The anesthetic considerations for surgical resection of arteriovenous malformations (AVMs) and dural arteriovenous fistulas (DAVFs) incorporate many principles that are common to craniotomies for other indications. However, a high-flow, low-resistance shunt results in chronic hypoperfusion of adjacent brain tissue that is vulnerable to ischemia and at high risk for hyperemia and hemorrhage as resection of the lesion redirects blood flow. A comprehensive understanding of AVM pathophysiology and rapidly titratable anesthetic and vasoactive agents allow the anesthesiologist to alter blood pressure targets as resection evolves for optimal patient outcome. Intensive management is continued post-operatively as the brain acclimatizes to new parameters.
Cerebral vascular physiology of arteriovenous malformations and dural arteriovenous fistulas
Brain arteriovenous malformations (AVMs) are anatomically characterized by a tangle of thin-walled vessels that connect the high-pressure arterial circulation to the low-pressure venous system, without an intervening capillary network. Dural arteriovenous fistulas (DAVFs), by comparison, are simpler lesions typically described as either direct or indirect. Direct DAVFs (nonsinus type) have a well-defined arteriovenous shunt and involve only a few vessels. Indirect DAVFs (sinus type) are more complex lesions with numerous, small arterial feeders that travel in the dura and drain into a venous sinus. DAVFs are found outside the pia and involve the dura. The abnormal vasculature of AVMs and, to a lesser degree DAVFs, has several major physiologic effects. Steal phenomenon results when there is high flow across a low-resistance shunt, directly from arterial to venous circulation. The shunt results in decreased pressure in feeding arteries (lower than systemic pressure) and elevated pressure in draining veins. The arterial feeders and venous drainers of the AVM are derived from normal cerebral circulation, and the normal brain tissue adjacent to the nidus that they supply suffers from reduced cerebral perfusion pressure (CPP). Giant AVMs or DAVFs can steal flow from remote vascular territories. The degree of hypotension may result in cerebral blood flow that falls below the normal range of autoregulation. However, studies suggest that vascular reactivity to CO 2 is preserved, indicating intact autoregulation that has been shifted to the left rather than completely abolished. The tissue adjacent to the AVM has a lower tissue P o 2 than normal, but normal pH and P co 2 , suggesting an adaptive capillary exchange mechanism to maintain normal CO 2 clearance despite chronic hypoxia. During AVM resection, the tissue PO 2 and pH increase while the P co 2 falls. Another type of pathophysiology characteristic of AVMs is the development of diffuse bleeding and brain edema intraoperatively or postoperatively. One proposed mechanism, normal perfusion pressure breakthrough (NPPB), postulates that elimination of the AVM shunt redistributes blood flow to vasculature accustomed to chronic hypotension. The “occlusive hyperemia” hypothesis attributes brain edema to the occlusion of normal venous drainage of tissue adjacent to the AVM during surgery, resulting in vascular engorgement. These phenomena are discussed in detail by Miller and Mirski elsewhere in this issue.
Although less has been reported regarding the pathophysiology of DAVFs, there seems to be less of a concern for physiologic steal and resetting of autoregulation than that which may occur in larger cortical AVMs. Likewise, there is less of an issue with perfusion “breakthrough” edema. However, resection attempts of large DAVFs still pose an anesthetic concern with respect to sudden acute blood loss, venous air embolism, and postoperative seizures. Hence, many of the major anesthetic considerations for resection of parenchymal AVMs are applicable to craniotomies for other indications such as DAVFs.
