated mTBI and moderate TBI, including the following domains: physical (FIM motor domain scores), cognitive (FIM cognitive domain), independence, employability (e.g., Disability Rating Scale), and community integration (Community Integration Questionnaire (CIQ)). The most common areas of cognitive impairment were processing speed, learning and memory, and word generation [35, 36].
Kashluba et al. [36] highlighted the importance of these findings in understanding the limitations of exclusively relying on GCS scores in the classification of TBI severity, as many of these individuals have length of unconsciousness or length of posttraumatic confusion (PTC) that are more consistent with moderate injuries. Persons with similar GCS scores in the mild range can have strikingly different outcomes if the injury was further complicated by an intracranial bleed. The authors argue for greater consideration of additional factors beyond GCS to predict outcome and determine severity of injury, including LOC, length of PTA, and time to follow commands. As the complicated mTBI group has been found to have similar outcomes to those sustaining moderate TBIs, more information regarding their outcomes is presented in the moderate-severe section of this chapter.
Moderate-Severe TBI
Moderate to severe TBI has typically been defined as a LOC longer than 30 min, PTA longer than 24 h, or a GCS of 9–12 for moderate severity and 3–8 for severe severity [3]. Following injury, persons with severe TBI may proceed through a series of stages, including coma, vegetative state, minimally conscious state, confused state (PTA), and recovery. While all will, by definition, have some period of coma (typically described as a GCS of 8 or below), most will never be in a vegetative state and it is unknown how many will be in a minimally conscious state at some point during recovery. For patients who are in vegetative and/or minimally conscious states, the duration of these states varies greatly [41]. Many people with moderate TBI, however, may never have been in a coma and none are in coma at hospital admission. Vegetative and minimally conscious states are not seen in moderate TBI unless there is deterioration due to some late occurring complication such as intracranial bleeding. However the confused state is seen in all patients with moderate or severe TBI. Incidence of persistent vegetative state (complete unawareness of self and environment lasting longer than 30 days with some preserved brainstem functioning such as eye opening) at 1 year post-injury is rare (e.g., 1 %; [41]). During a minimally conscious state, a person demonstrates inconsistent awareness of the environment and is inconsistent in following commands. The minimally conscious state is typically a temporary phase of recovery [42]. Subsequently, persons with TBI become responsive but confused. They often experience retrograde amnesia, such that they cannot recall events for a period of time prior to the head injury. In severe injuries, the last memory that can be recalled may have occurred days, months, or even longer before the injury. As recovery proceeds, the extent of retrograde amnesia typically shortens but the events surrounding the injury are not typically fully recalled. PTA is memory loss for events following the injury, including time in coma [43]. As other neurobehavioral impairments (e.g., disrupted sleep-wake cycle, disturbed consciousness, altered psychomotor activity, agitation) often accompany the memory and cognitive impairments seen in PTA, the broader term PTC is now often used to describe the early period of recovery following TBI [44]. PTC may be brief, such as several days, or long-lasting, such as longer than several weeks. PTA/PTC is typically considered to be resolved when a person demonstrates orientation and the ability to create and retain new memories [42]. PTA duration is best determined by serial administration of an orientation measure such as the Orientation Log or the Galveston Orientation and Amnesia Test. Agitated or restless behaviors during PTC have been associated with better outcomes than initial sluggishness or immobility [45]. Sherer et al. [46] found that patients who were more severely confused had worse outcomes in employability and productivity.
Neurobehavioral symptoms are common among those with moderate to severe TBI and typically include problems with irritability, temper, dizziness, sensitivity to noise, and blurred vision. Additionally, they may experience apathy or lack of initiative, as well as extreme fatigue or tiring easily [43]. The most common cognitive deficits following moderate to severe TBI include impairments in attention, processing speed, and learning and memory. Attentional difficulties can include distractibility, poor concentration, and reduced divided attention. Information processing speed is reduced, which can affect numerous other cognitive domains. Complex attention and executive functioning have frequently been found to be impaired following moderate to severe TBI [47, 48]. Some have argued that these deficits are the consequence of deficits in processing speed and attention [49]. Executive dysfunction may present as poor self-awareness, insight, or self-control, as well as impairments in organization, planning, and judgment. Memory problems typically consist of difficulties with learning new information, retaining it, and subsequently retrieving it. These difficulties may also be due to impairments in attention and executive functioning. Deficits in language and visuospatial constructional skills may also be present in more severe injuries [1]. Classic aphasia is relatively rare following TBI, unless there is a focal lesion [50]. Communication problems may be present due to word finding problems, organization, and executive functioning (e.g., social judgment, impulsivity). At 1 month post-injury, persons with moderate or severe TBI performed significantly worse on all cognitive measures than did controls, and those with severe injuries continued to perform worse than controls at 1 year post-injury [51].
