Approaching the EEG: An Introduction to Visual Analysis
L. John Greenfield Jr
THE APPROACH TO READING
Your first exposure to reading EEGs was probably a daunting experience. How should you approach the complex patterns of waves? It may have been even more discouraging to see your attending, an experienced EEG reader, rapidly page through a study, spending a second or less on pages that took you minutes to analyze. The ability to read EEGs correctly and with confidence comes with experience, as you learn an orderly approach to reading and develop skills of pattern recognition that make your examination more efficient. The goal of this book is to provide the knowledge base necessary to understand what you are looking at and to help you develop the analytical and pattern recognition skills that will make you a competent EEG reader. You will need to learn how not to miss pathologic waveforms (“underreading”) without agonizing over every deflection of the pen, but also not to misclassify normal waveforms, variants, or artifacts as pathologic (“overreading”), which can cause problems as well.
So, how do we begin? The goal of reading EEGs is to identify abnormal electric activity and determine its significance. But the only way to know what is abnormal is to begin by identifying what is normal. Before learning what is normal, we first have to learn how to look at an EEG. This may sound trivial, but it is a critical skill that most readers develop over months to years of experience. While there is no substitute for experience, the techniques experienced readers use to analyze EEGs efficiently can be broken down into a few simple procedures. First, you need to know how to analyze waveforms visually and break them down into their component parts. The next step is to learn what patterns to look for in patients of different ages and different states of wakefulness and sleep. Finally, you need to “tune” your eyes to recognize abnormalities in those patterns, to determine whether those abnormalities were generated in the brain rather than extraneous sources (“artifacts”—see Chapter 5), and to interpret the significance of the abnormal findings.
VISUAL ANALYSIS OF EEG WAVEFORMS
When you begin to read EEG recordings, you will need to look for characteristics of the activity that will allow you to describe it in precise terms. Even if you do not completely understand the significance of the activity you see, being able to describe it in a consistent fashion may help you (and others) to interpret your findings later, perhaps when more clinical information is available. EEG activity is described in the following terms: frequency, amplitude, distribution or location, symmetry, synchrony, reactivity, morphology, rhythmicity, and regulation. We will define each of these in detail below.
Frequency
Frequency is defined as the number of complete waveforms that occur per second, expressed as cycles per second (cps) or Hz. If brain waves were composed of uniform single frequencies and amplitudes, the task of interpreting cerebral activity would be much simpler. More commonly, though, each channel of EEG activity is the sum of multiple frequencies of different amplitudes. One of the first skills you need to learn is how to identify and categorize the frequencies in a given waveform.
Frequencies recorded in standard EEG recordings are divided into four standard frequency ranges or “bands,” as noted in Table 4.1 and shown in Figure 4.1.
In Figure 4.1A, we can determine the frequency by counting the number of waves between the 1-second vertical bars. But the frequencies represented in an EEG waveform are not always simple. Figure 4.1B shows several examples of more complex waveforms that can be seen when two or more frequencies occur at the same time at the same electrodes. If the frequencies are widely separated, it may be possible to discern the component frequencies underlying
the combined waveform, but it becomes more challenging when multiple frequencies are represented, particularly if they are close together. The bottom trace in Figure 4.1B is an average of 2.5, 5, 10, and 15 Hz, in which individual frequencies are difficult to distinguish. Moreover, frequency components do not remain constant but may shift from moment to moment. Complex mixtures of frequencies may not be as easily susceptible to visual frequency analysis, but you should train your eye to recognize the components that may be lurking in EEG waveforms.
the combined waveform, but it becomes more challenging when multiple frequencies are represented, particularly if they are close together. The bottom trace in Figure 4.1B is an average of 2.5, 5, 10, and 15 Hz, in which individual frequencies are difficult to distinguish. Moreover, frequency components do not remain constant but may shift from moment to moment. Complex mixtures of frequencies may not be as easily susceptible to visual frequency analysis, but you should train your eye to recognize the components that may be lurking in EEG waveforms.
TABLE 4.1 EEG frequency ranges | |||||||||||||||
---|---|---|---|---|---|---|---|---|---|---|---|---|---|---|---|
|
REVIEW
4.1: What are the frequency ranges for the major frequency bands seen in routine EEG recordings?
View Answer
4.1: Alpha activity is from 8 to 13 Hz, beta is from 13 to 25 Hz, theta is from 4 to 8 Hz, and delta is <4 Hz.
Amplitude
Amplitude is the size of the waveforms, measured in microvolts (µV). There are minor complexities here as well. Amplitude is often measured “peak to peak,” that is, from the highest to the lowest point of the sinusoidal wave. However, this can be misleading if there is drift of the waveform due to slow oscillations (eg, alpha waves superimposed on delta waves, as in Fig. 4.1B). The different frequency components may have different amplitudes associated with them. The breakdown of amplitudes for different frequencies can be done by sorting out the waveforms into the sum of sine waves of different frequencies by Fourier analysis (often done by computer using fast Fourier transform or FFT), which produces a graph of power vs frequency (recall from Chapter 1 that power is voltage times current and is thus proportional to the amplitude of the signal for each frequency component). In reporting the amplitude of the activity, you may choose to describe the overall amplitude of all components or the amplitude of each frequency component. Amplitude can be reported as a numeric range (eg, 20-40 µV) or in descriptive terms as low (0-25 µV), moderate (25-75 µV), or high (>75 µV) amplitude. Some pathologic conditions are associated with enormous amplitudes of hundreds of µV, such as hypsarrhythmia, a chaotic pattern seen in severe infantile epilepsies.
