A 53-year-old man suffered a severe traumatic brain injury after being struck in an automobile-pedestrian accident. He was intubated in the field and stabilized in the emergency department (ED). He has a previous history of a hernia repair (15 years) and no pertinent social history. His admission height and weight are 71 inches and 80 kg, respectively. He remains stable until hospital day 7 when a leukocytosis develops (WBCs 10.6 to 15.7 cells/mm3), and he was febrile overnight (Tmax, rectal 101.2°F) and develops macroscopically cloudy urine. The serum creatinine level rose from baseline 0.8 mg/dL to 2.1 mg/dL. A Foley catheter is in place. Serum lactate concentration is at 4.4 mmol/L His morning chest radiograph is unremarkable, showing an endotracheal tube in good position. Urinalysis is reported as cloudy with WBC clumps, 30 WBCs, and positive leukocyte esterase. Arterial blood gas levels on an inspiratory oxygen concentration of 40% are as follows: pH 7.31, Paco2, 36 mm Hg; Pao2, 76 mm Hg; and HCO3–, 20 mEq/L; blood pressure (BP), 91/50 mm Hg (mean arterial pressure [MAP], 64 mm Hg); heart rate (HR), 109 bpm; respiratory rate (RR), 29 bpm. The team diagnoses this patient with severe sepsis and activates the institution’s severe sepsis pathway. They draw blood and urine cultures, initiate fluid resuscitation with a normal saline bolus of 30 mL/kg, and empiric antibiotics are started.
What factors should be considered when selecting appropriate empiric antimicrobial therapy for this patient?
Selecting appropriate empiric antibiotics for a suspected infection is the focus of this chapter. As you prepare to initiate antimicrobial therapy for this patient, applying a systematic approach will lead to choosing antimicrobials more wisely. The clinical and laboratory findings suggest a catheter-associated urinary tract infection (CA-UTI) that has developed into severe sepsis. The patient’s end-organ dysfunction is evidenced by a serum creatinine level of 2.1 mg/dL and serum lactate concentration of 4.4 mmol/L. The overall plan of therapy includes volume resuscitation, elimination of the causative pathogen, clinical stabilization, and symptom resolution. Samples for culture should be taken from all suspected sources including two sets of blood cultures. Doing so prior to antibiotic initiation prevents false negatives, enabling much needed antimicrobial stewardship efforts to take place. Also, having blood cultures to guide therapy once empiric antibiotics are started enables clinicians to mindfully adjust or de-escalate therapy over time. If samples yield growth of an organism, then modifications to the regimen can be made in order to minimize unnecessary drug exposure and reduce the risk for developing resistance.
Early initiation of antibiotics is critical to the survival of patients with severe sepsis and septic shock. Kumar et al showed that mortality increases by 7% every hour antibiotics are delayed from the time of hypotension onset.1 Moreover, this relationship between antibiotic delays and mortality risk has been demonstrated in several other studies looking at sepsis of various etiologies.2-4 All this has led to the recommendation in the International Guidelines for the Management of Severe Sepsis and Septic Shock that antibiotics be administered within 1 hour of onset.5 Consensus guideline statements for other infectious diseases make similar recommendations for the timing of antibiotic administration.6-8
Antibiotic activity is determined by numerous factors. Antibiotics with little or no in vitro activity against suspected pathogens are considered inappropriate empiric antibiotic therapy and serve as an independent mortality risk factor in critically ill patients with infections.9,10 Using a systematic approach to account for specific factors (infection, host, antibiotic, and hospital) increases the likelihood of choosing empiric antibiotics that will provide the greatest likelihood for positive treatment outcomes (Figure 54-1).
