Anesthesia Considerations for Neurovascular Surgery
Pearls
Plasma biomarkers of cardiac dysfunction (e.g., troponin-I, serum B-type natriuretic peptide) are predictive of cardiovascular dysfunction after subarachnoid hemorrhage (SAH) and should be measured in all SAH patients. In the presence of elevated levels, or clinical symptoms of cardiac dysfunction (e.g., pulmonary edema, hemodynamic instability), a transthoracic echocardiogram should be performed and central venous or pulmonary artery monitoring should be considered for perioperative management.
Maintaining global and local cerebral perfusion pressure to avoid focal ischemia due to vasospasm or inadequate watershed blood flow is just as important as avoiding cerebral hypertension, which can cause aneurysm rupture or cerebral hyperemia.
Brain relaxation not only facilitates surgical dissection, but also may avert neurologic injury due to a local decrease in cerebral perfusion pressure. Hyperosmotic therapy with mannitol and/ or hypertonic saline along with maneuvers that optimize cerebral venous drainage (e.g., reverse Trendelenburg position, limited intrathoracic pressure, avoidance of excessive jugular vein torsion) are simple methods that may achieve and maintain adequate brain relaxation long after the effects of hypocapnia have waned.
Intraoperative maintenance of normoglycemia (80–120 mg/dL) during aneurysm clip ligation is associated with improved neuropsychiatric and Glasgow Outcome Scale outcomes at 3 and 12 months. Intensive insulin therapy with bolus and infusions of insulin can rapidly achieve normoglycemia; however, to avoid hypoglycemia, frequent monitoring of serum glucose is required (i.e., every 15 to 30 minutes).
Anatomically directed neurophysiologic monitoring may serve as an early warning sign of impending neurologic injury. Systematic investigation of the etiology of a change in neurophysiologic monitoring may allow early intervention to circumvent surgical or physiologic insults that produce intraoperative neuronal damage and postoperative neurologic morbidity.
The fundamental goal of general anesthesia is to produce a patient state in which surgical procedures can be safely performed. Besides maintaining an unaware patient who is oblivious to noxious stimulation, much time and effort is spent in maintaining homeostasis by frequently monitoring hemodynamic parameters and titrating and adding pharmacologic agents to maintain adequate perfusion to all organs. As more and more complex neurosurgical procedures are performed in patients with more severe acute and chronic coexisting diseases, the anesthesiologist also spends a significant amount of time monitoring and treating abnormalities in oxygen delivery and coagulation while employing more sophisticated monitors of neurologic well-being (e.g., processed electroencephalograms, evoked neurologic electrophysiologic responses) to maintain adequate blood flow to the neuronal tissue at risk. This chapter focuses on the physiologic and pharmacologic concepts that form the foundation for providing perioperative care of the complex neurovascular patient.
♦ Preoperative Management
Close collaboration and communication between the surgical and anesthesia teams allows formulation of the most appropriate perioperative plan for the patient. The preoperative laboratory tests that should be obtained prior to even urgent neurovascular surgery are presented in Table 13.1 . When time permits, it is essential that all patients with complex intracranial lesions undergo preoperative cardiac testing. The goal of this evaluation is not to identify candidates in need of cardiac revascularization, but rather to identify patients who may require more invasive hemodynamic monitoring to allow the safe administration of vasoactive agents to maintain intraoperative cerebral perfusion. Patients who have poorly controlled hypertension, metabolic syndrome, known coronary artery disease, or congestive heart failure should undergo, at a minimum, preoperative resting echocardiography. When clinical signs of angina are present, and to minimize the chances of a hypertension-induced aneurysmal rupture, adenosine sestamibi or thallium nuclear stress testing should be considered in place of treadmill or dobutamineinduced stress tests. The data from these tests will identify those patients with either a decreased ejection fraction (<25%) or significant coronary artery disease who may require perioperative pulmonary artery catheterization.
