Summary of Key Points
- •
The spinal column consists of the occipitocervical junction and subaxial spine.
- •
Complex ligamentous structures constrain excessive translation and rotation in the occipitocervical junction and, to a lesser extent, the subaxial spine.
- •
The cervical disc space is created by the interval between adjacent superior and inferior vertebral end plates.
- •
The ventral and dorsal roots both traverse the intervertebral neural foramen above their same-numbered pedicle.
- •
The spinal cord is perfused by a single anterior spinal artery (ASA) and paired posterior spinal arteries (PSAs).
- •
The vertebral arteries lie in close proximity to the cervical spinal cord and run through the foramen transversarium, entering this structure most commonly at C6 (87.5%).
- •
Several structures are readily visible or palpable along the ventral neck and serve as landmarks for underlying structures including the hyoid (C3), thyroid cartilage (C4), and cricoid cartilage (C6).
The cervical spine is composed of seven vertebral bodies, spanning the interval between the occiput, rostrally, and the thoracic spine, caudally. Individual segments are connected by an extensive complex of viscoelastic structures to form a single flexible but constrained column serving both neuroprotective and structural functions. A thorough understanding of the normal relationships between these osseous, discoligamentous, and neural elements is essential for safe and effective surgical intervention. These relationships, moreover, have important implications on the biomechanical and clinical presentation of spinal pathology.
Spinal Column
The cervical spine may be divided into two morphologically distinct zones: the occipitocervical junction and the subaxial spine. These zones are discussed separately to facilitate more detailed examination of their unique osseous and discoligamentous structures.
Occipitocervical Junction
Osseous Elements
The first and second vertebral segments attach to the occiput to form the craniocervical junction, which is a complex articular system permitting rotational and nutational movement. The first cervical segment (C1), or the atlas, is composed of a ventral and dorsal arch, joined laterally by symmetric lateral masses ( Figs. 8-1 through 8-3 ). The superior and inferior articulating surfaces of the lateral mass are concave to enable articulation with the occipital condyles, superiorly, and the shoulders of C2, the axis, inferiorly ( Figs. 8-4 and 8-5 ). The ventral arch forms a short bridge between the lateral masses with a dorsal articular surface forming a synovial joint with the odontoid process of C2 and an anterior tubercle ventrally to which the longus colli muscle attaches. The dorsomedial walls of the C1 lateral masses form a small tubercle, which serves as the attachment for the transverse atlantoaxial ligament. The dorsal arch of the atlas typically forms a posterior tubercle in the midline, which is a rudimentary equivalent to subaxial spinous processes. Caution should be exercised in dissection of this structure, as the dorsal arch may be incomplete. The dorsal arch is round in cross section in the midline and attenuates laterally to form a more flat surface where it attaches to the dorsal lateral masses. The superior surface of the lateral dorsal arch forms a groove known as the sulcus arteriosus on which the vertebral arteries run bilaterally; it is often thin or even dehiscent, and great care must be taken when exposing the inferior surface of the C1 lateral mass. The lateral masses are bounded laterally by short transverse processes that are fenestrated by the transverse foramen, or foramen transversarium . As in the subaxial spine, the vertebral artery traverses the transverse foramen of C1 before turning 90 degrees medially to run along the superior surface of the dorsal arch as described previously. This genu lies 1.5 to 2 cm lateral to midline, and dorsal dissection should seldom require exposure of this precarious region.





