Prescription of anticholinergic treatment used by Professor Charcot, dated 1887 from the College of Physicians of Philadelphia Library.
The cholinergic system and Parkinson’s disease
Some authors have postulated the theory of imbalance between acetylcholine and dopamine in the striatum as the origin of motor symptoms in PD [1, 5]. The dopaminergic and cholinergic systems are closely related and in constant balance. In PD, the degeneration of nigral neurons leads to decreased dopamine production, and this situation cause an imbalance between acetylcholine and dopamine. In PD, dopamine depletion blocks autoinhibition of acetylcholine release through muscarinic autoreceptors, leading to excessive acetylcholine release [5]. This hypothesis is supported by the fact that anticholinergics were the first treatment for PD [6].
The striatum is a nodal basal ganglia structure and is a major input station of the basal ganglia. Acetylcholine is an important neurotransmitter in the striatum based on its abundance [7]. The striatum is subdivided into two regions, the matrix and the striosomes. Both compartments contain medium spiny neurons, which comprise nearly 95% of striatal neurons, and affect the direct and indirect pathways [8, 9]. The remaining striatal neurons are interneurons and only 1–3% are giant aspiny cholinergic interneurons. These interneurons have multifold arborizing axons [10, 11], and this explains the high expression of cholinergic markers in the striatum [7]. In the striatum, acetylcholine acts via muscarinic and nicotinic receptors, in postsynaptic and presynaptic targets, and contributes to the activity of the medium spiny neurons [12]. The primary effect of acetylcholine on medium spiny neurons is depolarization via muscarinic receptor M1. This facilitation occurs primarily on medium spiny neurons of the indirect pathway [13].
Multiple studies in PD have shown loss of cholinergic neurons in the pedunculopontine nucleus (PPN) in PD [14–16]. The PPN is located in the mesencephalic tegmentum and contains cholinergic, GABAergic and glutamatergic neurons [17]. Effects on the PPN in PD are narrowly related to gait disturbance, and the severity of cholinergic neuronal depletion in the PPN correlates with the severity of motor symptoms [14]. Another cholinergic system change in PD is an increase in the muscarinic binding sites in the globus pallidus internus, probably due to a compensatory upregulation in response to a decrease in cholinergic activity [18]. These changes result in elevated peaks of acetylcholine in the striatum, and may affect the indirect pathway [5]. Some authors believe that anticholinergics can correct this imbalance in PD, thereby reducing the degree of neurotransmission mediated by neostriatal acetylcholine [19].
Nicotinic receptors also play a role in PD. Nicotinic receptors are located at presynaptic terminals. Substantial evidence from studies of epidemiologic and animal PD models suggest a protective effect and an inverse relationship on the development of PD [20]. However, clinical trials have not shown similar results [21, 22].
Pharmacokinetics and pharmacodynamics
The anticholinergic drugs available for use in the treatment of PD provide a different mechanism of action, which is an alternative to relieve some of the bothersome motor symptoms, especially tremors.
The anticholinergic drugs used in the treatment of PD are competitive antagonists of muscarinic receptors. The most commonly used worldwide are trihexyphenidyl (Artane®), benztropine (Cogentin®), biperiden (Akineton®) and procyclidine (Kemadrin®).
The mechanism of action of anticholinergics is still not fully known but is suspected to be a result of blocking of the muscarinic receptors in the striatum, acting as strong inhibitors of the presynaptic carrier-mediated dopamine transport mechanism [23]. In addition to the muscarinic blockade, anticholinergics act as agonists at the noradrenergic synapse, and also have N-methyl-d-aspartate (NMDA) antagonist receptor activity [24].
In general, most anticholinergic drugs have good and rapid oral absorption through the gut. The average the time to maximal plasma concentration (Tmax) is 2.5 h (1.5–3.5 h). The maximum plasma concentration (Cmax) is dose dependent and is different for each drug, with the exception of procyclidine as its plasma concentration is high even in small doses [25]. The half-life (t1/2) is also different in each drug; for example, trihexyphenidyl has a Cmax of 7μg/l for a 10mg dosage with a t1/2 of 33 h, and biperiden has a Cmax of 4–6μg/l for 4mg dosage with a t1/2 of 18–24 h. The results for these and the other two commonly used drugs are illustrated in Table 2.1.