Infection-specific factors such as source and tissue penetration are important considerations when selecting a regimen. Determining the infectious source is very helpful when deciding which drug, out of many, is reasonable. Intuitively, the level to which antimicrobials penetrate source tissue affects the success of treatment. To prevent resistance and ensure the selected medication is able to carry out its bactericidal or bacteriostatic effects, the antimicrobial must reach the site of infection in a biologically active form. Understanding the relationship between susceptibility data, tissue penetration, and clinical outcomes is also important. An example of this is the reported susceptibility of tigecycline and amikacin for an extended-spectrum β-lactamase (ESBL) producing Klebsiella in a patient requiring treatment for a CA-UTI. Although few options remain for the treatment of ESBL infections, choosing tigecycline in this case would be inappropriate, as it does not concentrate well in the urine. Drug penetration into deep tissues such as liver abscesses may also pose difficulties because enzymes and pH can alter the activity of some drugs at the site of infection.
Sampling suspected infectious sources (blood, urine, sputum, CSF, and wound) for culture and sensitivity prior to initial antibiotic administration offers clear advantages in achieving therapeutic success. False-negative culture results and changes in the constitution of sampled fluids are reasons why early cultures are important, especially in the treatment of meningitis, pneumonia, and UTIs.11,12 Ideally, 2 sets of blood cultures from different sites should be taken 1 hour apart. Each set should have one aerobic and one anaerobic bottle. In most ideal cases cultures should be collected at the time of fever onset, except in diseases such as endocarditis where cultures can be collected at any time because growth is considered qualitative-continuous.13
Patients reporting a particular drug allergy should normally not receive that medication again. One of the most common factors to consider is what and when alternatives should be used in a penicillin-allergic patient. Patients frequently mistake common intolerances such as GI upset as allergic reactions.14 Inaccurate or incomplete documentation of these reactions can also lead the prescriber to order an alternative when, in fact, it is not necessary. There are limitations to the clinical studies that looked at the risk of administering cephalosporins to penicillin-allergic patients, but it appears that < 5% of skin-test positive, penicillin-allergic patients will have an allergic reaction to cephalosporins.15-17 Because skin testing is often unavailable, it is generally acceptable in these cases to give cephalosporins when patients report a history of delayed skin reaction (eg, rash). Patients who provide a good history of immediate immunoglobulin E (IgE)-mediated hypersensitivity reactions (eg, anaphylaxis, laryngospasm, angioedema, hypotension) to penicillins should generally not receive cephalosporins.18 Monobactams (eg, aztreonam) and fluoroquinolones (eg, moxifloxacin) are examples of alternatives in the setting of IgE-mediated penicillin allergies. Antibiotic desensitization may be necessary in cases where there are no alternatives and the patient must receive the medication despite a history of an allergic reaction. Antibiotic desensitization is a labor-intensive process because it requires administration of small aliquots of drug that are doubled every 15 minutes until the therapeutic dose is achieved.19 Timeliness of antibiotic administration is very important, for every hour antibiotics are delayed, mortality in septic shock increases by 7%.20 Therefore, class alternatives should be chosen in patients with a history of an IgE-mediated drug allergy to minimize delays in antibiotic administration.