The second vertebral segment (C2), or axis (see Figs. 8-1 through 8-4 ), is a distinctive osseous structure. The odontoid process, a peglike rostral projection, forms a synovial joint with the atlas along its ventral border and allows the atlas to rotate on C2 with translational restriction created by a complex of ligamentous structures. The tip of the odontoid process serves as the attachment for the apical ligament, which connects C2 to the basion (the ventral lip of the foramen magnum). The tip is flanked by two bony prominences, which serve as the attachments for the paired alar ligaments. These ligaments span the divide between C2 and the occipital condyles. The transverse atlantoaxial ligament traverses the dorsal border of the odontoid process, which often carries a groove for this strong ligament. The neck of the odontoid process narrows to meet the vertebral body of C2 and is a common site for C2 fracture. The vertebral body culminates rostrally to form two bilaterally symmetric “shoulders,” which flank the odontoid process and articulate with the lateral masses of C1. A relatively long pars interarticularis spans the interval between superior and inferior articulating processes and is laterally bounded by the transverse process of C2. The disproportionate length of the C2 pars interarticularis has important clinical implications. Because the superior and inferior articulating processes of C2 are coronally offset, extension applies significant strain on the C2 pars interarticularis . Under forceful hyperextension the pars interarticularis may fracture, giving rise to the mechanism and morphology of the so-called hangman’s fracture. As at other levels, this process is fenestrated by the transverse foramen, which lies immediately lateral to the C2 pedicle and serves as the conduit for the vertebral artery. Unlike at other levels, the C2 foramen is angulated 45 degrees laterally so that the vertebral artery is partially roofed by the superior articular process. The inferior articular process forms an articulation with the C3 superior articular process and assumes the more typical orientation of subaxial lateral masses.
Ligamentous Structures
Whereas the articular surfaces of the occipitocervical junction are oriented to permit constrained flexibility, a complex of intervening ligamentous structures act in concert to restrict excessive translation and rotation (see Figs. 8-2 through 8-4 ). Three ligaments span the divide between the odontoid process and the occiput. In the midline, the apical ligament spans the interval between the basion and the tip of the odontoid process. This ligament, also known as the middle odontoid ligament or suspensory ligament, is of unclear biomechanical significance as it has been described to be absent in 20% of specimens. The alar ligaments are bilaterally symmetric structures approximately 1 cm in length spanning from the dorsolateral odontoid tip to the medial occipital condyles. Each alar ligament restricts excessive rotation and lateral bending to the contralateral side.
The cruciate, or cruciform ligament, is the most important ligamentous structure of the craniovertebral junction. It is composed of four limbs, which unite over the dorsal odontoid process. The superior limb, or ascending band, inserts on the occiput, whereas the inferior limb, or descending band, inserts on the dorsal body of C2. The transverse atlantoaxial ligament forms the transverse limbs of this complex and attaches to bony tubercles on the medial borders of the C1 lateral masses. The transverse ligament is a strong, inelastic structure composed of primarily dense collagen with a ventrolateral transition to fibrocartilaginous tissue near its insertion on the C1 lateral masses. As a consequence, this lateral transition portion is the zone most susceptible to traumatic rupture, and the transverse atlantoaxial ligament has been demonstrated to rupture under loads of 400-1100 N. Rupture of this ligament may be identified on magnetic resonance imaging (MRI), particularly on gradient-echo sequences, and is associated with atlantoaxial instability. On occasion, the insertion of this ligament will fracture off of the medial wall of one or both lateral masses, whereas the ligament itself remains intact. The computed tomography (CT) and MRI characterizations of these disruption patterns have important implications on the management of traumatic atlantoaxial instability.
The anterior atlanto-occipital membrane is the rostral continuation of the anterior longitudinal ligament (ALL), extending from the atlantoaxial complex to the basion. The posterior atlanto-occipital membrane is the occipitocervical homologue to the ligamentum flavum. It extends from the posterior arch of C1 to the posterior rim of the foramen magnum, or opisthion.
Subaxial Cervical Spine
The third through seventh cervical vertebral segments share a similar morphology by virtue of their homologous embryologic origin. Because of this uniformity, the osseous and discoligamentous features of a single canonical vertebral segment and level are described.