Properties of major anticholinergics used in Parkinson’s disease
Drug | Cmax (μg/l) for dose (mg) | Tmax (h) | t1/2 (h) | Preparationsa | Initial daily dosage | Titration | Final daily dosage |
---|---|---|---|---|---|---|---|
Trihexyphenidyl | 7 (10) | 1.3 | 33 | 2–5mg 0.4mg/ml | 1mg | Increase 2mg every 4–5 days | 6–10mg (2–3mg tid) |
Biperiden | 4–6 (4) | 1.5 | 18–24 | 2mg 5mg/ml | 1mg | Increase 1mg every 4 days | 6–9mg (2–3mg tid) |
Benztropine | 2.5 (1.5) | 7 | 0.5, 1, 2mg 1mg/ml | 0.5mg | Increase 0.5mg every 5–6 days | 2–6mg (1–2mg tid) | |
Procyclidine | 116 (10) | 1 | 8–16 | 2.5–5mg 2.5mg/5ml | 2.5–3mg | 15–30mg (5–10mg tid) |
The bioavailability of anticholinergics ranges from 30 to 90%; for example, procyclidine and biperiden have an absolute bioavailability of 75% and 33%, respectively [25, 26]. The tissue distribution of anticholinergics has been observed in laboratory animals and has been reported to be large. For biperiden, a brain/plasma area-under-the-curve ratio of 7–12 ng/(hml) has been reported [27]. These authors also showed that with an intravenous infusion of biperiden, maximal brain concentration was obtained within 3–10 min. The high concentrations of these drugs in the brain could be explained by their intensely lipophilic properties. Lipophilic biomolecules in the brain serve to promote the uptake of anticholinergics, and for trihexyphenidyl and biperiden, the uptake is also related to intralysosomal uptake of the drug [28]. The protein binding of anticholinergics has not been elucidated in humans, but in rats the binding of biperiden to plasma protein is approximately 90% [29].
For the most part, all anticholinergics are metabolized in the liver, and the pathways of excretion are urine and bile. There are some differences for each of these drugs. For trihexyphenidyl and procyclidine, the metabolism is due to hydroxylation of the alicyclic groups, and excretion is through urine and bile for trihexyphenidyl and through urine for procyclidine [30]. Benztropine is metabolized by N-oxidation, N-dealkylation and ring hydroxylation [31].
The dosage and titration vary for each drug (Table 2.2), but in general, to avoid the adverse effects of these kinds of medication, initiation of treatment should be with low dosages and slow titration, especially in the older population.
Commonly used drugs with secondary anticholinergic effects
Name of drug | Use |
---|---|
Diphenylhydramine Ipratropium bromide Clozapine Quetiapine Olanzapine Amitriptyline Nortriptyline Paroxetine | Antihistamine Bronchospasm Antipsychotic Antipsychotic Antipsychotic Antidepressant Antidepressant Antidepressant |
Beyond the anticholinergic drugs, there are many others drugs used for different conditions in PD, especially psychiatric symptoms (such as depression, psychosis and hallucinations), as well as drugs used for general conditions like allergies that also have anticholinergic properties acting on cholinergic receptors [32].
Current use of anticholinergic drugs in the management of Parkinson’s disease
While anticholinergic drugs were initially the most important drugs for treating PD, their use declined after the development of levodopa and most recently dopamine agonists. The occurrence of adverse effects also caused a decline in their use but to a much lesser extent. The current use of anticholinergics has been limited to the treatment of a PD resting tremor that is not responding satisfactorily to dopaminergics and PD-related dystonia [33]. One of the reasons beyond the advent of dopaminergic drugs and the presence of adverse events is the lack of clinical trials with anticholinergic drugs in recent years. The majority of studies in this class of drug were performed several decades ago, and most were conducted before the use of current clinical diagnostic criteria such as the UK Parkinson’s Disease Society Brain Bank criteria [34] for idiopathic PD, and also before the use of the Unified Parkinson’s Disease Rating Scale (UPDRS).