Main anesthetic considerations
Targeted Cerebral Perfusion Pressure
Normotension versus induced hypotension
Under normal physiologic conditions, cerebral blood flow (CBF) is controlled by autoregulation over a range of mean arterial pressures (MAPs), traditionally 50 to 150 mm Hg. The introduction of an AVM, which functions as a low-pressure, high-flow shunt, can result in arterial hypotension in territories adjacent to the AVM. Under conditions of chronic hypoperfusion, the lower limit of autoregulatory pressure range (LAR) may be less than normal (shifted to the left) ( Fig. 1 ). At pressures below this lower limit, despite maximal vasodilation, these penumbral areas may become ischemic. Larger, more vascular AVMs place a more extensive penumbral area at risk for ischemia. The deliberate use of hypotension as a technique for minimizing blood loss prior to treatment of the AVM must be used with caution and serious consideration given to the impact of a further drop in pressure to a chronically hypoperfused area. If autoregulation is impaired because of chronic hypoperfusion, therapeutic interventions may result in an abnormal increase in CBF, hyperemia, cerebral edema, or hemorrhage. With progressive occlusion of the AVM and increase in perfusion pressure to the surrounding tissue, it may be beneficial to lower the mean arterial pressure to prevent hyperemia, and at the very least prevent elevation of the pressure above baseline.

Treatment of hypertension
Careful management of hemodynamic parameters is a consideration during general anesthesia for nearly any type of surgery, but avoidance of hemodynamic lability resulting in either ischemia or hyperemia is even more crucial with surgery for intracranial vascular malformations. Large variations in blood pressure are associated with induction of general anesthesia, direct laryngoscopy, placement of Mayfield pins, and surgical incision, and should be managed aggressively.
Required hypertension during ischemia
Certain interventions or complications of treatment for AVM may necessitate induced hypertension to prevent ischemia. The brain tissue adjacent to the AVM that is subject to chronic hypoperfusion may be exquisitely sensitive to interruption of normal blood flow. Thrombosis, embolization, compression from retraction, or temporary mechanical occlusion of feeding vessels may compromise already meager blood flow to penumbral areas, making may it necessary to increase CPP and promote flow through collateral means. Changes in the visual appearance of the brain tissue, attenuation of evoked potential signals, or poor or delayed flow seen on intraoperative angiography may alert the surgeon and anesthesiologist to the possibility that a higher pressure is required to perfuse vulnerable areas. Drugs with α1-agonist effects such as phenylephrine, ephedrine, norepinephrine, and epinephrine do not alter CBF in primates and humans, though they do cause cerebral vasoconstriction in other animal models. If autoregulation is intact, augmentation of MAP and thus CPP does not result in a change in CBF within the limits of autoregulation. In cases where the blood-brain barrier (BBB) is defective, norepinephrine may cause vasodilation. β-Adrenergic agonists in small doses have minimal effect on cerebral vasculature, but at higher doses can increase cerebral metabolic rate for O 2 (CMR o 2 ) with accompanying increase in CBF.
Hemorrhage Risk
Postoperative intracerebral hemorrhage after excision of an AVM may be caused by insufficient hemostasis and/or inadequate blood pressure control, incomplete resection with residual AVM nidus, NPPB, or venous thrombosis.
Blood product transfusion: packed red blood cells, fresh frozen plasma, platelets
The need for perioperative transfusion is affected by the patient’s starting hematocrit, cardiovascular status, and intraoperative blood loss. Surgically induced blood loss is dependent on several important factors: the size and location of the AVM, surgical expertise, and history of preoperative embolization or radiotherapy to minimize the number and caliber of feeding vessels. Blood loss can also be influenced by operative patient position and intraoperative manipulation of blood pressure. In one retrospective, nonrandomized study, embolization was performed for AVM in 168 patients, 124 of whom had embolization as an adjunct to surgery. Thirty-one percent of the surgical patients required a blood transfusion with a mean of 1.4 units of packed red blood cells per surgical patient with a range of 0 to 18 units. In cases where ongoing surgical blood loss is modest, transfusion of red cells, fresh frozen plasma (FFP), and platelets should be based on serial measures of hematocrit, coagulation studies, and platelet count and function, respectively. In the event of massive hemorrhage, current data from trauma literature supports a more empiric transfusion formulation with a high proportional target ratio of red cells/plasma/platelets of 1:1:1. It is reasonable to have several units typed and crossed at the start of the case; if the AVM is complex or technically difficult to approach, blood loss may be sudden and massive, and a cooler of stored blood should be available in the room and checked in advance for immediate use.