Osseous Structures
Each vertebral segment can be divided into a ventral portion, the vertebral body, and a dorsal portion, the dorsal or vertebral arch. The cervical vertebral body is roughly cylindrical in geometry, although the anteroposterior (AP) diameter is typically smaller than the transverse diameter. Relative to the vertebral arch, the cervical vertebral bodies are smaller than the vertebral bodies of the thoracolumbar spine, likely because they bear significantly less load. The superior and inferior surfaces of the vertebral body serve as the superior and inferior end plates, respectively. The lateral edges of the superior end plate curve sharply upward to form the uncinate processes bilaterally, a unique feature of the cervical spine. These processes articulate with complementary bevels on the lateral surfaces of the adjacent inferior end plate. Although this articulation is referred to as the uncovertebral joint, it contains no synovial fluid and as a consequence is not a true joint ( Figs. 8-6 and 8-7 ).


The most distinctive feature of the cervical spine is the fenestration of the transverse processes, which flank each vertebral body. The transverse process projects ventrolaterally with a deep groove along its superior surface; this serves to carry the cervical spinal nerves. The transverse process terminates laterally with two prominences: the anterior and posterior tubercles. The anterior tubercle serves as an attachment for ventral cervical musculature, whereas the posterior tubercle serves as an attachment for the dorsal cervical musculature. The transverse foramen, or foramen transversarium , fenestrates the transverse process and carries the vertebral arteries bilaterally ( Figs. 8-8 and 8-9 ).


Importantly, the cervical pedicle serves as the dorsomedial wall of the foramen transversarium, exposing the vertebral artery to hazard when pedicle screws breach laterally ( Fig. 8-8 ). The cervical pedicles connect the dorsal arch with the vertebral body and are angled medially between 38 degrees and 48 degrees from the midsagittal plane. The pedicles of C3-7 range in outer diameter from 6 to 6.5 mm and in inner diameter from 2.7 to 3.1 mm. The pedicle is thinnest along its lateral wall. In aggregate, these features make safe placement of cervical pedicle screws a challenge. The medial wall is in close proximity to the thecal sac, the lateral wall is in close proximity to the vertebral artery, and the superior wall is in close proximity to the suprajacent nerve root.
The superior and inferior articular processes of the cervical spine are oriented obliquely on sagittal projection with complementary surfaces on adjacent segments. Together with the intervening bone, these articular processes combine to form lateral masses at each level, which are parallelogram-shaped in sagittal cross section. Adjacent lateral masses in the subaxial cervical spine are in close apposition so that in aggregate they form a flexible, cylindrical column of bone dorsolateral to the vertebral bodies. This pillar-like architecture affords axial load-bearing capacity to the dorsal vertebral arches. The oblique configuration of articular surfaces creates a shingle effect to the lateral masses and allows for flexion and extension while restricting translation, affording osseous neuroprotection for the enveloped cervical spinal cord and nerve roots (see Fig. 8-6 ). The lateral mass serves as a common anchor point for screw placement in the dorsal cervical spine, but an awareness of adjacent neurovascular structures is essential for safe placement avoidance of the vertebral arteries and cervical nerve roots. Rostral screw trajectory is protective of the exiting nerve root, whereas lateral trajectory protects the vertebral artery ( Figs. 8-11 through 8-13 .)