Failure of therapy due to drug resistance is a major consideration when choosing appropriate antibiotics. It is important to differentiate between the two general types of resistance exhibited by organisms: intrinsic and acquired. Intrinsic resistance refers to a characteristic trait found across a certain bacterial species. For example, enterococci species are intrinsically resistant to cephalosporins because of their production of penicillin-binding proteins, rendering that drug class ineffective. Acquired resistance refers to an adaptation in the genetic arrangement of a pathogen resulting in partial or complete resistance to an antimicrobial that was once effective.21 Prior antibiotic exposure is associated with the increased rate of infections due to methicillin-resistant Staphylococcus aureus (MRSA), vancomycin-resistant enterococci, and ESBL-producing (extended-spectrum β-lactamase) Escherichia coli and Klebsiella species.22,23 The rise in microbial resistance rates is thought to be due to a variety of factors, including persistent overutilization of antimicrobials in hospitals and the community. Another contributing factor may be the rise in immunodeficient patients receiving long-term prophylactic antimicrobial therapy for prevention of infections, such as the use of trimethoprim and sulfamethoxazole for the prevention of pneumocystis pneumonia in HIV patients. To encourage rational antimicrobial choices, hospital and regional susceptibility patterns should be monitored using local antibiograms.21 When choosing an empiric regimen, it is also important to avoid antibiotics the patient received in the previous 2 weeks because the pathogen may have developed resistance.6 E coli and Klebsiella are two bacteria among these species with ESBL genes that demonstrate β-lactam antibiotic resistance.24,25 Carbapenemase-producing strains of Pseudomonas aeruginosa and Klebsiella may also cause infections resistant to imipenem or meropenem.26 Klebsiella pneumoniae carbapenemase (KPC) organisms are resistant to all β-lactam antibiotics and if encountered, consideration should be given to therapy with colistin or tigecycline.27,28 Table 54-1 contains a list of important risk factors for multidrug-resistant organisms (MDROs) that cause community-acquired, healthcare-associated, hospital-acquired, and ventilator-associated pneumonias that should be considered during the antimicrobial selection process.6
|
Among critically ill patients there are numerous comorbidities that should be considered when choosing an antimicrobial regimen since many are predisposing to specific infectious diseases. Comorbidities can affect the choice of therapy as certain disease states provide an environment suitable for the growth of specific bacterial species. For example, the most common causes of community-acquired adult bacterial meningitis are Streptococcus pneumoniae and Neisseria meningitides. Some predisposing conditions put patients at an increased risk for other organisms, such as Listeria monocytogenes. Patients suspected of having meningitis in the setting of impaired cell-mediated immune compromise (ie, cancer, organ transplantation, corticosteroid therapy, AIDS, age) are at an increased risk for infection with L monocytogenes and should thus receive additional appropriate antibiotic coverage (ie, ampicillin 2 g q4h).29-34 Nosocomial meningitis occurs most commonly among patients having recently undergone a neurosurgical procedure, carrying a reported meningitis incidence of 0.3% to 1.5%.35-37 Nosocomial meningitis is typically caused by staphylococci and aerobic gram-negative bacilli organisms (ie, Listeria); therefore, antibiotic choices should include coverage for these organisms.
Other diseases predispose patients to specific infections, prompting coverage for the most likely causative pathogens. This is illustrated among patients with chronic pulmonary disease or cystic fibrosis who are at risk for recurrent respiratory infections. Another example includes patients with vascular insufficiency due to diabetes mellitus. This population is at an increased risk for developing soft tissue infections of the lower extremities.
Comorbidities can significantly influence drug levels and their resulting effects within the body. Because patients in the intensive care unite (ICU) often present with extensive past medical histories, it is important to consider how their concomitant disease states may impact antibiotic selection.
Renal failure is one of the most common comorbidities to consider when choosing an empiric antibiotic regimen. Reduced drug clearance and increased plasma levels should be anticipated when treating patients with many commonly used antibiotics (ie, aminoglycosides, β-lactams, carbapenems, daptomycin, fluoroquinolones, and vancomycin).
The same principle can be applied to patients with hepatic failure receiving treatment with clindamycin, linezolid, and metronidazole. However, the degree to which drug clearance is affected is less well established.
Obesity has the potential to increase drug volume of distribution and lead to variable plasma levels. This is especially true in patients receiving β-lactams, vancomycin, fluoroquinolones, carbapenems, and aminoglycosides.
Critical illness such as septic shock can cause unpredictable antibiotic plasma levels through increased volume of distribution and reduced renal and hepatic drug clearance.
