Animal Models of Depression and Epilepsy: The Genetically Epilepsy-Prone Rat
Phillip C. Jobe
Ronald A. Browning
Two strains of independently derived genetically epilepsy-prone rats (GEPRs) have been developed, one designated GEPR-3 and the other GEPR-9 (1,2,3). GEPR-3s have been shown to model aspects of epilepsy and affective disorder comorbidity (4,5,7). GEPR-3s are predisposed both to the expression of seizures and to dysfunctional affective episodes.
Comorbidity between the epilepsies and affective disorders in humans is a topic of increasing scientific interest (8,9,10,11,30). Evidence supports the concept that individuals with epilepsy are more likely to experience dysfunctional affective episodes than people in the general population. Moreover, the relationship appears to be bidirectional (13,14,15,16,17,18,19,20,21,22,23,24,25). Patients with affective disorder have an elevated risk for epilepsy. Conversely, individuals with epilepsy have an elevated possibility for expressing affective disorder.
Epilepsy and affective disorder comorbidity does not merely occur by happenstance in the same individual. Rather, evidence supports the hypothesis that people with comorbidity suffer from an underlying pathophysiology that causes predisposition to both disorders (4,5,17,18,30,32,34). A large body of evidence indicates that noradrenergic and serotonergic deficits are fundamental elements of the pathophysiology of depressive disorder. Likewise, these deficits are important causes of mammalian seizure predisposition.
The noradrenergic and serotonergic deficit hypothesis of predisposition both to epilepsy and depressive disorder was first published approximately 7 years ago (4), after having been presented earlier in other venues including scientific meetings and discussions with the U.S. Food and Drug Administration (FDA) (29). Additional details have appeared in the new millennium. Noradrenergic and serotonergic deficits are believed to be only two of several mechanisms that may cause predilection to seizures and dysfunctional affective episodes (5).
Criteria for determining the validity of a mammalian model of human depressive disorders are analogous to those employed in evaluating models of human epilepsy. In biological psychiatry, these are labeled face, construct, predictive, and provocation (etiological) validity (30). In the current chapter, face validity denotes that the mammalian model exhibits a symptom profile that is
similar to that of humans with epilepsy and depressive disorder. Predictive validity requires that the model correctly identify treatments that are useful in the management of patients with depression and epilepsy. Provocation validity or etiological validity asserts that events that elicit human seizures and depressive episodes must also evoke analogous episodes in the model. Construct validity requires that the underlying pathophysiology in the model be equivalent to that of humans with epilepsy and depressive disorder.
similar to that of humans with epilepsy and depressive disorder. Predictive validity requires that the model correctly identify treatments that are useful in the management of patients with depression and epilepsy. Provocation validity or etiological validity asserts that events that elicit human seizures and depressive episodes must also evoke analogous episodes in the model. Construct validity requires that the underlying pathophysiology in the model be equivalent to that of humans with epilepsy and depressive disorder.
The Genetically Epilepsy-prone Rat as a Model of Mammalian Epilepsy and Depressive Disorder Comorbidity
Face Validity
Expression of Dysfunctional Episodes
Seizures
Two sets of seizure circuitries that drive generalized motor behaviors have been identified in mammals. Both exist within the GEPR brain as well as in the brains of other epileptic and nonepileptic mammals, including humans (31,32,33,34). These circuitry sets are designated as forebrain seizure circuitry and brainstem seizure circuitry, respectively. Major differences characterize the behaviors driven by each set of circuitry. Indeed, the characteristics of the behaviors and the electroencephalogram (EEG) provide strong presumptive evidence for the anatomical location of the seizure-driving circuitry (35).
Forebrain seizures in GEPRs correspond electrographically and behaviorally to partial seizures in humans, whereas brainstem seizures in GEPRs correspond to generalized tonic-clonic seizures (GTCSs) in humans (35). Other subhuman mammals, including epileptic and nonepileptic primates, also express brainstem and forebrain seizure behaviors, either spontaneously or in response to appropriate stimuli.