Significant, long-term deficits are consistently found among persons with moderate to severe TBI. Moderate TBI appears to be associated with cognitive deficits for 6 months or longer post-injury; it is noteworthy that in a meta-analysis on cognitive outcome, Dikmen et al. [1] indicated that due to inconsistent use of cut points in severity labels, findings had to be tempered accordingly. Consistent with the dose–response theory, severe TBI is often associated with more extensive cognitive deficits than moderate TBI. For example, Novack et al. [52] found that the severely injured group had worse cognitive outcomes than did the moderately injured group at 12 months post-injury, and both groups were below normative cognitive performance levels.
Research on the timeline of cognitive recovery from moderate to severe TBI has generally indicated that the most rapid recovery occurs during the first 5 months post-injury [53]. Recovery typically continues between 6 months to 2 years post-injury [54] with the steepest rates of improvement between 2 and 5 months [53]. Millis et al. [55] found that further improvement can still be evident at 5 years post-injury. Rate and extent of recovery varies across cognitive domains, such that there is slower improvement for more complex functioning [56]. Consistent with this, Millis et al. [55] found that a greater proportion of persons with TBI demonstrated improvement on simpler memory tasks than on complex memory tasks. Recovery of reasoning and problem-solving skills was also variable, such that some people demonstrate sparing or improvement and others exhibit marked impairment up to several years post-injury. Their results also showed that the greatest improvements during years 1–5 post-injury occurred on measures of visuoconstruction (Block Design), problem-solving (Wisconsin Card Sorting Test), and complex attention (Trails B). Motor and visuospatial domains continue to show steep improvement throughout the first year post-injury [53]. Millis et al. [55] reported that tasks that require complex constructional skills and speed may be the most sensitive to late recovery (e.g., 1–5 years post-injury).
Residual cognitive deficits are common 2 years post-injury [54] and differences between persons with TBI and controls in attention, processing speed, memory, and executive functioning have been found up to 10 years post-injury [57]. Millis et al. [55] found that at 5 years post-injury (complicated mild to severe) cognitive functioning ranged from no measurable impairment to severe impairment. The course of cognitive recovery varies greatly among individuals. Millis et al. [55] found that 22.2 % of persons in the Traumatic Brain Injury Model Systems (TBIMS) national database demonstrated cognitive improvement during 1–5 years post-injury, while 62.6 % remained stable and 15.2 % deteriorated. The further decline of cognitive functioning during the recovery stage of TBI has also been reported by Till et al. [58]. Millis et al. [55] noted that the subgroup showing continued decline in cognitive functioning was the oldest group (e.g., middle age), with the next lower age group remaining stable, and the youngest group showing cognitive improvement. The declines were most evident on measures requiring cognitive flexibility and speeded performance and were of a great enough magnitude to be associated with functional declines. Bercaw et al. [59] suggested that possible reasons for this decline include medical comorbidities or emotional distress.
Cognitive performance measured during acute rehabilitation is useful in predicting 1-year outcomes, even above and beyond functional and injury severity factors. For example, neuropsychological status within the first month post-injury assessed during inpatient rehabilitation with a brief standard battery was predictive of handicap, functional outcomes, need for supervision, and employability at 1 year post-injury. An estimate of premorbid cognitive functioning (WTAR) was most predictive of outcomes at 1 year post-injury individually. A measure of executive functioning (TMT-B) also showed unique predictive power of functional independence (FIM) and general outcomes (GOS-E) [60].
Productivity Outcomes
Moderate to severe TBI has been associated with significant functional impairments at 3–5 years post-injury. Specifically, Dikmen et al. [61] noted that 60 % of their sample of persons with TBI reported having long-lasting cognitive difficulties that affected their abilities to complete daily tasks independently and 30 % were unable to return to school or work. Bercaw et al. [59] found that improvements in processing speed and learning were predictive of ratings of disability and functional independence at 2 years post-injury, even after controlling for effects of age and injury severity.