The threshold for transfusion of red cells is a subject of long-standing debate. General guidelines from the American Society of Anesthesiologists (ASA) state that “transfusion is indicated if the hemoglobin concentration is less than 6 g/dL and not indicated if >10 g/dL.” However, it is readily accepted that such guidelines must be modified in cases of high-risk procedures where there may be ongoing and substantial blood loss, such as AVM resection. Many guidelines, in addition, promote the consideration of organ ischemia (especially cerebral), ongoing bleeding, intravascular volume status, and patient’s risk factors in addition to the hemoglobin threshold.
Other considerations in addition to hemoglobin concentrations alone should assist in dictating optimal transfusion triggers during AVM resection surgery. Early canine studies, for example, demonstrated that hemodilution to a hematocrit of 30% resulted in limiting infarct volume following induction of focal cerebral ischemia as compared with either a higher or lower hematocrit. Thus, this hematocrit level was thought to strike the optimal balance between oxygen-carrying capacity and hemorheology or blood viscosity by decreasing cerebrovascular resistance and enhancing cerebral perfusion. Without definitive clinical support, the 30% blood hematocrit goal has nonetheless been a relative standard in neurosurgical cases over many years. Additional evidence in support of a more generous hemoglobin level during AVM cases comes from studies demonstrating that ischemic areas of the brain often lose their capacity for vascular autoregulation, in which case viscosity has a more significant impact on cerebral perfusion. Dhar and colleagues used positron emission topography scanning to demonstrate an increase in cerebral oxygen delivery and reduced oxygen extraction fraction after blood transfusion in patients with subarachnoid hemorrhage and a hemoglobin of less than 10 g/dL. When hemoglobin is less than 10 g/dL, the investigators concluded that the benefit of increased oxygen-carrying capacity outweighs the concern of increased viscosity, thereby increasing oxygen delivery and augmenting cerebral reserve.
There are additional infectious, immunologic, metabolic, and hemodynamic risks associated with transfusion, and the anesthesiology and neurosurgery teams must weigh all considerations in formulating their blood component targets. In light of the evidence presented it is reasonable, and also the authors’ physiologic strategy, to use a transfusion threshold of hemoglobin less than 10 g/dL in the intraoperative and postoperative patient undergoing AVM resection. The risk of sudden hemorrhage invoking critical cerebral ischemia requires a more liberal strategy than a stable, critically ill, nonneurologic patient.
Disseminated intravascular coagulation
Disseminated intravascular coagulation (DIC) is rare after an uncomplicated craniotomy. Cortical brain tissue has a high concentration of thromboplastins, and release of such compounds normally serves to stimulate coagulation. In certain pathologic states such as traumatic brain injury, brain tumor, or extensive surgical manipulation, the extrinsic coagulation cascade may be robustly triggered, resulting in extensive clotting (mainly in the microcirculation), activation of fibrinolysis, consumption of platelets and coagulation factors, and subsequent bleeding. In 3164 patients who underwent primary craniotomy at the Mayo Clinic, the investigators estimate the incidence of developing DIC within 72 hours of surgery to be between 0.13% and 0.44%, with a mortality rate ranging from 43% to 75%. Treatment is aimed at reversing the underlying cause of DIC and transfusing platelets, FFP, or fibrinogen-rich cryoprecipitate as indicated.