Dysfunctional Affective Episodes
Dysfunctional affective episodes or their analog manifestations are well known in humans and have been reported for other mammals. The circuitry for dysfunctional episodes probably exists in normal brain as well as in the brains of mammals suffering from affective disorder. Normal people experience elements of depressive episodes if negative life events are of sufficient magnitude. Loss of a spouse or an intimate friend may be sufficient to cause bereavement with manifestations that mimic many of those exhibited in a depressive episode. Other stressors are also believed to contribute to similar consequences. Genetic factors and environmental influences as determinants of affective disorder are prominent themes in biological psychiatry. A substantial body of evidence supports the view that stressful events contribute to the development of depressive illness (30,36,37,38). GEPR-3s exhibit analog manifestations of dysfunctional affective episodes in response to a stressful event known as the forced swim test (7). For example, in the forced swim test, the rate and extent of immobility development are much more pronounced in GEPR-3s than in nonepileptic controls.
Predisposition to Dysfunctional Episodes
Seizures
A communiqué on the term “epilepsy” was published in 2005 jointly by the International League Against Epilepsy and the International Bureau for Epilepsy (39).
Epilepsy is a disorder of the brain characterized by an enduring predisposition to generate epileptic seizures and by the neurobiologic, cognitive, psychological, and social consequences of this condition. The definition of epilepsy requires the occurrence of at least one epileptic seizure.
Seizure predisposition is a prominent trait of the GEPR, with this attribute being more pronounced in the GEPR-9 than in the GEPR-3 strain. At least some of the neurobiological determinants of seizure predisposition appear to underlie a comorbidity of the
GEPR-3, wherein this model is also predisposed to analog manifestations of depressive disorder (7). Although investigations into depressive disorder are more limited with GEPR-9s than with GEPR-3s, both strains may ultimately be shown to be models of comorbidity.
GEPR-3, wherein this model is also predisposed to analog manifestations of depressive disorder (7). Although investigations into depressive disorder are more limited with GEPR-9s than with GEPR-3s, both strains may ultimately be shown to be models of comorbidity.
The data for seizure predisposition in GEPRs have been described and summarized (4,5,35,40,30). As in humans with epilepsy, seizures in GEPRs are triggered by endogenous and exogenous stimuli that fail to produce seizures in nonepileptic mammals. Also, GEPRs and humans with epilepsy exhibit exaggerated seizure responsiveness and/or abnormally low thresholds to several convulsant chemicals and electrical stimuli.
Endogenous and Exogenous Stimuli that Cause Seizures in GEPRs, but Fail to Produce Seizures in Nonepileptic Mammals
Infrequent spontaneous GTCSs occur in intact GEPRs and most humans with epilepsy, but not in intact nonepileptic mammals (1,42,43). It is believed that these seizures are triggered by endogenous variations in neuronal/glial cell activity coupled with persistent seizure predisposition. In GEPR-3s and GEPR-9s, seizure predisposition is known to endure from early postnatal days (44,45,46) throughout the aging process (Jobe, unpublished observations). Behaviorally, these spontaneous seizures are identical to those known to be determined by brainstem seizure circuitry, and are therefore believed to provide an index of brainstem seizure predisposition. Predisposition of the brainstem seizure circuitry to putative spontaneous GTCSs is evident in GEPR-9s even in the absence of intact neuronal connections to the forebrain (47,48,49,50). Following a complete precollicular transection, the fraction of GEPR-9s that exhibits spontaneous GTCSs is approximately double that of nonepileptic control rats. Moreover, the spontaneous seizures following precollicular transection are more severe in GEPR-9s than in nonepileptic controls. The incidence of spontaneous seizures in transected GEPR-3s is approximately equal to that observed in nonepileptic controls.