Cognitive and behavioral sequelae after TBI can interfere with returning to or gaining employment. Estimates for the rate of unemployment following TBI range from 60 to 80 % [62, 63]. A strong dose–response relationship has been shown between severity of TBI and return to work rates, with lower rates of return for more severe injuries [64, 65]. The early indicators of TBI severity (e.g., GCS, length of PTA, time to follow commands) and functional indicators of injury severity (e.g., measures of disability and handicap such as DRS or FIM) have been associated with rates of returning to work at 1 year post-injury [66].
Doctor et al. [67] reported unemployment rates at 1 year post-injury of 31 % among persons with mTBI, 46.4 % among those with moderate TBI, and 62.1 % among those with severe TBI, all of whom were working prior to their injuries. These rates were significantly higher than those of the general population, ranging from 8 to 10 %. Dikmen et al. [21] had similar findings, such that persons with more severe TBIs had lower return rates than those with mild or moderate injuries. Additionally, they reported that persons with TBI had a lower return to work rate than trauma controls (49 %vs. 63 %) at 1 year post-injury. The greatest rate of returning to work post-TBI occurs between 1 and 6 months post-injury; however, those with more severe TBIs may continue to return to work over a longer period of time as compared to those with mTBIs who reach an asymptote earlier. It is noteworthy that only a very small percentage of those with the most severe TBIs return to work within 2 years of injury [64].
Neuropsychological status is also an important factor in returning to work. Dikmen et al. [64] found that neuropsychological functioning at 1 month post-injury was associated with employment rates. Specifically, 96 % of those with “excellent” cognitive abilities were working; these people likely had milder injuries and good premorbid functioning. Intact executive functioning, attention, and memory skills are critical for adequate performance and job success. In a meta-analysis of the predictive power of neuropsychological assessment in vocational outcomes, Crepeau and Scherzer [68] found that executive functioning was highly correlated with employment outcomes and measures of language, visuospatial abilities, and global cognitive status was moderately correlated with employment. Cifu et al. [66] reported that neuropsychological assessment occurring in the acute phase of rehabilitation was not predictive of employment outcomes at 1 year. They hypothesized that this finding may be related to the fact that a minimum threshold of functioning must be present to complete neuropsychological testing, which reduces the range of assessment by removing the most severe injuries from the analyses. They also suggested that the specific domains of neuropsychological assessment may not adequately map onto functional skills and abilities; whereas, cognitive assessment on a more functional measure (e.g., DRS) was predictive of return to work. Sherer et al. [69], however, found that early cognitive assessment was predictive of later employment outcomes. This review study also indicated inconclusive findings regarding the relationship between late or concurrent neuropsychological assessment with employment outcomes. Consistent with this, Putnam and Fichtenberg [70] indicated that the predictive power of neuropsychological assessment attenuates as time increases between time of injury and time of neuropsychological testing. They argued that comprehensive assessments, especially approximately 1 month post-injury, are more informative than later assessments.
Demographic variables have also been associated with rates of returning to work post-TBI. Dikmen et al. [64] found that age, education, and pre-injury work history were predictive of post-injury employment. Specifically, they found that persons over age 50, persons with less than a high school education, and those with unstable work histories were less likely to return to work and if they did return, the time to do so was longer. Having no history of alcohol and drug abuse or premorbid psychiatric diagnosis has also been associated with more productive activity at 1 year post-injury [71]. Lower scores on measures of productivity in community integration have been associated with older age, premorbid behavioral problems, less education, and being unmarried at the time of the injury [72].
Unfortunately, the literature on return to work often consists exclusively of measurement of employment status, without further assessment of other job-related variables. Cifu et al. [66] argued that a measurement of percentage of employment among persons with TBI at various time points post-injury provides a distorted picture of the overall adjustment to employment among this group. The literature is lacking in description of job retention and changes in level of employment (e.g., less complex tasks, fewer responsibilities, fewer hours) [65]. However, several studies have looked at job stability rates. For example, Machamer et al. [73] found that premorbid characteristics, including extent of pre-injury work history and earnings, and lower neuropsychological functioning post-injury were significantly related to difficulty maintaining stable employment 3–5 years post-injury. In their study, 46 % of those who returned to work maintained stable, uninterrupted employment. Kreutzer et al. [74] also indicated relationships between demographic variables and stable employment after TBI, including minority status, marital status, and educational background. They also noted that driving independence was strongly related to post-injury work stability.
