Arterial Anatomy and Strokes
Main Text
Preamble
“Stroke” is a generic term that describes a clinical event characterized by sudden onset of a neurologic deficit. However, not all strokes are the same! Stroke syndromes have significant clinical and pathophysiologic heterogeneity that is reflected in their underlying gross pathologic and imaging appearances. Arterial ischemia/infarction—the major focus of this chapter—is by far the most common cause of stroke, accounting for 80% of all cases.
The remaining 20% of strokes are mostly hemorrhagic, divided between primary “spontaneous” intracranial hemorrhage (sICH), nontraumatic subarachnoid hemorrhage (SAH), and venous occlusions. Both sICH and SAH were discussed extensively in preceding chapters, and venous occlusions will be discussed in the following chapter.
We begin by briefly reviewing the normal intracranial arteries and their vascular distributions. With this solid anatomic foundation, we then turn our attention to the etiology, pathology, and imaging manifestations of arterial strokes.
Normal Arterial Anatomy and Vascular Distributions
Intracranial Internal Carotid Artery
Normal Anatomy
The intracranial internal carotid artery (ICA)follows a complex course with several vertical or horizontal segments connected by three curved genus. There are six intracranial segments: The petrous (C2), lacerum (C3), cavernous (C4), clinoid (C5), ophthalmic (C6), and communicating (C7) segments (8-1) (8-2). C1 is the ICA within the neck known as the cervical ICA.
The petrous (C2) segment is contained within the carotid canal of the temporal bone. As the ICA enters the skull at the carotid canal, it lies just anterior to the internal jugular vein. At this point, the ICA goes from being mobile in the neck, to relatively fixed in the bone, where it is more vulnerable to traumatic shearing forces and dissection.
The C2 segment is surrounded by the extensive sympathetic plexus. The C2 subsegments are joined at the genu. There is a short vertical segment, a genu, where the petrous ICA turns anteromedially in front of the cochlea, and a longer horizontal segment. The ICA exits the carotid canal at the petrous apex.
The C2 segment has two small but important branches. The vidian artery, a.k.a. the artery of the pterygoid canal, anastomoses with external carotid artery (ECA) branches, most commonly the internal maxillary artery. The second branch is the small caroticotympanic artery, which supplies the middle ear.
The lacerum (C3) segment is a short segment that extends from the petrous apex just above foramen lacerum, curving upward toward the cavernous sinus. It is covered by the trigeminal ganglion and has no branches.
The cavernous (C4) segment has three subsegments joined by two genus. There is a posterior vertical (ascending) portion, a posterior (more medial) genu, a horizontal segment, an anterior (more lateral) genu, and an anterior vertical (subclinoid) segment. The C4 segment is covered by trigeminal ganglion posteriorly.
The abducens nerve (CNVI) is inferolateral to the C4 segment. The major branches include the meningohypophyseal trunk, which arises from the posterior genu and supplies the pituitary, tentorium, and clival dura. The inferolateral trunk arises from the horizontal segment and supplies the cavernous sinus dura and cranial nerves. The inferolateral trunk anastomoses with the ECA branches through foramen rotundum, spinosum, and ovale.
The clinoid (C5) segment is a short segment between the proximal and distal dural rings of the cavernous sinus. The C5 segment terminates as the ICA exits the cavernous sinus and enters the subarachnoid space near the anterior clinoid process. There are no important branches unless the ophthalmic artery arises within the cavernous sinus.
The ophthalmic (C6) segment is the first ICA segment that lies completely within the subarachnoid space. It extends from the distal dural ring at the superior clinoid to just below the posterior communicating artery (PCoA) origin.
There are two important branches that arise from the ophthalmic ICA segment. The ophthalmic branch originates from the anterosuperior C6 ICA, passes through the optic canal to the orbit. The ophthalmic artery gives off ocular, lacrimal, and muscular branches. There are extensive anastomoses with the ECA. The superior hypophyseal artery courses posteromedially and supplies the anterior pituitary, infundibulum, and optic nerve and chiasm.
