Introduction
Cerebral radiation necrosis is a challenging and potentially devastating complication of cranial irradiation. Based on the temporal relationship to radiation therapy (RT), it can be classified as pseudoprogression (a form of early subacute injury) or radiation necrosis (a form of delayed injury). Pseudoprogression typically occurs within 3 months of fractionated RT and usually reverses spontaneously after a few weeks. Delayed radiation necrosis typically develops months or years after RT and is considered more irreversible. Radiation necrosis can have a waxing and waning clinical course but may also be relentlessly progressive. , The pathophysiology is attributed to a combination of vascular endothelial cell damage, glial cell injury, and immune–mediated reactions. These cascading events lead to increased permeability of the blood-brain barrier, followed by coagulative necrosis and demyelination. Vascular endothelial growth factor (VEGF) and hypoxia-inducible factor 1α appear to be increased in the tumor micro-environment and may play important roles in the development of radiation necrosis. ,
Diagnosis
Both early pseudoprogression and delayed radiation necrosis are typically characterized by increased contrast-enhancement or the appearance of ring-enhancing lesions on T1-weighed magnetic resonance imaging (MRI) sequences as well as increased vasogenic edema on T2-weighed MRI sequences. These changes may be accompanied by neurological symptoms such as headache, seizure, speech difficulty, cognitive dysfunction, or vision deficits. The clinical and imaging characteristics are indistinguishable from tumor progression or recurrence. However, they typically regress over time, and surgical resection of enhancing lesions reveals extensive necrotic tissues that may also contain minimal viable tumor cells that exhibit significant treatment-related changes. ,
The diagnosis of radiation necrosis can be very challenging and can pose significant dilemma for treating physicians. Prolonged follow-up of the clinical course with imaging is often preferred; surgery with histopathologic confirmation is the gold standard for confirming the diagnosis. , However, surgery is not always possible or preferable, and observation may risk delaying salvage treatment for recurrent tumor. Advanced imaging techniques, such as MR perfusion, MR spectroscopy, and positron emission tomography (PET), have been reported by different institutional studies as effective at distinguishing radiation necrosis from tumor recurrence with mixed results. , ,
MR perfusion . In one of the largest studies on MR perfusion, Barajas et al. retrospectively analyzed 57 glioblastoma (GBM) patients who developed progressively enlarging lesions within the RT fields after chemoradiotherapy and were evaluated using MR perfusion. Their study cohort consisted of 40 patients who had histologic confirmation of recurrent tumor, 15 with histologically confirmed radiation necrosis, and 2 with clinically diagnosed radiation necrosis. Although relative cerebral blood volume (rCBV), the common parameter used to analyze MR perfusion, was statistically higher among the recurrent tumor cohort than the radiation necrosis cohort, there was significant overlap between the two groups. Overall, rCBV did not reliably distinguish recurrent GBM from radiation necrosis (sensitivity: 79%; specificity: 72%). This study showed that relative peak height (rPH), a less common parameter to analyze MR perfusion, was more accurate (sensitivity: 89%; specificity: 81%). However, these findings have not been consistently replicated in other retrospective studies.
MR spectroscopy . MR spectroscopy measures metabolic composition of tissue such as lipid and choline to distinguish tumor and necrosis. However, it suffers from poor spatial resolution and low reproducibility, and its accuracy has been mixed in the literature. , ,
Positron emission tomography (PET) . Fluorodeoxyglucose (FDG)-based PET has generally shown modest predictive accuracy for distinguishing radiation necrosis from recurrent tumor in small retrospective studies, with sensitivity ranging from 65–81% and specificity ranging from 40–94%. Normal brain unfortunately exhibits variable FDG update and can obscure the uptake of a tumor. Radiation necrosis can also trigger repair mechanisms to increase glucose uptake, leading to a false-positive interpretation. Novel amino acid tracers, such as 3,4-dihydroxy-6-(18F)-fluoro-phenylalanine (FDOPA) or (18F)-fluciclovine, may be superior to FDG for PET evaluation of suspected radiation necrosis but are not widely available in most clinics. To date, none of these advanced imaging techniques have been validated in large prospective studies to reliably diagnose radiation necrosis from recurrent tumor. Thus, findings should be interpreted with caution and evaluated as part of the larger context of the patient’s clinical course and other risk factors for radiation necrosis or recurrent tumor. Suspected radiation necrosis cases should ideally be managed by an experienced multidisciplinary team of neurosurgeons, radiation oncologists, and neuro-oncologists.