Patients presenting with subarachnoid hemorrhage (SAH) usually represent a more complex group to evaluate preoperatively because of the high incidence of electrocardiographic abnormalities, the presence of major clinical risk factors for concomitant coronary artery and systemic arterial atherosclerosis, and the real phenomenon of SAH-induced cardiac dysfunction.1 , 2 Electrical disturbances as well as possible myocardial lesions are summarized in Table 13.2 , in increasing order of severity. Despite the association between post-SAH electrocardiogram (ECG) abnormalities and post-SAH 3-month mortality, there is no association between SAHinduced ECG abnormalities and myocardial function.2 In fact, myocardial wall motion abnormalities, which are well documented by echocardiogram or nucleotide venticulography, are not related to coronary artery disease.3 These transient wall motion abnormalities (<14 days) are caused by the catecholamine surge that occurs with SAH and follow a noncoronary artery distribution. Because of the strong association between plasma biomarkers of myocardial injury (e.g., cardiac troponin I [cTI], and serum B-type natriuretic peptide [BNP]) and clinically significant left ventricular dysfunction, all patients with SAH should have a baseline cTI and BNP measured on admission.4 , 5 Subsequent preoperative workup is based on these levels and the presence or absence of clinical signs of heart failure (Fig. 13.1). Those patients with clinically significant ventricular dysfunction are more likely to require perioperative vasoactive agents to maintain cerebral perfusion pressure that may necessitate the placement of a cardiac output monitors (e.g., pulmonary artery catheter, transesophageal echocardiography).
♦ Intraoperative Management
Perioperative management of a patient undergoing neurovascular intervention should focus on the following goals:
Maintaining adequate cerebral perfusion pressure and avoiding secondary cerebral ischemia due to hypoperfusion
Optimizing working conditions for the neurosurgeon, including providing adequate brain relaxation throughout surgery to minimize the risk of retractor injury
Monitoring for impending neurologic injury and performing corrective maneuvers to undo surgical and physiologic induced changes that may be associated with neurologic injury
Hemodynamic Management
One of the most important goals of intraoperative management of neurovascular patients is to maintain adequate cerebral perfusion pressure, thereby preventing secondary cerebral injury due to hypoperfusion (ischemia). In addition, it is important to avoid unnecessary hypertension, which can lead to aneurysmal rupture or perfusion pressure breakthrough (e.g., perilesion edema, resection bed hemorrhage). All anesthetic regimens that maintain patient amnesia/sedation, prevent patient movement in response to noxious stimulation, and provide hemodynamic stability have demonstrated equal safety and efficacy in intracranial surgery.6 The following perioperative events may be associated with transient noxious stimulation that can lead to rapid increases in blood pressure:
Laryngoscopy and tracheal intubation
Placement in Mayfield head fixation
Surgical incision
Dural traction
Brainstem manipulation
Placement of brain retractors during posterior fossa and middle fossa surgery

Overly aggressive prophylaxis for treatment of hypertension may lead to unintended hypotension and inadequate cerebral perfusion. Therefore, with the use of ultrarapid offset opioids (e.g., remifentanil or alfentanil) and short-acting vasoactive agents (e.g., esmolol, nicardipine, or nitroprusside), hypertension from these brief, noxious stimuli can be prevented or treated, whereas unintended hypotension is limited in duration and easily corrected. Special consideration should be given to hemodynamic management at the time of temporary occlusion of feeding vessels to facilitate permanent clip placement. To ensure adequate distal cerebral perfusion through collateral vessels, it is necessary to increase mean arterial pressure (MAP) at this time by approximately 20% from baseline values. Once temporary clips are removed and neuromonitoring signals are confirmed to be unchanged, MAP can be returned to its original level.