The communicating (C7) segment is the last ICA segment and extends from just below the PCoA origin to the terminal ICA bifurcation into the anterior cerebral artery (ACA) and middle cerebral artery (MCA). The C7 segment passes between the optic (CNII) and oculomotor (CNIII) nerves.
The major branches of the C7 segment are the PCoA and the anterior choroidal artery. The PCoA joins the anterior to the posterior circulation. The anterior choroidal artery courses posteromedial and then turns superolateral in the suprasellar cistern. It enters the temporal horn at the choroidal fissure. It supplies the choroid plexus, medial temporal lobe, basal ganglia, and posteroinferior internal capsule. The anterior choroidal artery is reciprocal with the posterior choroidal arteries, which are branches of the posterior cerebral artery (PCA).
Variants and Anomalies
There are three important ICA vascular anomalies: An aberrant ICA, a persistent stapedial artery, and an embryonic carotid-basilar anastomosis.
An aberrant ICA is a congenital vascular anomaly that enters the posterior middle ear cavity from below and hugs the cochlear promontory as it crosses the middle ear (8-3). An aberrant ICA is important to recognize, as it may mimic a middle ear tumor, the glomus tympanicum paraganglioma. Approximately 30% of aberrant ICAs have an associated persistent stapedial artery.
Appearance of an aberrant ICA on thin-section (< 1-mm) temporal bone CT or CTA is diagnostic (8-4). The artery appears as a tubular structure crossing the middle ear from posterior to anterior. There may be an enlarged inferior tympanic canaliculus. The location of the aberrant ICA is posterior and lateral to the expected site of the petrous carotid canal. It is typically unilateral and may be discovered at the time of routine physical examination, during middle ear surgery, or as an incidental imaging finding. It has been associated with pulsatile tinnitus and conductive hearing loss.
A persistent stapedial artery is a rare congenital vascular anomaly where the embryonic stapedial artery persists. This anomaly is typically found incidentally and is associated with an aberrant ICA. On temporal bone CT or CTA, there is absence of the foramen spinosum. The persistent stapedial artery may be seen as a tiny linear vessel in the medial aspect of the middle ear. There is typically enlargement of the anterior tympanic segment of the facial nerve canal. It is often a bilateral lesion.
Embryonic carotid-basilar anastomoses are abnormal connections between the carotid and basilar system related to persistence of normally transient embryonic-type arterial supply. There are four types, named according to their anatomic relationship to specific cranial or spinal nerves. From superior to inferior, these are a persistent trigeminal artery (CNV), persistent otic artery (CNVIII), persistent hypoglossal artery (CNXII), and proatlantal intersegmental artery (C1-C3) (8-5).
The persistent trigeminal artery (PTA) arises from the intracavernous ICA and connects with the basilar artery (BA) and parallels the course of the trigeminal nerve. On lateral DSA and sagittal CTA and MRA, it often forms a trident shape (8-6). It is the most common of the persistent anastomoses and is identified in 0.1-0.2% of cases. Nearly 25% of patients with PTA have associated vascular anomalies, such as aneurysm, moyamoya, aortic coarctation, and arterial fenestrations.
The persistent otic artery (POA) arises from the petrous ICA to connect with the BA through the internal auditory canal. It is very rare with only a few cases reported. The persistent hypoglossal artery arises from the cervical ICA at the C1-C2 level and connects to the BA. It is a rare lesion with a prevalence of ~ 0.03-0.09%. The proatlantal intersegmental artery arises from the cervical ICA at the C2-C3 level, or, rarely, from the ECA to connect with the vertebral artery (VA) between C1 and the occiput.