Intraoperative Monitoring of Evoked Potentials
Intraoperative cortical mapping has been used for AVMs located in particularly eloquent regions, such as the language (under awake anesthesia) or motor (under general anesthesia) cortex to facilitate its preservation. Many commonly used anesthetic agents affect evoked potentials. All volatile anesthetics, and to a lesser extent high-dose opioids, benzodiazepines, and sedative-hypnotic agents (propofol), cause an increase in latency and decrease in amplitude, although the effect is dose dependent and likely minimal in neurologically intact patients during surgery under routine anesthetic requirements. Nitrous oxide has a more substantial effect, decreasing signal amplitude. Because motor evoked potentials are more sensitive to anesthetics than somatosensory evoked potentials, their employment may warrant the use of intravenous anesthetic agents in place of volatiles or nitrous oxide, but these considerations must be weighed with each anesthetic’s effect on hemodynamics, cerebral metabolism and blood flow, and impact on postoperative neurologic assessment.
Postoperative Neurologic Assessment
Postoperative neurologic assessment is of crucial importance following AVM resection, and the anesthesiologist must facilitate a smooth emergence from anesthesia in order for the patient to be thoroughly evaluated. A comprehensive preoperative neurologic examination that documents any baseline deficits is obligatory. The anesthetic plan at the conclusion of the case should consist of rapidly titratable agents that permit fast recovery from general anesthesia; this can be achieved with a total intravenous anesthetic, volatile anesthetics, or a combination of agents. Drugs that may be helpful in this regard include nitrous oxide, fentanyl, and remifentanil. When intraoperative neuromonitoring signals are of concern, it is common practice to use multiple agents for maintenance to minimize the impact of any single agent on signal strength.
Main anesthetic considerations
Targeted Cerebral Perfusion Pressure
Normotension versus induced hypotension
Under normal physiologic conditions, cerebral blood flow (CBF) is controlled by autoregulation over a range of mean arterial pressures (MAPs), traditionally 50 to 150 mm Hg. The introduction of an AVM, which functions as a low-pressure, high-flow shunt, can result in arterial hypotension in territories adjacent to the AVM. Under conditions of chronic hypoperfusion, the lower limit of autoregulatory pressure range (LAR) may be less than normal (shifted to the left) ( Fig. 1 ). At pressures below this lower limit, despite maximal vasodilation, these penumbral areas may become ischemic. Larger, more vascular AVMs place a more extensive penumbral area at risk for ischemia. The deliberate use of hypotension as a technique for minimizing blood loss prior to treatment of the AVM must be used with caution and serious consideration given to the impact of a further drop in pressure to a chronically hypoperfused area. If autoregulation is impaired because of chronic hypoperfusion, therapeutic interventions may result in an abnormal increase in CBF, hyperemia, cerebral edema, or hemorrhage. With progressive occlusion of the AVM and increase in perfusion pressure to the surrounding tissue, it may be beneficial to lower the mean arterial pressure to prevent hyperemia, and at the very least prevent elevation of the pressure above baseline.
Treatment of hypertension
Careful management of hemodynamic parameters is a consideration during general anesthesia for nearly any type of surgery, but avoidance of hemodynamic lability resulting in either ischemia or hyperemia is even more crucial with surgery for intracranial vascular malformations. Large variations in blood pressure are associated with induction of general anesthesia, direct laryngoscopy, placement of Mayfield pins, and surgical incision, and should be managed aggressively.
Required hypertension during ischemia
Certain interventions or complications of treatment for AVM may necessitate induced hypertension to prevent ischemia. The brain tissue adjacent to the AVM that is subject to chronic hypoperfusion may be exquisitely sensitive to interruption of normal blood flow. Thrombosis, embolization, compression from retraction, or temporary mechanical occlusion of feeding vessels may compromise already meager blood flow to penumbral areas, making may it necessary to increase CPP and promote flow through collateral means. Changes in the visual appearance of the brain tissue, attenuation of evoked potential signals, or poor or delayed flow seen on intraoperative angiography may alert the surgeon and anesthesiologist to the possibility that a higher pressure is required to perfuse vulnerable areas. Drugs with α1-agonist effects such as phenylephrine, ephedrine, norepinephrine, and epinephrine do not alter CBF in primates and humans, though they do cause cerebral vasoconstriction in other animal models. If autoregulation is intact, augmentation of MAP and thus CPP does not result in a change in CBF within the limits of autoregulation. In cases where the blood-brain barrier (BBB) is defective, norepinephrine may cause vasodilation. β-Adrenergic agonists in small doses have minimal effect on cerebral vasculature, but at higher doses can increase cerebral metabolic rate for O 2 (CMR o 2 ) with accompanying increase in CBF.