Predictable GTCSs, in response to hyperthermia, occur in intact adult GEPR-9s, but not in nonepileptic controls (51). Whether GEPR-3s are susceptible to seizures induced by elevated body temperature is unknown. The mechanism of hyperthermic seizure initiation in the GEPR has not been subjected to scientific inquiry. Essentially, all developing and adult GEPR-3s and GEPR-9s, but not normal mammals, are susceptible to sound-induced brainstem-determined GTCSs (45,52,53). Some immature and adult GEPRs have been observed to exhibit GTCSs that are associated with handling and/or postural alterations. These appear to occur unpredictably and infrequently (PC Jobe, unpublished observations).
Forebrain-determined seizure predisposition in intact adult GEPRs is evident in studies showing that the rostral circuitry is susceptible to activation by brainstem-determined seizures (45). Such caudal-to-rostral transition is rare to nonexistent in nonepileptic control rats. Ontogenetic studies show that from postnatal days 15 through 30, putatively seizure naive GEPR-3s exhibit secondary forebrain-determined seizures in response to brainstem-determined seizures (45). Secondary forebrain seizures do not occur in untreated, developing GEPR-9s.
Secondary forebrain seizures have also been detected in adult GEPRs subjected to brainstem seizure repetition. Two stimulation protocols have been used in pertinent studies. In one protocol, sound-induced brainstem seizures were induced once daily for up to 24 days (54). In the other, three brainstem seizures were induced each day (55). Data from these experiments suggest that two mechanisms are operative in the transition from brainstem- to forebrain-determined seizure activity. First, severe brainstem-determined seizures have a limited capacity to provide protection against forebrain-determined seizures. As a result, forebrain-determined seizures do not become evident in the once-daily paradigm until at least 20 days of stimulation have been delivered. Secondly, we suggest
that brainstem seizures kindle components of the forebrain. The appearance of forebrain-determined seizures in the 3-per-day study provides evidence for this hypothesis. Accordingly, we suggest that in the 3-per-day study, forebrain kindling was sufficient to overcome anticonvulsant effects exerted on this circuitry by severe brainstem seizures. Experimentally induced alterations in brainstem seizure severity in the repetition paradigms confirmed these hypotheses.
that brainstem seizures kindle components of the forebrain. The appearance of forebrain-determined seizures in the 3-per-day study provides evidence for this hypothesis. Accordingly, we suggest that in the 3-per-day study, forebrain kindling was sufficient to overcome anticonvulsant effects exerted on this circuitry by severe brainstem seizures. Experimentally induced alterations in brainstem seizure severity in the repetition paradigms confirmed these hypotheses.
Another factor may influence the occurrence of forebrain-determined seizures in response to brainstem-determined seizures: the duration of the brainstem-determined seizure. Essentially, all recordings show that brainstem seizure repetition increases the duration of the set of spike-wave complexes that occurs following the electrodecremental interval in class 9 brainstem seizures (49,54). Therefore, the interval between the initial high-amplitude spikes and spike-wave complexes increases dramatically with repetition, as does the interval between the initial spikes and the onset of forebrain-determined seizures. Perhaps, the anticonvulsant properties of the brainstem-determined seizure arise from the initial high-amplitude activity at the beginning of the brainstem seizure and dissipate during the long interval of postelectrodecremental spike-wave activity that is developed through brainstem seizure repetition.
The cortical EEG of class 9 and 10 brainstem-determined seizures in GEPR-9s corresponds very closely to the morphology of EEGs recorded from the caudal circuitry in fully generalized brainstem-determined seizures (34,49,50,56). Accordingly, the EEG begins with high amplitude spikes and spikes waves with a sudden transition to an electrodecremental interval. During the tonic extensor component of the behavioral seizures, this very low amplitude activity evolves into repetitive spike-wave complexes that progress to higher amplitudes and lower frequencies. With this increasing amplitude, progressive increases occur in the amplitude of clonic behaviors superimposed on generalized tonic rigidity. In the absence of the onset of a forebrain-determined seizure, the spike-wave complexes gradually decrease in amplitude until devolving into the postictal stage. However, with the secondary onset of a forebrain-determined seizure, the cortical EEG observationally changes morphology with different events occurring in association with different classes of forebrain-determined seizure. Quantitative analyses of the cortical EEGs before, during, and after the transition to forebrain-determined seizure activity may reveal characteristic distinctiveness in the tonic-clonic spike-wave complexes driven by brainstem-determined seizures versus the clonic behaviors characteristic of forebrain circuitry. Future studies may determine the exact mechanisms by which brainstem seizures act to preclude and initiate the expression of the forebrain-determined seizures.