Circle of Willis
Normal Anatomy
The circle of Willis (COW) has 10 components: Two ICAs, two proximal or horizontal (A1) ACA segments, the anterior communicating artery (ACoA), two PCoAs, the BA, and two proximal or horizontal (P1) segments of the PCAs (8-7) (8-8). The MCA is not part of the COW.
Vascular Territory
Important perforating branches arise from all parts of the COW and supply most of the basilar brain structures. COW variants are the rule, not the exception. One or more components of the COW is hypoplastic or absent in the majority of cases. A hypoplastic or absent PCoA is the most common COW variant.
Anterior Cerebral Artery
Normal Anatomy
The ACA is the smaller, more medial terminal branch of the supraclinoid ICA. Its first (horizontal) ACA segment is also termed A1. Small perforating vessels arise from A1 and supply the medial basal ganglia.
The A2or vertical ACA segment extends from the A1-ACoA junction to the corpus callosum rostrum (8-9). The A3 (callosal) segment curves anteriorly around the corpus callosum genu then divides into two terminal ACA branches, the pericallosal and callosomarginal arteries.
Vascular Territory
Cortical ACA branches supply the anterior 2/3 of the medial hemispheres and corpus callosum, the inferomedial surface of the frontal lobe, and the anterior 2/3 of the cerebral convexity adjacent to the interhemispheric fissure (8-10). Penetrating ACA branches (mainly the medial lenticulostriate arteries) supply the medial basal ganglia, corpus callosum genu, and anterior limb of the internal capsule.
Middle Cerebral Artery
Normal Anatomy
The MCA has four defined segments. The M1MCA (horizontal) segment extends laterally from the ICA bifurcation toward the sylvian (lateral cerebral) fissure. Lateral lenticulostriate and anterior temporal arteries arise from the M1(8-11). The lateral lenticulostriates supply the lateral putamen, caudate nucleus, and external capsule. The M1 segment usually bifurcates or trifurcates just before it enters the sylvian fissure. The postbifurcation trunks enter the sylvian fissure then turn upwards. In current stroke trials, the M1/M2 junction is considered the MCA as it turns upwards in the sylvian fissure. Classic teaching of the location of the M1/M2 junction has been at the bifurcation/trifurcation. It is important to be descriptive when discussing the location of an intraluminal thrombus, as it may directly impact patient care.
The M2 (insular) branches turn posterosuperiorly in the sylvian fissure, following a gentle curve (the genu or “knee” of the MCA). Several branches sweep upward over the surface of the insula. The insular M2 segments end at the top of the sylvian fissure.
MCA branches loop at or near the top of the sylvian fissure, then course laterally under the parts (“opercula”) of the frontal, parietal, and temporal lobes that hang over and enclose the sylvian fissure. These are the M3or opercular segments. MCA branches become the M4segments when they ramify over the lateral surface of the cerebral hemisphere.
Vascular Territory
The MCA has the largest vascular territory of any of the major cerebral arteries. The MCA supplies most of the lateral surface of the cerebral hemisphere with the exception of a thin strip at the vertex (supplied by the ACA) and the occipital and posteroinferior parietal lobes (supplied by the PCA) (8-12). Its penetrating branches supply most of the lateral basal brain structures.
Posterior Cerebral Artery
Normal Anatomy
The two PCAs are the major terminal branches of the distal BA.
The P1 segment extends laterally from the BA bifurcation to the junction with the PCoA. The P1 segment has perforating branches that course posterosuperiorly in the interpeduncular fossa to enter the undersurface of the midbrain.
The P2segment extends from the P1-PCoA junction, running in the ambient (perimesencephalic) cistern as it sweeps posterolaterally around the midbrain (8-13). P3 (quadrigeminal) is a short segment that lies entirely within the quadrigeminal cistern. It begins behind the midbrain and ends where the PCA enters the calcarine fissure of the occipital lobe. The P4segment terminates within the calcarine fissure, where it divides into the terminal PCA trunks, including the calcarine artery.