Hemorrhage Risk
Postoperative intracerebral hemorrhage after excision of an AVM may be caused by insufficient hemostasis and/or inadequate blood pressure control, incomplete resection with residual AVM nidus, NPPB, or venous thrombosis.
Blood product transfusion: packed red blood cells, fresh frozen plasma, platelets
The need for perioperative transfusion is affected by the patient’s starting hematocrit, cardiovascular status, and intraoperative blood loss. Surgically induced blood loss is dependent on several important factors: the size and location of the AVM, surgical expertise, and history of preoperative embolization or radiotherapy to minimize the number and caliber of feeding vessels. Blood loss can also be influenced by operative patient position and intraoperative manipulation of blood pressure. In one retrospective, nonrandomized study, embolization was performed for AVM in 168 patients, 124 of whom had embolization as an adjunct to surgery. Thirty-one percent of the surgical patients required a blood transfusion with a mean of 1.4 units of packed red blood cells per surgical patient with a range of 0 to 18 units. In cases where ongoing surgical blood loss is modest, transfusion of red cells, fresh frozen plasma (FFP), and platelets should be based on serial measures of hematocrit, coagulation studies, and platelet count and function, respectively. In the event of massive hemorrhage, current data from trauma literature supports a more empiric transfusion formulation with a high proportional target ratio of red cells/plasma/platelets of 1:1:1. It is reasonable to have several units typed and crossed at the start of the case; if the AVM is complex or technically difficult to approach, blood loss may be sudden and massive, and a cooler of stored blood should be available in the room and checked in advance for immediate use.
The threshold for transfusion of red cells is a subject of long-standing debate. General guidelines from the American Society of Anesthesiologists (ASA) state that “transfusion is indicated if the hemoglobin concentration is less than 6 g/dL and not indicated if >10 g/dL.” However, it is readily accepted that such guidelines must be modified in cases of high-risk procedures where there may be ongoing and substantial blood loss, such as AVM resection. Many guidelines, in addition, promote the consideration of organ ischemia (especially cerebral), ongoing bleeding, intravascular volume status, and patient’s risk factors in addition to the hemoglobin threshold.
Other considerations in addition to hemoglobin concentrations alone should assist in dictating optimal transfusion triggers during AVM resection surgery. Early canine studies, for example, demonstrated that hemodilution to a hematocrit of 30% resulted in limiting infarct volume following induction of focal cerebral ischemia as compared with either a higher or lower hematocrit. Thus, this hematocrit level was thought to strike the optimal balance between oxygen-carrying capacity and hemorheology or blood viscosity by decreasing cerebrovascular resistance and enhancing cerebral perfusion. Without definitive clinical support, the 30% blood hematocrit goal has nonetheless been a relative standard in neurosurgical cases over many years. Additional evidence in support of a more generous hemoglobin level during AVM cases comes from studies demonstrating that ischemic areas of the brain often lose their capacity for vascular autoregulation, in which case viscosity has a more significant impact on cerebral perfusion. Dhar and colleagues used positron emission topography scanning to demonstrate an increase in cerebral oxygen delivery and reduced oxygen extraction fraction after blood transfusion in patients with subarachnoid hemorrhage and a hemoglobin of less than 10 g/dL. When hemoglobin is less than 10 g/dL, the investigators concluded that the benefit of increased oxygen-carrying capacity outweighs the concern of increased viscosity, thereby increasing oxygen delivery and augmenting cerebral reserve.