A host’s ability to mount an adequate immune response is important when considering which organisms to cover. Immunosuppressive illness (ie, untreated AIDS or neutropenia secondary to chemotherapy) confers a risk for opportunistic infections not found in healthy immune-competent adults. Organisms such as P aeruginosa and Acinetobacter baumanni are normally commensal bacteria. However, drug-resistant gram-negative bacteria are increasingly causing more infections among the immunodeficient population.38 Identifying patients at risk for these highly resistant organisms requires careful interpretation of individual culture results as well as local and institutional antibiograms. Antibiotic stewardship includes coordinated interventions designed to improve the appropriate use of antimicrobials by promoting the selection of the optimal antimicrobial drug regimen, dose, duration of therapy, and route of administration. Judicious utilization of vancomycin with empiric antibiotic regimens is also important because its overuse is connected to the development of drug-resistant organisms such as Enterococcus species and S aureus.39-42 Coagulase-negative Staphylococcus is not an aggressive pathogen, but it is frequently identified as the cause of bacteremia in neutropenic patients. Because coagulase-negative staphylococci are not associated with rapid clinical decline, there is usually no compelling need to treat such infections with vancomycin at the time of fever onset,43 nor should treatment be initiated for one out of two blood cultures positive for coagulase-negative staphylococci. Table 54-2 lists circumstances in fever and neutropenia where treatment with a broad gram-positive antibiotic should be considered.38
|
Incorporating principles of pharmacodynamics, pharmacokinetics, and microbiological data has become more critical than ever. Emergence of resistant organisms across healthcare provision in community settings is rising, creating new challenges for providers.44 Understanding antimicrobial pharmacodynamics (ie, susceptibility rate, extent of microbial killing activity, and postantibiotic effects) provides a more prudent approach for deciding the optimal dose and interval of antimicrobials than traditional approaches that concentrate only on pharmacokinetics.45