Vascular Territory
The PCA supplies most of the inferior surface of the cerebral hemisphere with the exception of the temporal tip and frontal lobe. It also supplies the occipital lobe, posterior 1/3 of the medial hemisphere and corpus callosum, and most of the choroid plexus (8-14). Penetrating PCA branches are the major vascular supply to the midbrain and posterior thalami.
Variants and Anomalies
A common normal variant is the “fetal” origin of the PCA.Here, the proximal PCA arises from the ICA instead of from the basilar bifurcation. “Fetal” PCA origin is seen in 10-30% of cases. This variant is easily recognized on CTA, MRA, and DSA.
A rare but important PCA variant is an artery of Percheron (AOP). Here, a single dominant thalamoperforating artery arises from the P1 segment and supplies the rostral midbrain and bilateral medial thalami.
Vertebrobasilar System
Normal Anatomy
The vertebrobasilar system consists of the two VAs, the BA, and their branches. Four VA segments are identified. Only one—the V4 segment—is intracranial (8-15).
The V1 (extraosseous segment) arises from the ipsilateral subclavian artery and courses posterosuperiorly to enter the C6 transverse foramen. The V2 segment courses superiorly through the C6-C3 transverse foramina until it reaches C2, where it first turns superolaterally through the “inverted L” of the transverse foramen and then turns upward to pass through the C1 transverse foramen.
V3begins after the VA exits the C1 transverse foramen. The V4 (intracranial) segment extends from the foramen magnum to its junction with the BA. The VAs unite at or near the pontomedullary junction to form the BA. After giving off pontine and cerebellar branches, the BA terminates by dividing into the two PCAs.
Vascular Territory
The vertebrobasilar system normally supplies all of the posterior fossa structures as well as the midbrain, posterior thalami, occipital lobes, most of the inferior and posterolateral surfaces of the temporal lobe, and upper cervical spinal cord (8-16).
Arterial Infarcts
Acute Cerebral Ischemia-Infarction
Imaging is the basis of rapid stroke triage. Because of speed and accessibility, NECT and CTA (with or without CT perfusion) are the most commonly used modalities.
There are four “must know” questions in acute stroke triage that need to be answered rapidly and accurately. (1) Is intracranial hemorrhage or a stroke “mimic” present? (2) Is a large vessel occluded? (3) Is part of the brain irreversibly injured (i.e., is there a core of critically ischemic, irreversibly infarcted tissue)? (4) Is there a clinically relevant“penumbra” of ischemic but potentially salvageable tissue?
4 “MUST KNOW” ACUTE STROKE QUESTIONS
• (1) Is there intracranial hemorrhage (or stroke “mimic”)?
• (2) Is large vessel occluded?
• (3) Is part of brain irreversibly injured?
• (4) Is ischemic “penumbra” present?
Terminology
Stroke—a generic term meaning sudden onset of a neurologic event—is also referred to as a cerebrovascular accident (CVA) or “brain attack.”
The distinction between cerebral ischemia and cerebral infarction is subtle but important. In cerebral ischemia, the affected tissue remains viable, although blood flow is inadequate to sustain normal cellular function. In cerebral infarction, frank cell death occurs with loss of neurons, glia, or both.
Timing is important in patient triage . Hyperacute stroke designates events within the first six hours following symptom onset. In hyperacute stroke, cell death has not yet occurred, so the combined term acute cerebral ischemia-infarction is often used. Acute strokes are those 6-48 hours from onset.
Etiology
Stroke Subtypes
Atherosclerotic (ASVD) strokes are the most common type of acute arterial ischemia/infarction, representing ~ 40-45% of cases.
Most large artery territorial infarcts are embolic, arising from thrombi that develop at the site of an “at-risk” ASVD plaque. The most common site is the carotid bifurcation. The most frequently occluded intracranial vessel is the MCA.