In the last Cochrane review of anticholinergics for symptomatic management of PD, the authors evaluated the efficacy and tolerability of anticholinergic drugs in the motor treatment of PD compared with placebo [35]. In the review, they included randomized controlled trials comparing anticholinergic drugs versus placebo or no treatment in new-onset or advanced PD, either as monotherapy or as adjunctive therapy. Initially, there were 14 potentially qualifying studies published from 1954 to 1986, and five were excluded for technical or methodological issues. The remaining nine studies, with a total of 221 patients participating, were included. The duration of the studies was between 5 and 20 weeks. Different drugs were investigated (benzhexol, orphenadrine, benztropine, bornaprine, benapryzine and methixine), and one study used two different anticholinergics drugs. All the studies reported improvement from baseline in at least one outcome measure with the exception of one study. Five studies reported both tremor and other parkinsonian motor manifestations as outcome measures, while the rest of the studies used disability and other parkinsonian signs but no tremor. All studies demonstrated anticholinergics to be better than placebo for improving motor symptoms as adjunct therapy or monotherapy. Neuropsychiatric and cognitive adverse events were described in six studies. Side effects, in particular cognitive and neuropsychiatric, were the most common reason for withdrawal. There is insufficient available data to compare efficacy or tolerability among anticholinergics.
There have been a few studies using anticholinergic drugs for PD in recent years, with most focusing on the management of sialorrhea and bladder dysfunction in PD. A randomized, double-blind, placebo-controlled study with ipratropium bromide in 17 patients with PD and troublesome drooling showed a mild improvement on a subjective measure of sialorrhea, without significant adverse events, although it did not affect objective measures of saliva production [36]. Most recently two randomized, double-blind, placebo-controlled crossover studies, one with glycopyrrolate for sialorrhea in 23 patients with PD, showed that 1mg three times daily of glycopyrrolate, an anticholinergic drug with poor permeability across the blood–brain barrier, is safe and effective for sialorrhea in PD [37]. Another pilot study comprised 19 patients taking a single dose of oral, slow-dissolving thin films containing tropicamide. The results showed that 1mg tropicamide resulted in a significant saliva reduction when compared with placebo, and without adverse events [38]. A 12-week dose-titration trial of controlled-release oxybutynin for neurogenic bladder concluded that controlled-release oxybutynin was safe and effective for this condition [39].
The first report of anticholinergics improving tremors was in 1949 [40], and this was followed by other studies confirming the benefits of anticholinergics [41, 42]. In our experience, we have seen improved tremor in similar PD patients with good tolerability, especially in young PD patients (under 60 years old). In those patients with medication-refractory tremors, we believe it is a good option to try anticholinergics before considering surgical interventions, although there is no evidence to argue in favor of a preferential effect of anticholinergics on tremor rather than other motor symptoms [35].
Another use of anticholinergic drugs in PD is to treat dystonia. Dystonia in PD, which occurs in different stages of the disease, is common in the early stage of young-onset PD and is most often seen as either a “wearing off” or a dyskinetic phenomenon, especially at peak dose, but also as biphasic dyskinesia in advanced PD [43]. End-of-dose dystonia is present most often as an early-morning and generally painful foot dystonia but can involve any part of the body. Dystonias as a dyskinetic phenomenon are most common as a facial dystonia. Anticholinergics are useful in the treatment of dystonia, especially DYT1 dystonia [44]. For PD dystonia, there have been no randomized trials with anticholinergics. There is a report of benefit from anticholinergics in wearing off of foot dystonia [45].