When temporary clipping of parent vessels is not an option for anatomic/morphologic reasons, adenosine-induced flow arrest offers a unique method of transiently and profoundly reducing systolic blood pressure so as to facilitate clip ligation of the aneurysm.7 Other techniques to reduce aneurysm neck turgor that have been described include deep hypothermic circulatory arrest, temporary occlusion of the extracranial common carotid artery, and endovascular balloon catheter retrograde suction deflation.8 These methods, however, are logistically more difficult to carry out. Brief periods of profound hypotension can also be produced by infusions and boluses of sodium nitroprusside or esmolol, although both of these drugs are less predictable in their dose– response relationships. Adenosine has proven to be a safe, predictable agent for achieving the brief flow arrest often needed to apply a permanent clip, and can be used repeatedly. Compared with deep hypothermic circulatory arrest, adenosine offers the advantage of avoiding the coagulopathy associated with deep hypothermia, as well as the hyperglycemia and rebound hyperthermia that occur after cardiopulmonary bypass.9 Based on our observational series, in patients receiving a background anesthetic consisting of remifentanil, 0.5 minimum alveolar concentration (MAC) of volatile anesthetic, and a propofol infusion achieving a burst suppression ratio of 0.7, there is a significant linear relationship between the dose of adenosine, the duration of asystole, and the duration of profound hypotension (Fig. 13.2). An adenosine dose of 0.35 mg per kilogram of ideal body weight (preferably delivered via a central venous catheter) is the recommended starting dose to achieve approximately 45 seconds of profound systemic hypotension.10 Timing of adenosine administration, whether planned or after inadvertent intraoperative rupture of an aneurysm, must be carefully coordinated with the neurosurgeon so that adequate working time is available for the successful placement of each clip or series of clips.
Patient Positioning
Neurovascular surgical procedures can often last anywhere from 5 to 24 hours. Careful attention by the entire operative team should be paid to the positioning of the patient. Prolonged pressure or stretch of peripheral nerves (e.g., brachial plexus, ulnar nerve) may result in peripheral neuropathy. This risk may be minimized by using sufficient padding to vulnerable pressure points, adjusting all joints so that there is not extreme stretch on any neural plexus, and utilizing neurophysiologic monitors to warn of the development of peripheral nerve injury and adjusting the affected limb to correct the problem.11 Positioning may also impact the surgical field if the patient’s trunk and limbs are not adequately secured to allow sufficient manipulation or rotation of the operating table without shifting the body. Finally, ignoring the effects of a patient’s position on jugular venous drainage or the pressure on the abdomen and chest may result in inadequate cerebral venous drainage that may impede adequate brain relaxation despite aggressive therapeutic maneuvers (e.g., excessive hyperosmotic therapy or cerebrospinal fluid [CSF] drainage). In addition, excessive neck flexion or rotation may impede venous drainage from the tongue, which, in the extremes of circumstances, could result in tongue edema and even necrosis, requiring prolonged endotracheal intubation due to airway obstruction.