Independent Living and Community Integration Outcomes
Dikmen et al. [75] reported that 76 % of persons with TBI returned to independent living, in contrast to 93 % of trauma controls at 1 year post-injury. Again, increased injury severity (as measured by length of coma) was associated with reduced likelihood of returning to independent living; specifically, the rates were 89 % for less than 1 h and 1–24 h of coma, 74 % for 1–6 days, 49 % for 7–13 days, 55 % for 14–28 days, and 23 % for more than 29 days. Temkin et al. [65] noted that many persons with TBI who had previously lived independently resided with their parents for the first few years post-injury. Hart et al. [76] reported that neuropsychological assessment, particularly of executive functioning, was predictive of need for supervision after TBI.
Social functioning and community integration are also often affected by moderate to severe TBI. Problems in these areas have been reported to be greater than those in basic ADLs at 1 year post-injury and also exhibit a dose–response relationship with injury severity [65, 77]. Participation in leisure activities also appears to be reduced among those with moderate to severe TBI, even many years post-injury [65]. Relative stability of social functioning, albeit lower than community norms, has been reported for at least 5 years postrehabilitation. Evidence for some improvement in the subdomains of home integration and occupation was reported [78]. Female gender tended to predict better outcomes in community integration overall and specifically in home integration [72].
Life Satisfaction
Reductions in life satisfaction and quality of life post-TBI have also been reported [65]. A study examining changes in life satisfaction over time following TBI showed that a decline in the second year postdischarge was followed by steady improvement. The authors reported that this pattern could represent a “honeymoon period” in which persons with TBI do not fully appreciate the consequences of their injuries until sometime after discharge and then satisfaction increases as people begin to adapt and use accommodations for long-lasting sequelae [78]. Those who have better awareness of their deficits secondary to TBI have been found to report less life satisfaction as compared to persons with impaired awareness of deficits [79]. Unlike other domains described in this section, life satisfaction does not appear to have a strong dose–response relationship with injury severity, with research showing either no effect [80] or increased life satisfaction with greater severity of injury [81, 82]. Pierce and Hanks [83] reported that this counterintuitive finding that enhanced life satisfaction follows more severe injuries may be explained by oversensitivity to symptoms seen in persons with mild injuries. They found that the strongest predictor of life satisfaction was level of participation or extent of involvement in activities; execution and ability to do various activities was also predictive of satisfaction but to a lesser extent.
Psychological Sequelae of TBI
TBI has been associated with increased risk of psychological disorders, both organically and situationally, with regard to reactions to injury and disability. This section primarily focuses on organically based changes which may occur following moderate to severe TBI, with some highlights of other causes and rates of disorders. Possible biological causes for psychological changes following TBI include the possibility that hypoxia leads to the release of free radicals and excitotoxic neurotransmitters. Additionally, neurochemical changes can occur subsequent to TBI, including effects on norepinephrine, serotonin, dopamine, and acetylcholine [84]. Anxiety and depression have been shown to have the strongest association with TBI and are perhaps the most commonly studied psychological changes. A meta-analysis by van Reekum et al. [85] revealed that the highest relative risks (RR) following TBI were for major depression (RR 7.5), bipolar disorder (RR 5.3), anxiety disorders (RR 2.0), and panic disorder (RR 5.8). Interestingly, although there are many pharmacologic approaches to dealing with these disorders in the medical and psychiatric literature, there are no approved medications for treating these disorders in those with TBI and medications are used off-label. For a review see Waldron-Perrine, Hanks, and Perrine [86].
Mood Disorders
Depression. Research has shown compelling evidence for TBI causing major depression [85]. Estimates for the occurrence of depression during the first year post moderate to severe TBI range from 14 to 42 %; rates for future time points, up to 50 years post-injury, were 11–61 %. The rates of moderate to severe depression as measured by the Center for Epidemiological Studies Depression Scale (CES-D) following complicated mild to severe TBI ranged from 31 % at 1 month to 17 % at 3–5 years post-injury [87]. Major Depression occurred in 44.3 % of persons with TBI in the time up to 7.5 years post-injury [85]. These estimates are much higher than the lifetime prevalence of depression in the general community [88]. Dikmen et al. [87] suggest that the elevated rates of depression post-TBI cannot be fully explained by the presence of somatic symptoms associated with brain injury, as depressed affect and lack of positive affect are also increased in persons with TBI.