Clinical cases
Case . A 69-year-old female with a newly diagnosed, IDH -wildtype, MGMT promoter methylated GBM underwent a gross total resection and then received standard fractionated RT of 60 Gy in 30 fractions ( Fig. 24.1A ) with concurrent temozolomide. One month after completion of chemoradiotherapy, her MRI was stable ( Fig. 24.1B ). However, 3 months after RT, there was increased enhancement around the resection cavity ( Fig. 24.1C ), with the radiology report stating “increased thick rim of enhancement around the resection cavity with associated elevated cerebral blood volume and markedly increased surrounding T2 hyperintensity, concerning for tumor progression.” She was continued on adjuvant temozolomide, and her MRI subsequently improved without changing her treatment ( Fig. 24.1D ).

Teaching Points . Approximately 20–30% of glioma patients can develop early pseudoprogression in the weeks after standard fractionated RT. As was the case for the patient presented in this case, imaging reveals findings that mimic those of tumor progression; however, in the case of pseudoprogression, serial imaging subsequently shows improvement or resolution of contrast enhancement and perilesional vasogenic edema.
The Response Assessment in Neuro-Oncology (RANO) criteria recommend that when there is worsening enhancement on MRI within 3 months of RT, progression should only be defined if the new enhancement is outside of the high-dose region (80% isodose line) of RT or if surgery confirms recurrent tumor. Recognition of this phenomenon is important to accurately counsel patients and to prevent premature discontinuation of effective adjuvant therapy. Concurrent chemotherapy, immunotherapy, and tumor-specific factors such as methylation of MGMT gene promoter appear to increase the risk of pseudoprogression. Interestingly, pseudoprogression has recently been reported to be less common in oligodendrogliomas with 1p/19q codeletion than IDH -mutant or IDH -wildtype astrocytomas. ,
Clinical Pearls
- 1.
Pseudoprogression is an earlier and reversible form of radiation necrosis that typically occurs within 3 months of chemoradiotherapy for glioma and resolves spontaneously.
- 2.
Although there is no imaging modality proven to provide accurate diagnosis of radiation necrosis, MR perfusion, MR spectroscopy, or PET may be considered to help supplement the diagnostic workup.
Case . A 56-year-old male with a grade 3 anaplastic oligodendroglioma with 1p/19q co-deletion, IDH mutation, and MGMT methylation underwent a subtotal resection. He subsequently received adjuvant chemotherapy of procarbazine/lomustine/vincristine, followed by RT using proton beam therapy to 59.4 Gy (Gray) relative biological equivalents (RBE, Fig. 24.2A ). Restaging MRI after RT demonstrated stable postsurgical changes ( Fig. 24.2B ). Approximately 1 year after RT, MRI showed an enhancing lesion abutting the left lateral ventricle and increased enhancement around the resection cavity ( Fig. 24.2C ). MRI perfusion and PET were concerning for progression. Multiple core biopsies showed necrosis only, and laser interstitial thermal therapy (LITT) of the enhancing region was performed. Enhancement subsequently recurred with worsening symptoms of confusion and headache. LITT was performed again, and the patient was treated with 6 months of bevacizumab with significant improvement of the enhancement and his symptoms ( Fig. 24.2D ).

Teaching Points . Delayed radiation necrosis occurs in approximately 5–10% of glioma patients treated with standard fractionated RT. The median latency time of developing symptomatic radiation necrosis is approximately 1 year after RT, with the vast majority occurring within 2 years of RT. As with the patient presented in this case, radiation necrosis may follow a progressive course with recurrence of imaging findings even after initial surgical treatment.
The risk of radiation necrosis appears to be related to radiation dose, radiation fraction size, and chemotherapy use. There are emerging data that 1p/19q co-deleted oligodendrogliomas may have increased risk of developing radiation necrosis than non–co-deleted astrocytomas, exhibiting relative steep dose-volume response when increasing volumes of brain are irradiated to 60 Gy. For example, a 5% increase of the percent of brain volume receiving 60 Gy is associated with approximately a 10% increase of radiation necrosis incidence for oligodendrogliomas.
Clinical Pearls
- 1.
Delayed radiation necrosis can occur months or years after fractionated RT and can continue to wax and wane even after surgical resection.
- 2.
Surgical resection or prolonged follow up of clinical course remains the best way to establish the diagnosis of radiation necrosis at the current time.