Brain Bulk Management
Actively managing brain bulk, even in those patients with a normal starting intracranial pressure, may reduce direct pressure exerted by retractors in the field, thereby minimizing the risk of retractor-induced brain ischemia and injury.12 Aside from acute short-term hyperventilation, hyperosmolar therapy has long been the cornerstone of brain bulk and intracranial pressure treatment. Mannitol and hypertonic saline are the osmotic agents of choice (Table 13.3). 13 – 15 Both agents decrease brain bulk and intracranial pressure by two different mechanisms. The initial mechanism is a decrease in blood viscosity that improves flow and increases oxygen delivery to brain tissue. This results in a compensatory vasoconstriction that reduces cerebral blood volume and therefore intracranial pressure. The delayed but major mechanism of reduced brain bulk is an osmotically mediated decrease in brain water content. Mannitol is renally excreted and produces a strong osmotic diuretic effect. Hypertonic saline, on the other hand, has a relatively small natriuretic effect, making it an attractive alternative to mannitol in certain circumstances (e.g., when cerebral perfusion pressure is difficult to maintain otherwise). Prospective, randomized, double-blind studies comparing the effects of hypertonic saline and mannitol on brain relaxation and serum electrolyte balance showed that both modalities were efficacious, though hypertonic saline may be more efficacious in achieving brain relaxation in supratentorial brain tumor patients. In addition, the mannitol group had decreased serum sodium, a greater fluid deficit, and increased plasma lactate levels compared with the hypertonic saline group.14 , 15 Depending on the extent of initial brain bulk, 0.25 to 1 g/kg of mannitol is usually infused intravenously over 10 to 20 minutes. Alternatively, a dose of 1 to 2 mL/kg of 3% saline can be given as an intravenous bolus, followed by an infusion of 1 to 2 mL/kg/h with a plasma sodium goal of 145 to 155 mEq/dL. If repeated doses of mannitol, or long-term infusions of hypertonic saline, are needed, plasma osmolality should be closely monitored and kept under 320 mOsm/L. In addition, care should be taken to monitor for rebound cerebral edema that may occur with the abrupt discontinuation of any infusions of a hyperosmotic agent.16