The development of depression post-TBI is secondary to numerous factors, including premorbid personality, psychiatric history, social support, reaction to injury and disability, and organic changes [85]. Dikmen et al. [87] reported that the rates of depression were largely unassociated with severity of the brain injury (e.g., complicated mild, moderate, and severe). Increased depressive symptoms have, however, also been reported to be associated with less severe TBIs [85, 87]. Dikmen et al. [87] explain that the literature assessing the relationship between TBI severity and depression is mixed, likely because the relationship is complex and mediated by many factors, including awareness of deficits, injury-related disabilities, etc. Symptoms of depression may increase as a person becomes more aware of his or her deficits. Dikmen et al. [87] also found that premorbid factors, such as unstable work history, less than high school education, and alcohol abuse, predicted the presence of depression after TBI. Premorbid psychiatric disorders may also contribute to post-TBI depression [89]. Research has generated mixed findings for gender differences in the development of depression post-TBI, several showing no relationship [90–92] and some showing an increased risk for females [93, 94].
Robinson and Jorge [89] argue that although depression may be related to changes in social functioning, it is “not simply a psychological response to the severity of physical or intellectual impairment” (p. 237). Lesion location(s) and changes in neurotransmitters have been considered organic causes of depression post-TBI of moderate to severe severity [85, 95–97]. Associations between lesions in the left dorsolateral frontal cortex, left basal ganglia, and to a lesser extent, right hemisphere and depression have been reported [78, 97, 98]. Depressive symptoms post-TBI may also be related to hypometabolism in the lateral and dorsal frontal cortex, specifically the cingulate gyrus, and increased activation in the ventral limbic structures, such as the amygdala, medial thalamus, and prelimbic cortex [99–101].
Although researchers have noted that our understanding of the neurobiological underpinnings of depression is limited, Rosenthal et al. [102] hypothesized that lesions/contusions to the frontal pole where noradrenergic and serotonergic projections enter the cortex could greatly disrupt cortical aminergic functions, possibly leading to depression. Kelly et al. [103] found that 18 % of persons with TBI had growth hormone deficiency/insufficiency at 6–9 months post-injury and also were 3.7 times more likely to report depression, suggesting a possible relationship between hormone changes and depression subsequent to TBI.
Bipolar disorder. Bipolar affective disorder was present in 4.2 % of persons with TBI over 7.5 years post-injury in the van Reekum et al. [85] meta-analysis, which is higher than the general lifetime prevalence rate of 0.8 % [88]. Males were found to have an increased likelihood of bipolar or cyclothymia in a small sample size (e.g., 1 of 10 females and 4 of 8 males; [94]). Shukla et al. [104] found that 50 % of persons developing mania post-TBI also had seizures, suggesting a possible link of mania secondary to seizure. Pope et al. [105] reported that persons with mania post-TBI responded preferentially to valproate rather than the typical drug of choice, lithium, which further supports a seizure hypothesis. Mania may also be associated with right hemisphere lesions, limbic system lesions [106], and temporal basal polar lesions [91]. Jorge et al. [91] reported that mania has not been associated with severity of TBI, level of cognitive, physical, or social impairments, or personal/familial history of psychiatric disorder.
Anxiety
Generalized anxiety disorder (GAD). In a meta-analysis, van Reekum et al. [85] reported that 9.1 % of persons with TBI had GAD over the 7.5 years post-injury. This is approximately two times the community prevalence rates. GAD is often comorbid with depression following TBI, just as it is in community samples [94]. Comorbid anxiety and depression were associated with a longer duration of mood disturbance as compared to non-anxious, depressed persons with TBI. Research has been mixed as to the association between severity of injury and risk of GAD. Epstein and Ursano [107] described the interrelationships between anxiety and TBI as multifactorial, and at that time the relationship to specific tissue damage was unclear; however, some studies have pointed to certain typical lesion occasions. Jorge et al. [91] reported that right hemisphere lesions were associated with anxious depression, and left anterior lesions were associated with major depression without anxiety. Right orbito-frontal lesions have also been associated with anxiety and depression [108].