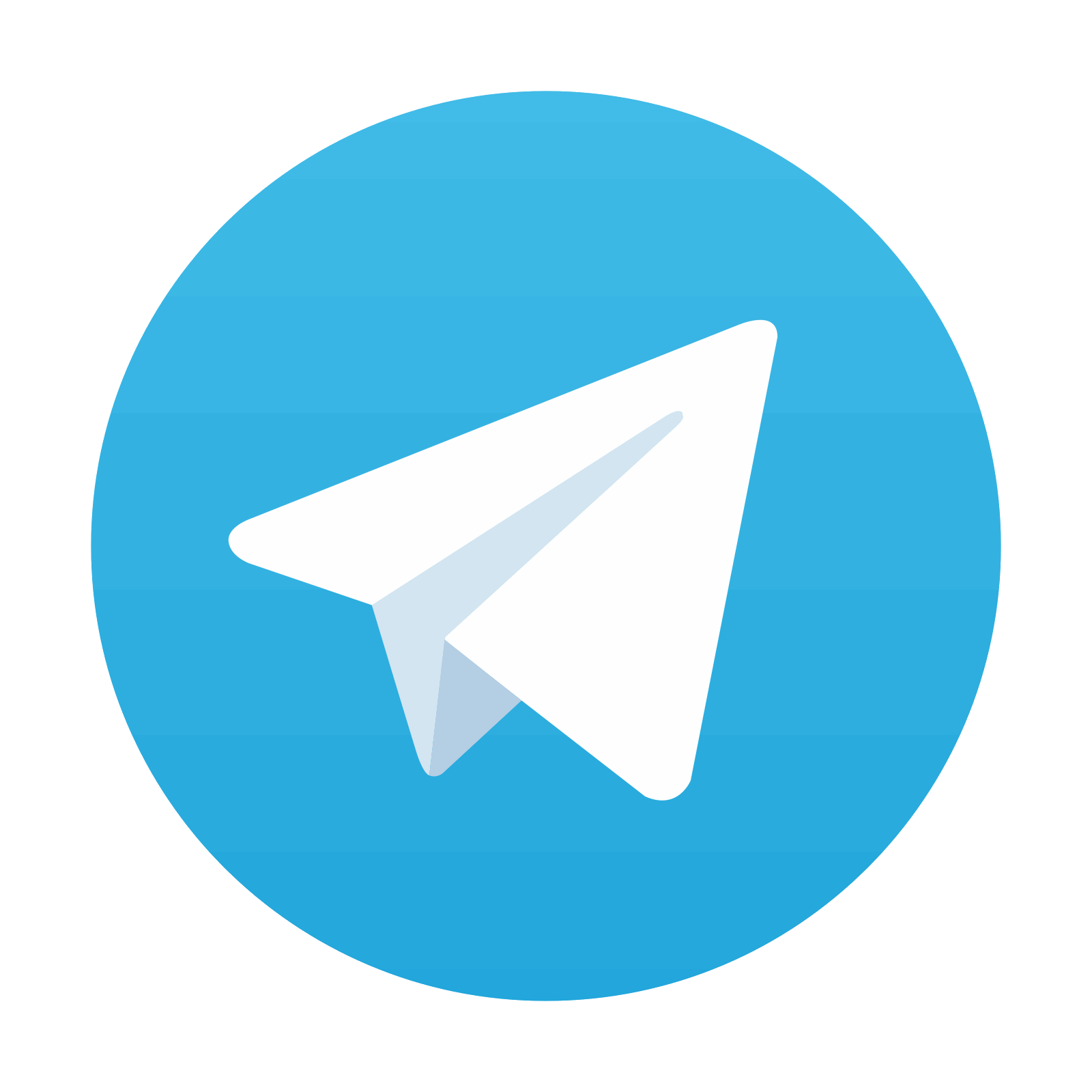
Stay updated, free articles. Join our Telegram channel

Full access? Get Clinical Tree
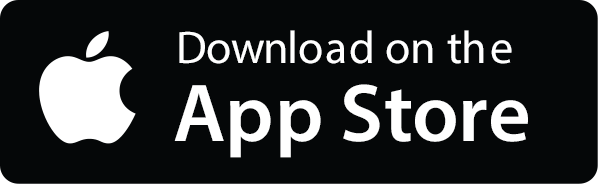
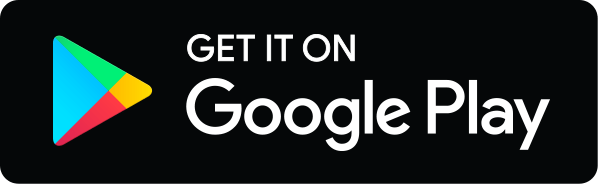