Small vessel disease represents 15-30% of all strokes. Small artery occlusions, a.k.a. lacunar infarcts, are defined as lesions measuring < 15 mm in diameter. Many are clinically silent, although a strategically located lesion (e.g., in the internal capsule) can cause significant neurologic impairment.
Lacunar infarcts can be embolic, atheromatous, or thrombotic. Most involve penetrating arteries in the basal ganglia/thalami, internal capsule, pons, and deep cerebral white matter (WM).
Cardioembolic disease accounts for another 15-25% of major strokes.
Pathophysiology
An estimated two million neurons are lost each minute when a major vessel, such as the MCA, is suddenly occluded. CBF falls precipitously. The center of the affected brain parenchyma—the densely ischemic core—typically has a CBF < 6-8 cm³/100 g/min.
Neuronal death with irreversible loss of function occurs in the core of an acute stroke. A relatively less ischemic penumbra surrounding the central core is present in ~ 1/2 of all patients. CBF in the penumbra is significantly reduced, falling from a normal of 60 cm³/100 g/min to 10-20 cm³/100 g/min. This ischemic but not-yet-doomed-to-infarct tissue represents physiologically “at-risk,” but potentially salvageable, tissue.
Pathology
Location
The MCA is the most common site of large artery thromboembolic occlusion (8-17) followed by the PCA and vertebrobasilar circulation. The ACA is the least commonly occluded major intracranial vessel.
Size and Number
Acute infarcts can be solitary or multiple and vary in size from tiny lacunar to large territorial lesions that can involve much of the cerebral hemisphere.
Gross Pathology
An acutely thrombosed artery is filled with soft purplish clot that may involve the entire vessel or just a short segment.
Gross parenchymal changes are minimal or absent in the first 6-8 hours, after which edema in the affected vascular territory causes the brain to appear pale and swollen. The gray matter (GM)-WM boundaries become less distinct and more “blurred.” As the gyri expand, the adjacent sulci are compressed, and the sulcal-cisternal CSF space is effaced.
Clinical Issues
Epidemiology and Demographics
Stroke is the third leading cause of death in industrialized countries and is the major worldwide cause of adult neurologic disability.
Strokes affect patients of all ages—including newborns and neonates—although most occur in middle-aged or older adults. Children with strokes often have an underlying disorder, such as right-to-left cardiac shunt, sickle cell disease, or inherited hypercoagulable syndrome. Strokes in young adults are often caused by dissection (spontaneous or traumatic) or drug abuse.
Presentation
Sudden onset of a focal neurologic deficit, such as facial droop, slurred speech, paresis, or decreased consciousness, is the most common presentation. The NIH Stroke Scale (NIHSS) is a 15-item neurologic examination scale to assess stroke severity and changes in clinical status. The score ranges 0-42 with higher scores indicating greater stroke severity.
Natural History
Stroke outcome varies widely. Between 20-25% of strokes are considered “major” occlusions and cause 80% of adverse outcomes. Six months after stroke, 20-30% of all patients are dead, and a similar number are severely disabled.
Prognosis in individual patients depends on a number of contributing factors, i.e., which vessel is occluded, the presence or absence of robust collateral blood flow, and whether there is a significant ischemic penumbra.
Uncontrolled brain swelling with herniation and death can result from so-called malignant MCA infarction. In such cases, emergent craniectomy may be the only treatment option.
Treatment Options
Ultrafast stroke triage is essential with the goal of a “door to needle” time (i.e., from arrival in the emergency department to intervention) < 60 minutes.
The single most important factor in successful intervention is patient selection with the two most important considerations being (1) elapsed time from symptom onset and (2) imaging findings.
Once hemorrhage has been excluded by NECT, intravenous recombinant tissue plasminogen activator (rTPA) may be administered, as patients are often transferred to a major stroke center (“drip and ship”), especially if elapsed time is less than three hours from ictus (the so-called golden hours). Most centers have expanded the treatment window for IV tPA to four and 1/2 hours from onset of symptoms.