Posttraumatic stress disorder (PTSD). PTSD was reported to occur in 14.1 % of a large sample in van Reekum’s [85] meta-analysis over 7.5 years post-TBI with a relative risk of 1.8. PTSD appears to be more common in persons with less severe TBI, as it is most likely that those with mild injuries have memories related to their accident (e.g., motor vehicle accident, assault) whereas those with severe injuries are less likely to have any recall of these stressful events secondary to LOC or amnesia [109]. Among persons with mTBI who experienced acute stress disorder at 1 month post-injury, 82 % later developed PTSD, whereas it only occurred in 11 % of persons with mTBI who did not have acute stress disorder [110]. Many persons who experience PTSD post-TBI have also suffered other physical traumas, which can also cause or contribute to PTSD [111]. In a small sample, Ohrey et al. [112] found higher rates of PTSD among females post-TBI.
Panic disorder. van Reekum et al. [85] reported that 9.2 % of persons with TBI had panic disorder over the 7.5 years post-injury in a meta-analysis, which has a relative risk of approximately 5.8 as compared to the community prevalence rates. Typical neuroanatomical areas that have been implicated in panic attacks include orbitofrontal, cingulate, and medial temporal cortical areas, which are also frequent locations for TBI-related lesions [111]. Yet, as van Reekum et al. [85] notes, there is no available data at this point to support a pathophysiologic hypothesis of panic disorder in TBI.
Obsessive compulsive disorder (OCD). With regard to obsessive and compulsive symptoms, van Reekum [85] noted in the meta-analysis of articles spanning a 7.5 year period following TBI, 6.4 % of people had OCD, which indicates a relative risk of 2.6 as compared to a community sample. It was thought that OCD associated with TBI may be relatively brief and could be very effectively treated with antidepressants, as compared to the generally more chronic OCD [111, 113]. Based on available research, Kant et al. [114] have concluded that there is a possible causative involvement of the frontal systems impairment in developing OCD post-TBI. Drummond and Gravestock [115] have also suggested that the limbic system, which has been implicated as a checking system, may become oversensitive post-TBI. The organic relationship between TBI and OCD is yet unclear, but areas that may be implicated include damage to the right orbital cortex, right orbitofrontal cortex, frontal cortex, occipital cortex, basal ganglia (especially caudate), and the limbic system [111].
Personality Disorders
Several studies have reported an increase in rates of personality disorders following TBI [85, 116]. Koponen et al. [116] reported that roughly one third of persons with TBI in their sample had personality disorders, noting that personality disorders are also prevalent in persons prone to TBI and may reflect premorbid factors rather than post-injury conditions exclusively. The specific diagnoses reported vary by research study, including avoidant, borderline, narcissistic [94], antisocial, and obsessive-compulsive [116]. Such authors have noted that changes in personality are likely more common than developing symptoms consistent with an identified personality disorder and that these changes are associated with damage or changes to subcortical and frontal systems [85]. Moreover, if one looks at the nature of the behavioral disturbances after moderate to severe TBI, the clinical presentation is more reflective of neurobehavioral disturbance rather than true personality change or personality disorder.
Schizophrenia and Psychosis
Schizophrenia has not been found to be strongly associated with TBI, with a minor, if any, relative risk of schizophrenia post-TBI, 0.5 as compared to community rates of 0.7 % [85]. Several authors have concluded that there is no evidence and that it is very unlikely that TBI causes or increases the risk of schizophrenia [85, 117, 118]. Mixed evidence has been reported regarding a relationship between TBI before age 10 and increased risk of schizophrenia and/or earlier age of onset [117, 119, 120]. Kim [118] explained that the research evidence provides contradictory results concerning a possible stress-diathesis model in which TBI may affect the risk of expression of schizophrenia in persons at risk for the disorder, highlighting the need for additional research to be conducted.