Early research and recommendations have focused on antimicrobial time-course and concentration measurements in various tissues, serum, and body fluids. However, contemporary approaches apply data that illustrate patterns of antimicrobial activity over time against particular species (ie, local antibiograms), which can provide useful information when choosing a drug regimen. For example, E coli is demonstrating increased resistance rates of up to 30% to 50% compared with traditional drug therapies such as ciprofloxacin and ampicillin/sulbactam, which would not be preferred agents in patients with more serious infections.
Antimicrobials display two basic types of killing activity: concentration-dependent killing and time-dependent killing. Concentration-dependent killing refers to drugs where higher concentrations result in an increased rate and degree of microbial killing (ie, aminoglycosides, fluoroquinolones, amphotericin B).46-49 Extended-interval aminoglycoside dosing regimens (eg, 6 mg/kg once daily) were developed to take advantage of this killing activity, while minimizing toxicity. High rates of clinical success are observed when aminoglycosides dosages are determined to provide a peak minimum inhibitory concentration (MIC) ratio of 8 to 10 in severe gram-negative infections including nosocomial pneumonia.50,51 Although extended-interval aminoglycoside dosing raises peak serum levels, this dosing strategy may also decrease the risk of nephrotoxicity and ototoxicity associated with these drugs. Renal tubular cell and middle ear endolymph uptake of aminoglycosides is more effective with low-sustained concentrations, leading to potentially toxic tissue concentrations compared with high intermittent levels. This supports the evidence that extended-interval dosing has a lower probability of causing nephrotoxicity than traditional dosing.52-54
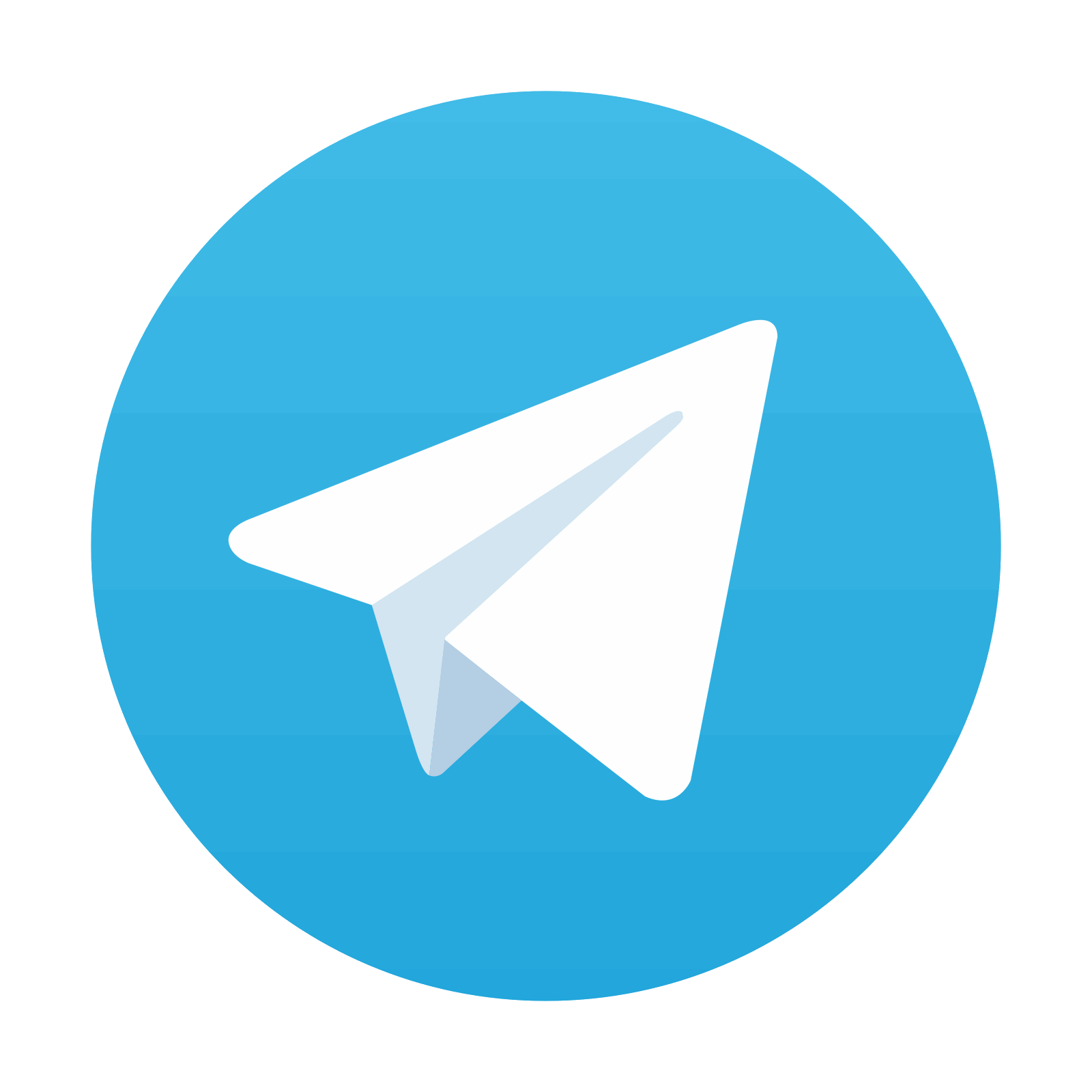
Stay updated, free articles. Join our Telegram channel

Full access? Get Clinical Tree
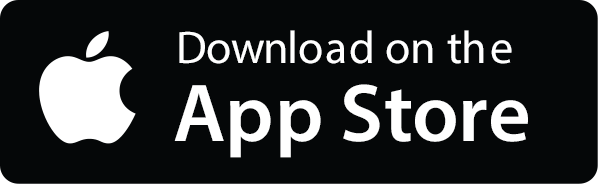
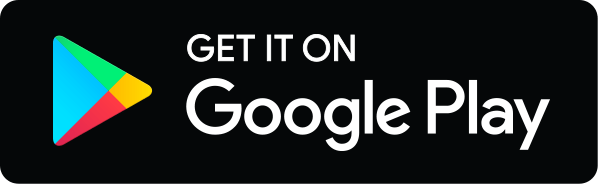