The benefits of anticholinergics to treat axial symptoms in PD were reported in a few trials [42, 46, 47]. A recent study showed that the anticholinergic agent trihexyphenidyl improved axial symptoms after deep-brain stimulation of the subthalamic nucleus [48]. Only a few reports have been published comparing dopaminergic therapy and anticholinergics directly with objective measures. In a small study, Koller [49] compared trihexiphenidyl and carbidopa/levodopa taken for 2 weeks and found improvement of tremors. Another study by Parkes et al. [50] demonstrated improvement of resting tremor, rigidity and bradykinesia with levodopa when compared with benzhexol. There was a more robust improvement of rigidity and tremors using levodopa after 6 months but only mildly after using benzhexol. Akinesia responded better to levodopa, with only a negligible improvement with benzhexol. In another single-dose study, Schrag et al. [51] compared biperiden intravenously and apomorphine on two consecutive days and found that both drugs were effective in reducing tremor in PD, although without evidence for selective anticholinergic responsiveness of parkinsonian tremor.
It is still unclear if there is a synergistic effect between levodopa and anticholinergics. One study found that combining anticholinergic drugs with levodopa could affect the profile of the levodopa concentration in plasma. Levodopa absorption was reduced because there was a delay in gastric emptying of levodopa, but the fluctuation in the plasma concentration was less after anticholinergics, which could be beneficial in fluctuating patients [52]. This synergistic effect has been reported by others authors [47, 50]. However, other reports found that the chronic use of anticholinergic agents may have a negative impact in levodopa absorption [53]. The same authors commented that patients stabilized on levodopa often present a severe deterioration after withdrawal of anticholinergics. In these patients, addition of benzhexol will produce improvement [54].
Adverse effects and contraindications of anticholinergic drugs
As we know, anticholinergics act by blocking the muscarinic receptor, in both the central and peripheral nervous systems; hence, they may be responsible for peripheral or nonneurological and central or neurological side effects (Table 2.3). Current evidence has shown that anticholinergics, when compared with other antiparkinsonian drugs, are associated with a higher risk of adverse effects, especially in the elderly, who seem to be more susceptible. Many factors may contribute to this, including increased permeability of the blood–brain barrier, pharmacodynamic changes related to aging, the fact that the aging brain is particularly sensitive to these medications, and the risk of polypharmacy and drug–drug interactions [55]. Although the use of anticholinergics in PD is low, there are many other drugs with anticholinergic properties (e.g. antidepressants, amantadine) [32] that PD patients receive that may cause anticholinergic side effects, mainly neurological side effects. These side effects may be uncomfortable for younger patients, but the effects in older patients could be devastating. The central adverse effects could be a serious problem too. Cognitive impairment and dementia are common problems observed in patients with PD [56]. Cholinergic deficits play an important role in the cortical neurochemical alterations in cognitive impairment [57]. Central anticholinergic side effects may be subtle but bothersome, such as sedation, the inability to concentrate and confusion, or more severe, such as agitation, hallucinations and cognitive decline. Ehrt et al. [58] reported a significant association of anticholinergic properties and the incidence of cognitive decline in a large cohort of PD patients followed for 8 years, with a rate of cognitive decline 6.5 times higher in the group using drugs with anticholinergic properties compared with those who were never exposed to them. The cognitive issues induced by anticholinergics are more related to executive function [59] and short-term memory [60].