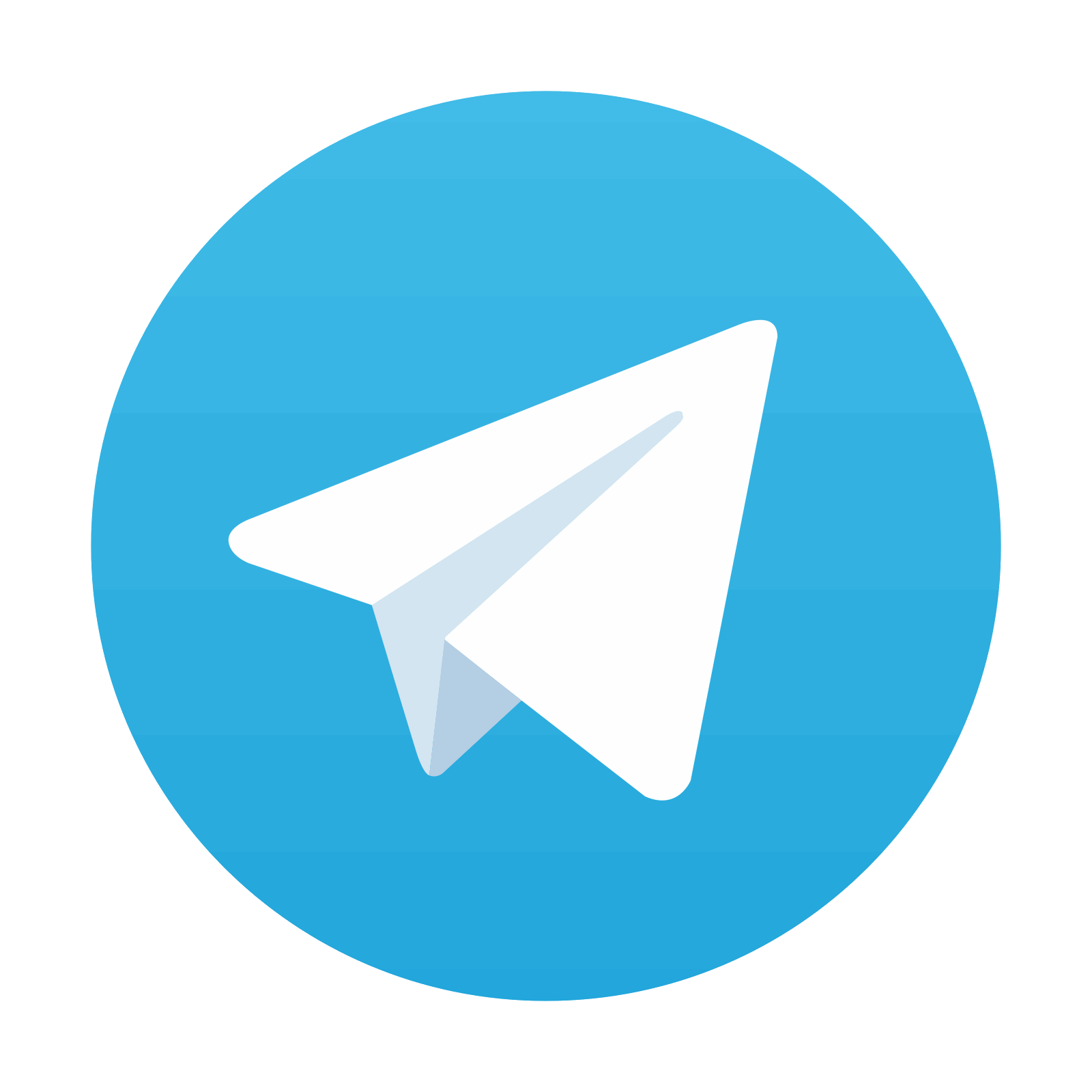
Stay updated, free articles. Join our Telegram channel

Full access? Get Clinical Tree
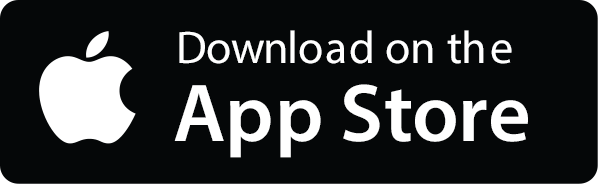
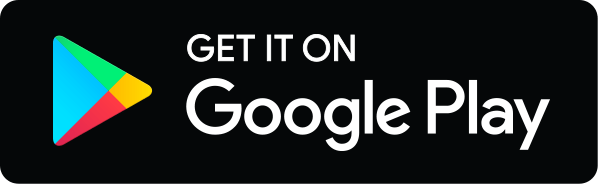