There are additional infectious, immunologic, metabolic, and hemodynamic risks associated with transfusion, and the anesthesiology and neurosurgery teams must weigh all considerations in formulating their blood component targets. In light of the evidence presented it is reasonable, and also the authors’ physiologic strategy, to use a transfusion threshold of hemoglobin less than 10 g/dL in the intraoperative and postoperative patient undergoing AVM resection. The risk of sudden hemorrhage invoking critical cerebral ischemia requires a more liberal strategy than a stable, critically ill, nonneurologic patient.
Disseminated intravascular coagulation
Disseminated intravascular coagulation (DIC) is rare after an uncomplicated craniotomy. Cortical brain tissue has a high concentration of thromboplastins, and release of such compounds normally serves to stimulate coagulation. In certain pathologic states such as traumatic brain injury, brain tumor, or extensive surgical manipulation, the extrinsic coagulation cascade may be robustly triggered, resulting in extensive clotting (mainly in the microcirculation), activation of fibrinolysis, consumption of platelets and coagulation factors, and subsequent bleeding. In 3164 patients who underwent primary craniotomy at the Mayo Clinic, the investigators estimate the incidence of developing DIC within 72 hours of surgery to be between 0.13% and 0.44%, with a mortality rate ranging from 43% to 75%. Treatment is aimed at reversing the underlying cause of DIC and transfusing platelets, FFP, or fibrinogen-rich cryoprecipitate as indicated.
Intraoperative Monitoring of Evoked Potentials
Intraoperative cortical mapping has been used for AVMs located in particularly eloquent regions, such as the language (under awake anesthesia) or motor (under general anesthesia) cortex to facilitate its preservation. Many commonly used anesthetic agents affect evoked potentials. All volatile anesthetics, and to a lesser extent high-dose opioids, benzodiazepines, and sedative-hypnotic agents (propofol), cause an increase in latency and decrease in amplitude, although the effect is dose dependent and likely minimal in neurologically intact patients during surgery under routine anesthetic requirements. Nitrous oxide has a more substantial effect, decreasing signal amplitude. Because motor evoked potentials are more sensitive to anesthetics than somatosensory evoked potentials, their employment may warrant the use of intravenous anesthetic agents in place of volatiles or nitrous oxide, but these considerations must be weighed with each anesthetic’s effect on hemodynamics, cerebral metabolism and blood flow, and impact on postoperative neurologic assessment.
Postoperative Neurologic Assessment
Postoperative neurologic assessment is of crucial importance following AVM resection, and the anesthesiologist must facilitate a smooth emergence from anesthesia in order for the patient to be thoroughly evaluated. A comprehensive preoperative neurologic examination that documents any baseline deficits is obligatory. The anesthetic plan at the conclusion of the case should consist of rapidly titratable agents that permit fast recovery from general anesthesia; this can be achieved with a total intravenous anesthetic, volatile anesthetics, or a combination of agents. Drugs that may be helpful in this regard include nitrous oxide, fentanyl, and remifentanil. When intraoperative neuromonitoring signals are of concern, it is common practice to use multiple agents for maintenance to minimize the impact of any single agent on signal strength.