Exaggerated Seizure Responsiveness and/or Abnormally Low Thresholds
Many convulsant modalities produce seizures in both GEPRs and nonepileptic mammals. However, GEPRs are distinguished by abnormally low thresholds or exaggerated seizure responsiveness to many of these convulsant challenges, including aminophylline (57), minimal and maximal electroshock (58), flurothyl (44), hyperbaric conditions (59), pentylenetetrazol (58), and the barbiturate abstinence syndrome (60). Additional evidence for seizure predisposition in the forebrain and brainstem seizure circuitry is also evident in the exaggerated responsiveness to limbic kindling seizures. Both GEPR-3s and the GEPR-9s exhibit limbic kindling acceleration, with the fastest rate occurring in the GEPR-9s (61,62,63). In GEPR-9s, class 1 through 5 kindling seizures initiated by an electrical stimulus in the amygdala secondarily ignite GTCS, a behavior that is determined by activation of the brainstem seizure circuitry (63). These secondary seizures in GEPR-9s are unlike those in the Racine classification scheme (64). Rather, they are identical to those of severe brainstem convulsions (e.g., class 6 through 9) as defined in the severity classification system of Jobe et al. (3,65).
With typical kindling paradigms, nonepileptic control rats do not appear to exhibit secondary brainstem convulsions. Indeed, only after an extreme schedule—of more than 550 stimulations at the rate of 15 times weekly (66)—do nonepileptic rats begin to exhibit even the mildest form of brainstem seizure (class 1 according to the severity scale of Jobe et al.) (3,65). In GEPR-9s, the secondary brainstem-determined seizures have a tendency to occur during the after discharge in the amygdala. However, in some instances, brainstem seizures occur up to 60 seconds following the termination of the after discharge. During the interseizure interval, the EEG in the amygdala is usually characterized by low-amplitude, low-frequency waves with occasional spikes.
With typical kindling paradigms, nonepileptic control rats do not appear to exhibit secondary brainstem convulsions. Indeed, only after an extreme schedule—of more than 550 stimulations at the rate of 15 times weekly (66)—do nonepileptic rats begin to exhibit even the mildest form of brainstem seizure (class 1 according to the severity scale of Jobe et al.) (3,65). In GEPR-9s, the secondary brainstem-determined seizures have a tendency to occur during the after discharge in the amygdala. However, in some instances, brainstem seizures occur up to 60 seconds following the termination of the after discharge. During the interseizure interval, the EEG in the amygdala is usually characterized by low-amplitude, low-frequency waves with occasional spikes.