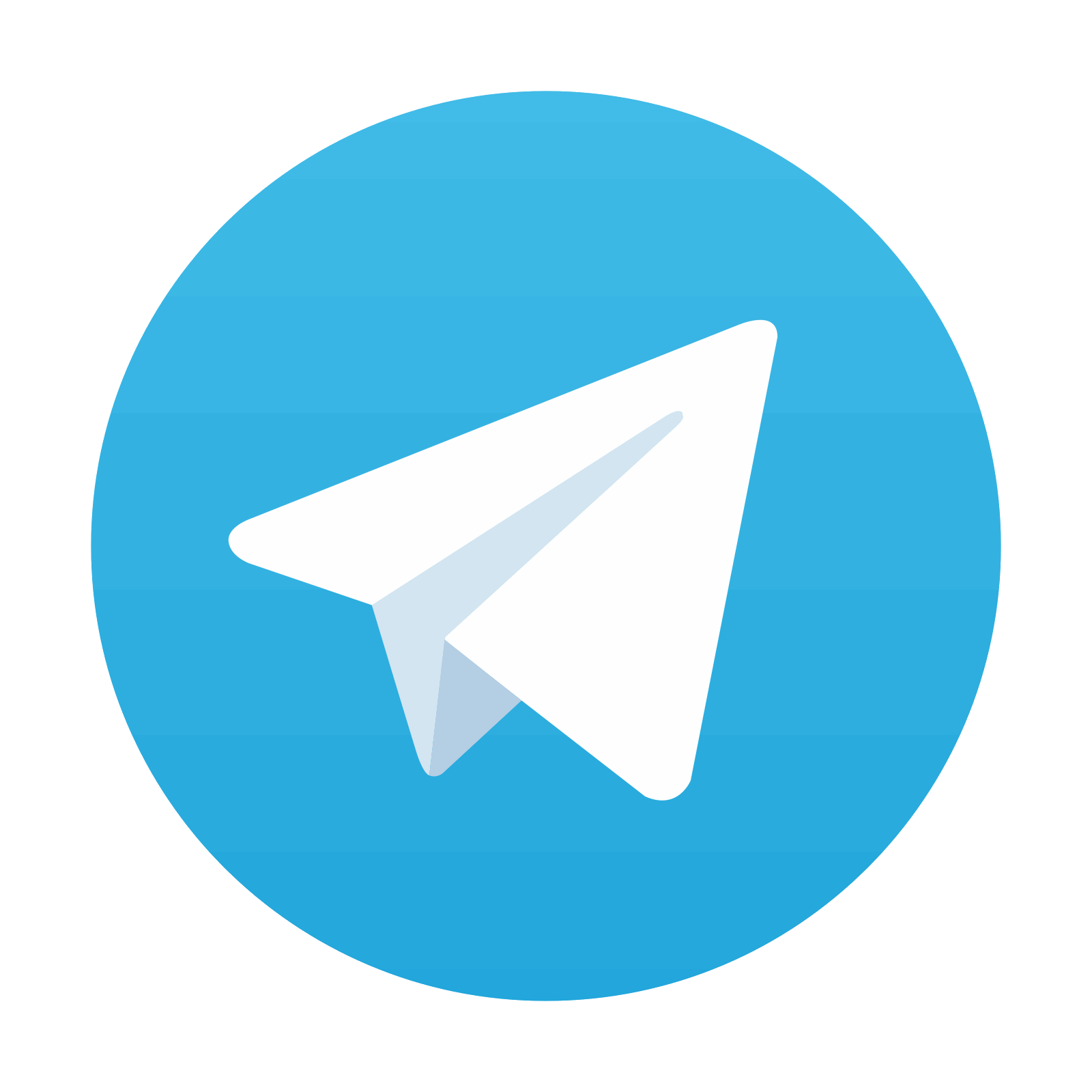
Stay updated, free articles. Join our Telegram channel

Full access? Get Clinical Tree
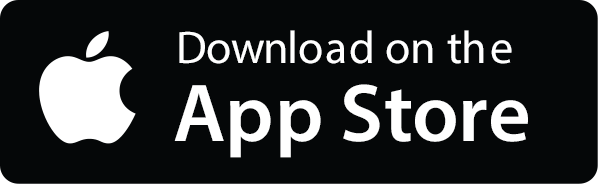
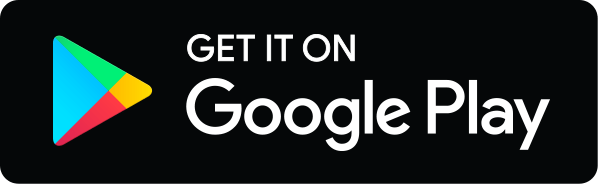