There are other effective ways of decreasing brain bulk (Table 13.4). Perhaps the simplest, and often most effective, way to manage brain bulk is to pay close attention to head and neck positioning. During fixation of the Mayfield device to the operating room table, care must be taken to avoid excessive flexion or rotation of the neck that may obstruct cerebral venous outflow. Placing the patient in 10 to 30 degrees of reverse Trendelenburg position facilitates venous outflow as well as CSF drainage and lowers intracranial pressure (ICP). However, if orthostatic hypotension occurs it should be treated with volume replacement or vasoconstrictors to maintain adequate cerebral perfusion pressure.
As mentioned above, hyperventilation-induced hypocapnia, with a goal PaCO2 of 30 mm Hg, rapidly and effectively reduces ICP; more aggressive hyperventilation can aggravate cerebral ischemia through excessive vasoconstriction. This effect on cerebral arterial resistance is short lived because the brain compensates for CSF respiratory alkalosis by increasing bicarbonate elimination from the CSF.
Decreasing the cerebral metabolic rate (CMR) effectively reduces brain bulk by decreasing cerebral blood flow requirements. There are two methods of decreasing CMR. The administration of a bolus or infusion of a hypnotic such as thiopental or propofol is the most rapid and controllable method. Hypothermia, on the other hand, is more difficult to achieve rapidly without overshoot. Moreover, hypothermia is not only difficult to reverse due to the lack of efficient rewarming devices, but also, if the core temperature is <33.5°C, it may produce a coagulopathy and hypokalemia that not only cause surgical and hemodynamic disturbances, respectively, but also may result in delayed awakening secondary to decreased metabolic clearance of anesthetics (e.g., propofol, neuromuscular junction blocking agents). Although volatile agents decrease CMR, they are direct cerebral arterial dilators. Therefore, when other modalities have failed to provide adequate brain relaxation, their discontinuation can also be considered, and a total intravenous anesthesia technique, utilizing an opioid and 100 to 200 μg/kg/min of propofol, can be used instead.6
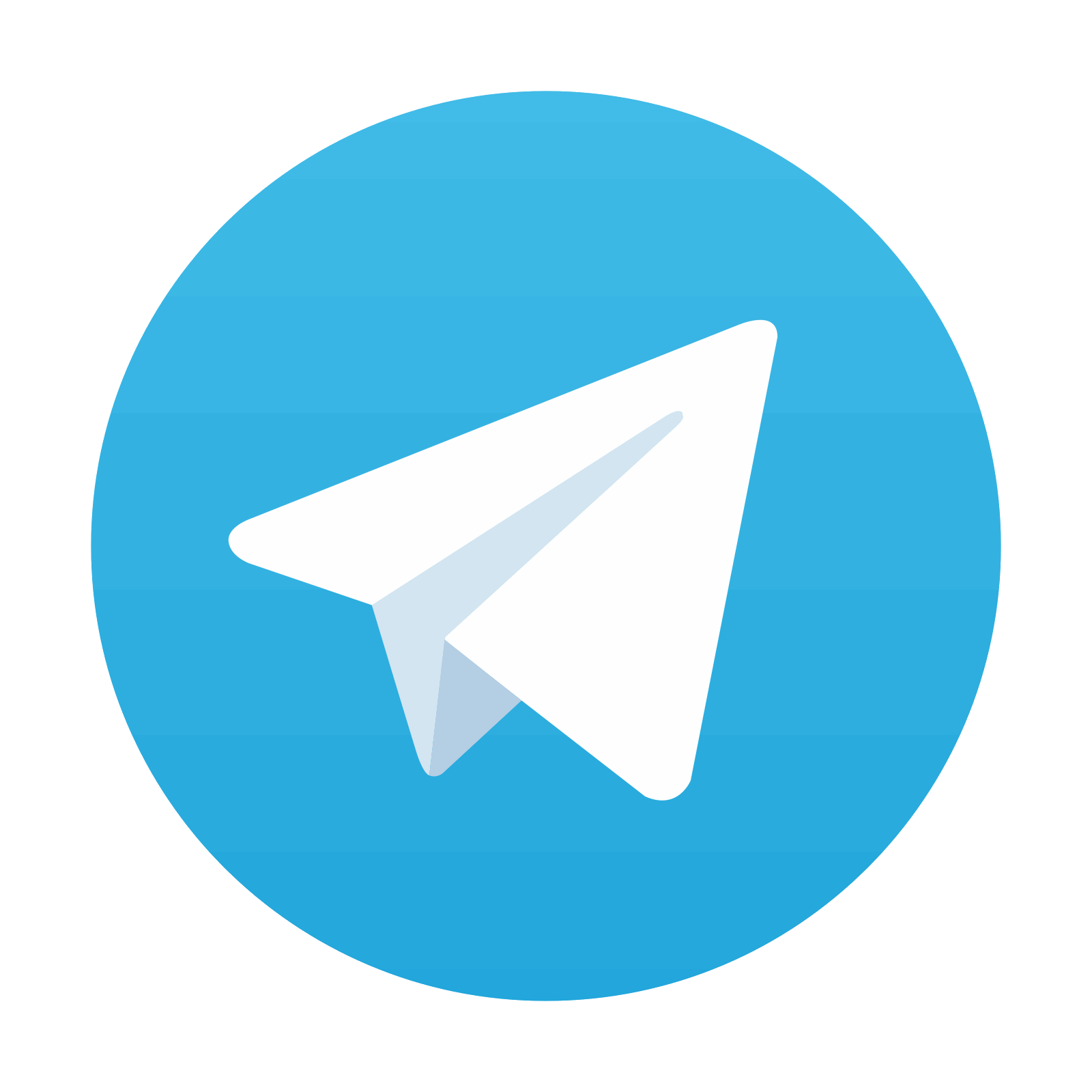
Stay updated, free articles. Join our Telegram channel

Full access? Get Clinical Tree
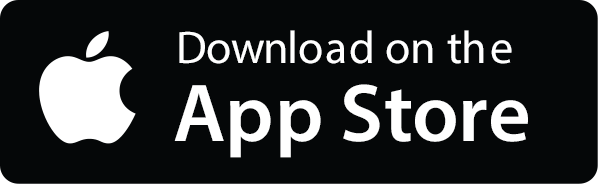
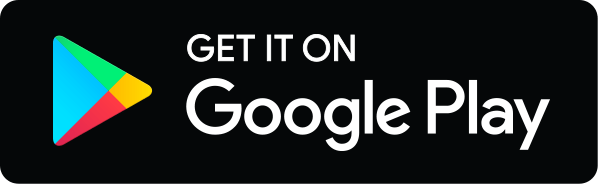