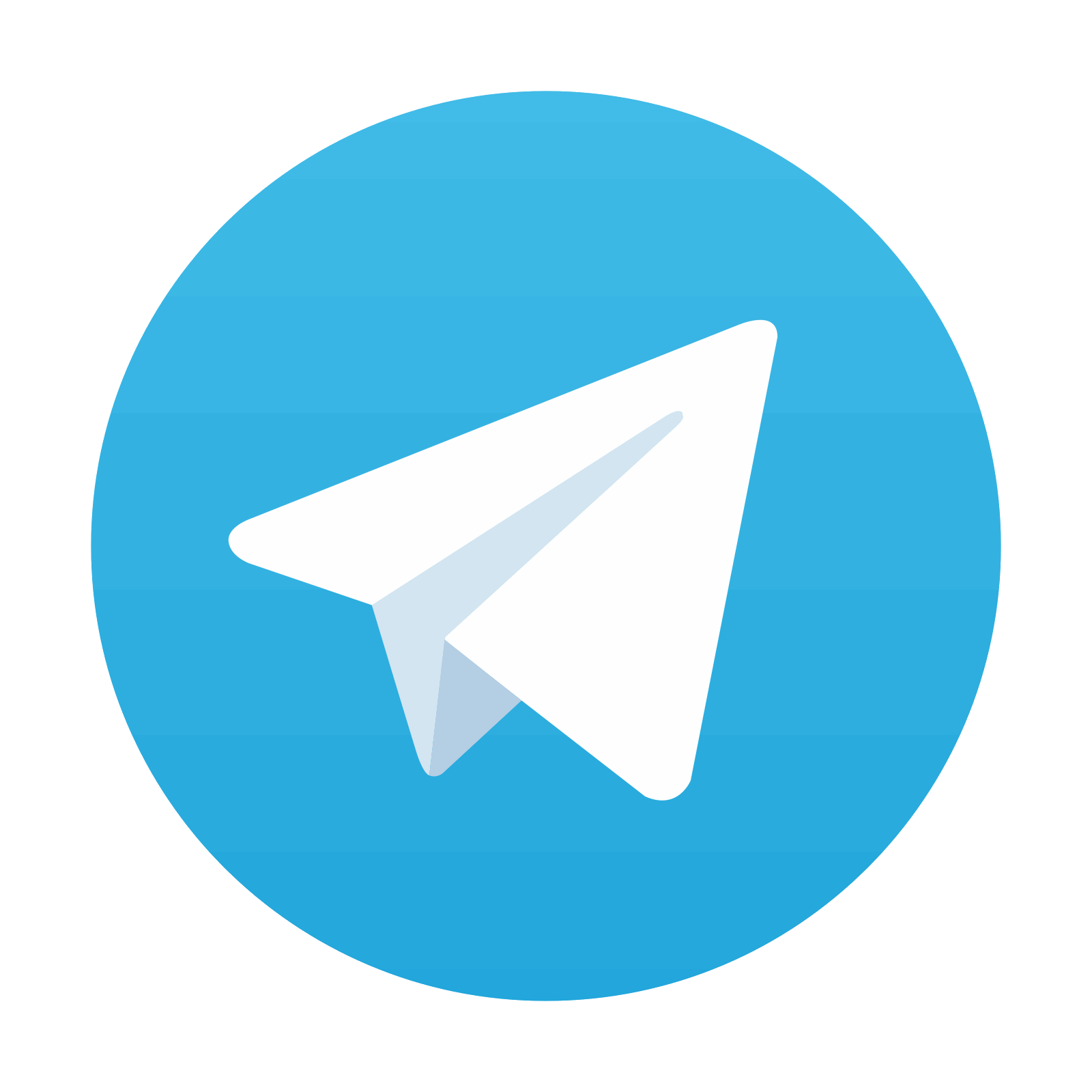
Stay updated, free articles. Join our Telegram channel

Full access? Get Clinical Tree
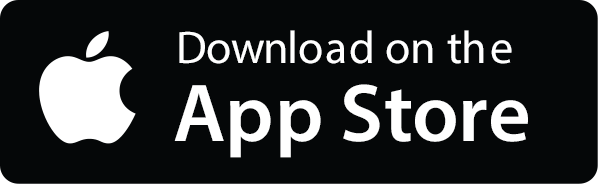
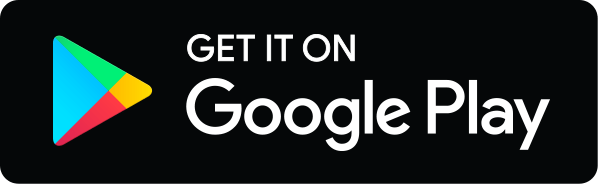