Several large studies have shown that the most effective treatment for acute large vessel occlusion (LVO) by thrombus is endovascular mechanical thrombectomy. Endovascular therapy (EVT) significantly improves outcomes and reduces long-term disability after ischemic stroke. Stent retriever and clot aspiration are the two most currently used EVT techniques with continued improvements leading to very high reperfusion rates.
Evaluating endovascular success is done with a modified thrombolysis in cerebral infarction (TICI) grading system. The TICI score ranges 0-3 with grade 0 = no reperfusion, grade 1 = limited distal filling past the initial occlusion, grade 2 = further reperfusion with subdivisions based on the amount of reperfused MCA territory (2a: < 50%; 2b: > 50%; 2c: 90-99%), and grade 3 indicates complete reperfusion (8-26). Grade ≥ 2b is considered “successful” reperfusion.
Imaging
“Brain Attack” Protocols
The primary goals of emergent stroke imaging are (1) to distinguish “bland” or ischemic stroke from intracranial hemorrhage and (2) to select/triage patients for possible reperfusion therapies.
Most protocols begin with emergent NECT to answer the first“must know” question in stroke imaging: Is intracranial hemorrhage or a stroke “mimic” (such as subdural hematoma or neoplasm) present? If a typical hypertensive hemorrhage is identified on the screening NECT and the patient has a history of systemic hypertension, no further imaging is generally required. CTA is sometimes requested to evaluate for active bleeding (spot sign).
Once intracranial hemorrhage is excluded, the second critical issue is determining whether a major cerebral vessel is occluded. CTA can be obtained immediately following the NECT scan and is the noninvasive procedure of choice for depicting potentially treatable major vessel occlusions. MRA is more susceptible to motion artifact, which is accentuated in uncooperative patients. DSA is typically reserved for patients undergoing EVT.
The third and fourth questions can be answered with either CT or MR perfusion (pCT, pMR) studies. Both can depict what part of the brain is irreversibly damaged (i.e., the unsalvageable core infarct) and determine whether there is a clinically relevant ischemic penumbra (potentially salvageable brain).
CT Findings
A complete multimodal acute stroke CT protocol includes nonenhanced head CT, an arch-to-vertex CTA, and dynamic first-pass pCT. With helical acquisition, the entire protocol can be completed within 15 minutes as a single examination with separate contrast boluses. CTA with pCT improves diagnostic accuracy compared with NECT alone and does not delay intravenous tPA or EVT.
NECT
Initial NECT scans—even those obtained in the first six hours—are abnormal in 50-60% of acute ischemic strokes if viewed with narrow window width.
The most specific, but least sensitive, sign is a hyperattenuating vessel filled with acute thrombus. A dense MCA sign is seen in 30% of cases with documented M1 occlusion (8-18). Less common sites for a hyperdense vessel sign are the intracranial ICA, BA, and MCA branches in the sylvian fissure (dot sign) (8-19).
Uncommon but important NECT findings that indicate vascular occlusion include one or more calcified emboli (8-34), most likely from an “at-risk” ulcerated atherosclerotic plaque in the cervical or cavernous ICA. It is critically important to identify calcified cerebral emboli, as they carry a near 50% risk of repeat ischemic stroke.
Blurring and indistinctness of GM-WM interfaces can be seen in 50-70% of cases within the first three hours following occlusion. Loss of the insular cortex (insular ribbon sign) (8-21A)and decreased density of the basal ganglia (disappearing basal ganglia sign)are the most common findings.
Wedge-shaped parenchymal hypodensity with indistinct GM-WM borders and cortical sulcal effacement develops in large territorial occlusions (8-21B). If > 1/3 of the MCA territory is initially involved, the likelihood of a “malignant” MCA infarct with severe brain swelling rises, as does the risk of hemorrhagic transformation with attempted revascularization.