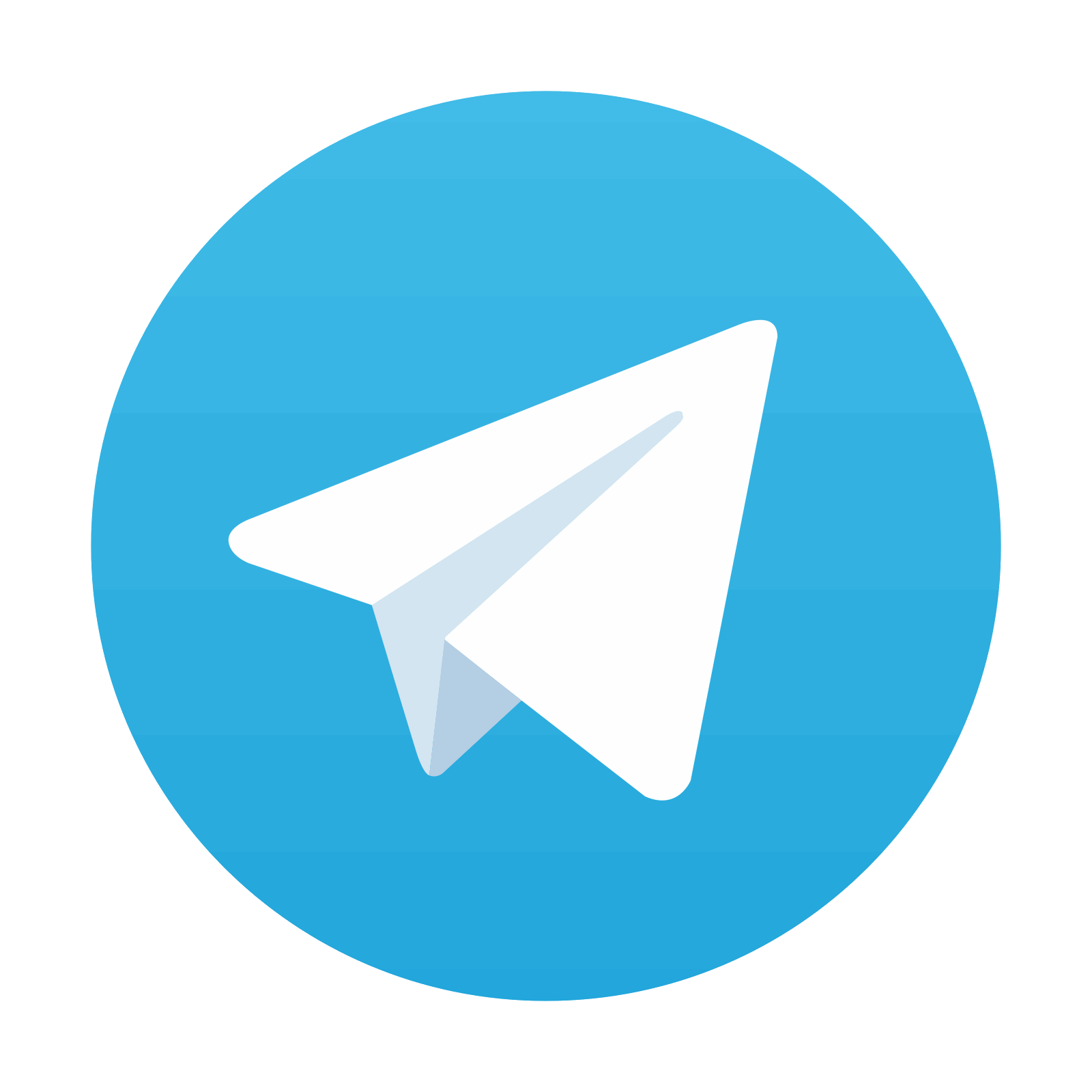
Stay updated, free articles. Join our Telegram channel

Full access? Get Clinical Tree
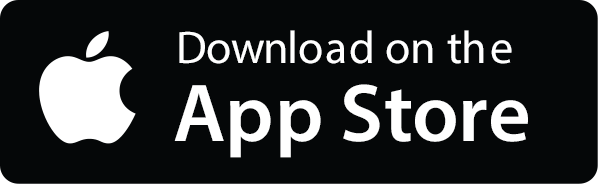
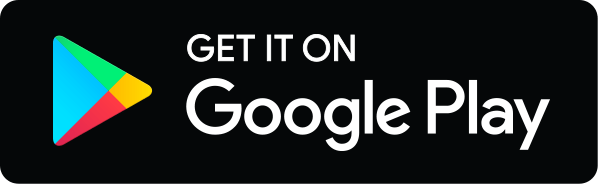