Common side effects and contraindications of anticholinergics
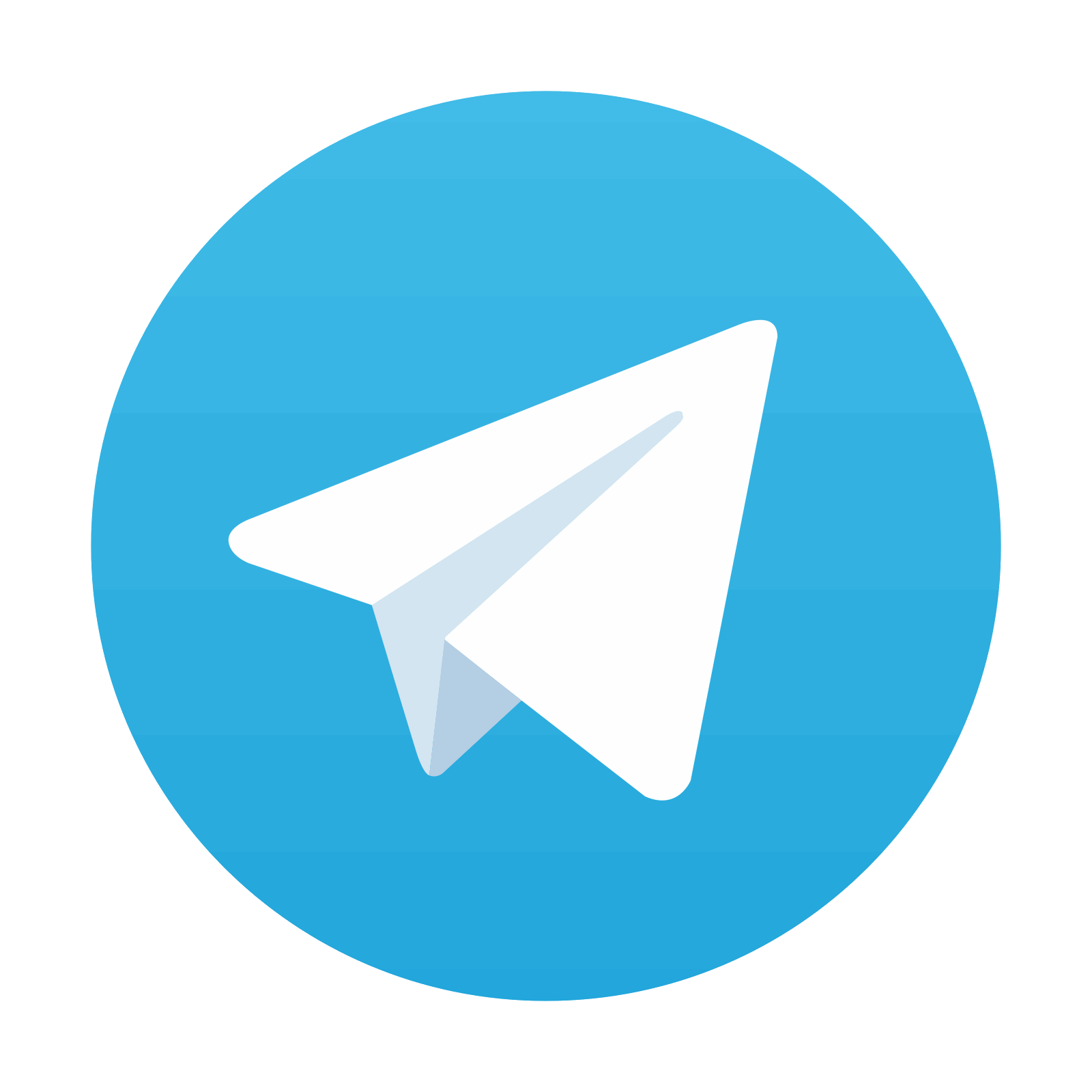
Stay updated, free articles. Join our Telegram channel

Full access? Get Clinical Tree
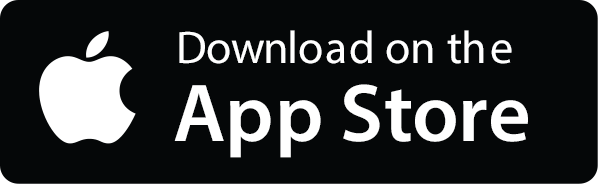
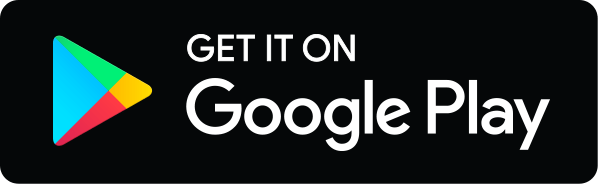