The Alberta Stroke Program Early Computed Tomographic Score (ASPECTS) is a straightforward, quick, and reproducible measure of early ischemic change (8-20). ASPECTS is calculated by subtracting one point for each of 10 regions affected. ASPECTS ≤ 6 equates to > 1/3 of the MCA territory and has been associated with increased risk of hemorrhage and poor outcome (8-21).
In the past, most trials excluded patients with ASPECTS of < 5 or a core infarct volume of > 70 mL. These patients, however, have poor outcomes. Therefore, newer trials have expanded the treatment windows to include patients with lower ASPECTS. These stroke trials have continued to show improved patient outcomes with EVT in patients with lower ASPECTS, 3-5.
CTA
CTA (with or without CT perfusion) quickly answers the second“must know” stroke question (8-22A), i.e., is a major vessel occlusion with a “retrievable” intravascular thrombus present? CTA defines the intravascular thrombus and assesses collateral blood flow.
pCT
The third and fourth“must know” questions can be answered with whole-brain pCT. pCT depicts the effect of vessel occlusion on the brain parenchyma itself, offering a rapid assessment of cerebral hemodynamics and parenchymal viability that is key to acute stroke management (8-23).
Classic pCT has three major parameters: Cerebral blood volume (CBV), CBF, and mean transit time (MTT). CBV is the volume of flowing blood in a given volume of brain. CBF is the volume of flowing blood moving through a given volume of brain in a specified amount of time. MTT is the average time it takes blood to transit through a given volume of brain. Parameters similar to MTT often used in pCT include time to peak (TTP) and time to drain (TTD).
All three pCT parameters can also be depicted either visually or on a color scale. The standard color scale is graduated from shades of red and yellow to blue and violet. With CBV and CBF, perfusion is portrayed in red/yellow/green (highest) to blue/purple/black (lowest). Well-perfused GM appears red/yellow, WM appears blue, and ischemic brain is blue/purple.
The densely ischemic infarct core—the irreversibly injured brain—shows matched reduction in bothCBV and CBF and is seen as a dark blue/purple or black area (8-23A). Prolonged MTT is seen as a red area in contrast to the blue brain, in which transit time is normal (8-23C).
An ischemic penumbra with potentially salvageable tissue is seen as a “mismatch” between markedly reduced CBV in the infarcted core and a surrounding area (penumbra) characterized by decreased CBF with normal or even transiently increased CBV. Thus, the potentially salvageable brain tissue is equivalent to CBV – CBF. Between 15-20% of large MCA infarcts cause hypoperfusion with reduced CBF in the contralateral cerebellum, a phenomenon called crossed cerebellar diaschisis.
Parameters present on automated processing maps include CBF, time to maximum of the tissue residue function (Tmax), CBV, and MTT. Only CBF and Tmax have been widely studied in randomized clinical trials. Automated pCT software estimates core infarct volume on the basis of a < 30% threshold for CBF reduction and penumbral volume on the basis of a threshold > 6 seconds for prolongation of Tmax. Automated processing can quickly measure volumes with CBF < 30% (ischemic core infarct) and Tmax > 6 seconds (penumbra) as well as calculate mismatch volumes and ratios (8-23D).
Management of acute ischemic stroke is continually evolving. The initial target mismatch profile for those patients who may benefit from EVT included an ischemic core volume < 70 mL, a mismatch ratio > 1.8, and a mismatch volume > 15 mL (8-25). New studies are changing these values to be more inclusive of patients eligible for EVT with favorable results.
MR Findings
Although CT/CTA/pCT is often preferred because of accessibility and speed, “expedited” rapid stroke protocols with only fast FLAIR, T2*, DWI, and pMR can be used. MR is superior to CT in detecting small ischemic and lacunar strokes.