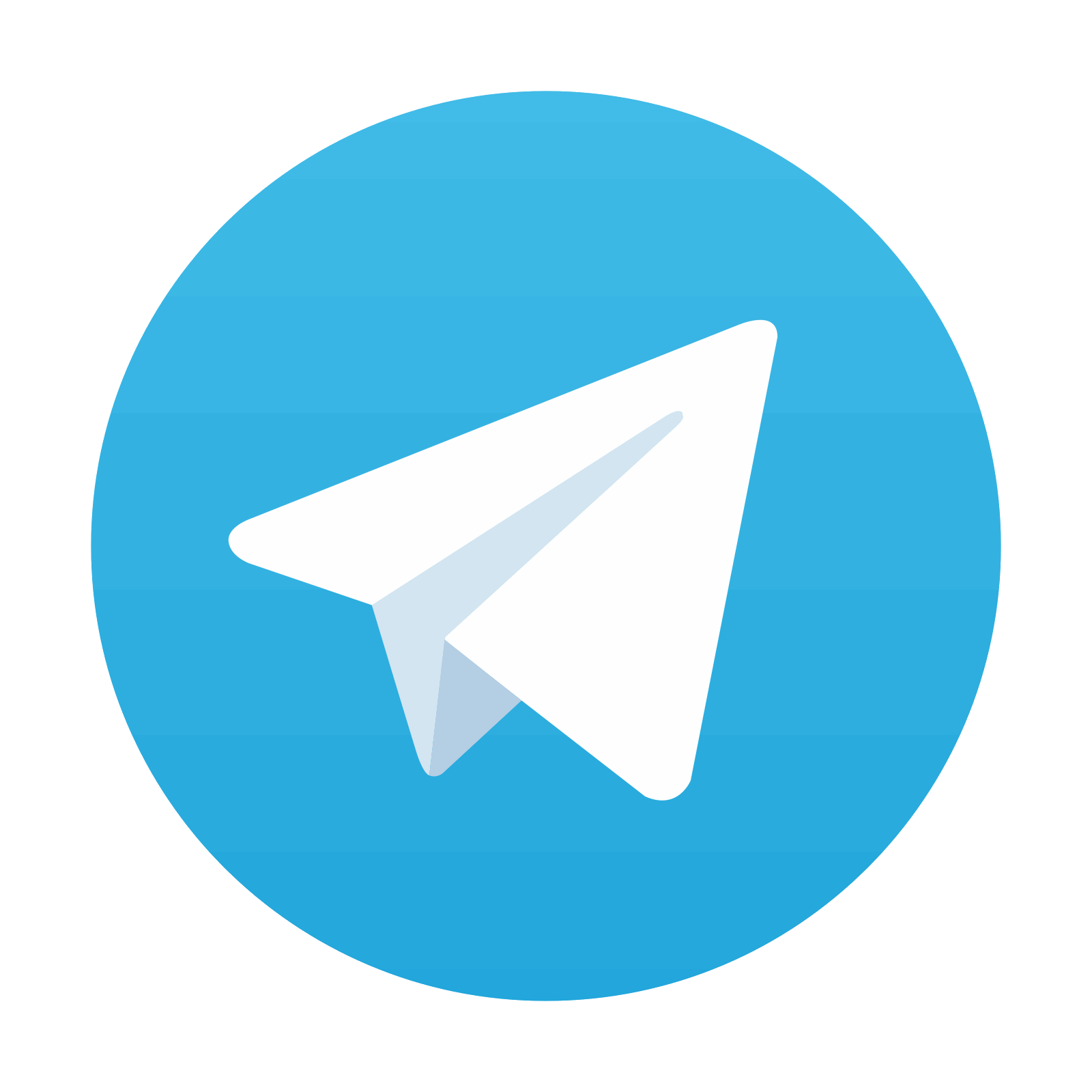
Stay updated, free articles. Join our Telegram channel

Full access? Get Clinical Tree
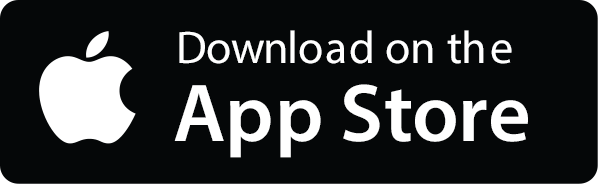
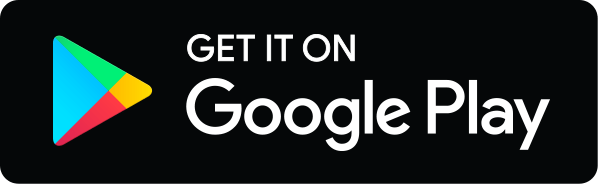