T1WI
T1WI is usually normal within the first 3-6 hours. Subtle gyral swelling and hypointensity begin to develop within 12-24 hours and are seen as blurring of the GM-WM interfaces. With large vessel occlusions, loss of the expected “flow void” in the affected artery can sometimes be identified.
T2/FLAIR
Only 30-50% of acute strokes show cortical swelling and hyperintensity on FLAIR scans within the first four hours. Nearly all strokes are FLAIR positive by seven hours following symptom onset (8-22C). Intraarterial hyperintensity on FLAIR is an early sign of stroke and indicates slow flow, either from delayed antegrade flow or, more commonly, retrograde collateral filling across the cortical watershed (WS) (8-24A). FLAIR-DWI “mismatch” (negative FLAIR, positive DWI) has been suggested as a quick indicator of viable ischemic penumbra and eligibility for thrombolysis.
T2* GRE
Intraarterial thrombus can sometimes be detected as “blooming” hypointensity on T2* (GRE, SWI) (8-22B). Large MCA infarcts sometimes exhibit increased hypointensity in deep medullary veins due to prolonged transit time and increased deoxyhemoglobin. Although hemorrhagic transformation may sometimes occur as early as 24-48 hours following ictus, it is more typical of late acute and early subacute infarcts.
T1 C+
Postcontrast T1 scans show intravascular enhancement (8-24D). Parenchymal enhancement is uncommon in acute/hyperacute ischemia.
DWI and DTI
Around 95% of hyperacute infarcts show diffusion restriction on DWI with hyperintensity on DWI (8-22D) (8-24B)and corresponding hypointensity on ADC. DTI is even more sensitive than DWI, especially for small pontine and medullary lesions.
pMR
Restriction on DWI generally reflects the densely ischemic core of the infarct, whereas pMR depicts the surrounding “at-risk” penumbra. A DWI-PWI mismatch is one of the criteria used in determining suitability for EVT.
Angiography
Findings include abrupt vessel cut-off, a meniscus sign, tapered or “rat-tail” narrowing, or tram-track appearance (contrast around intraluminal thrombus) (8-26). Others are “bare” or “naked” area(s) of nonperfused brain, slow antegrade filling with intraarterial contrast persisting into the capillary or venous phase, and pial collaterals with retrograde filling across the cortical WS.
Less common signs are hyperemia with a vascular “blush” around the infarcted zone (so-called luxury perfusion) and “early draining” veins.
ACUTE STROKE IMAGING
NECT
• Hyperdense vessel ± dot sign
• “Blurred,” effaced GM-WM borders
Insular ribbon sign
“Disappearing” basal ganglia
• Wedge-shaped hypodensity
Involves both cortex, WM
• Look for Ca⁺⁺ emboli (≈ 50% risk of future stroke)
CECT
• ± enhancing vessels (slow flow, collaterals)
CTA
• Shows site, length of major vessel thrombus
• ASVD
Extracranial: Aorta, carotid bifurcation
Intracranial: Cavernous ICA, COW + branches
pCT
• Infarct core (irreversibly damaged brain)
Matched perfusion (CBV, CBF both decreased)
CBF < 30% (automated)
• Ischemic penumbra
Perfusion “mismatch” (decreased CBF but normal CBV)
Tmax > 6 (automated)
T1WI/T2WI
• Usually normal in first 4-6 hours
• ± loss of expected “flow void”
FLAIR (use narrow windows)
• 50% positive in first 4-6 hours
Cortical swelling, gyral hyperintensity
Intraarterial hyperintensity (usually slow flow, not thrombus)
T2* (GRE, SWI)
• Thrombus may “bloom”
• Large infarcts may show prominent hypointense medullary veins
• Microbleeds (chronic hypertension, amyloid)
DWI and DTI
• > 95% restriction within minutes
Hyperintense on DWI trace
Hypointense on ADC map
• “Diffusion-negative” acute strokes
Small (lacunar) infarcts
Brainstem lesions
Rapid clot lysis/recanalization
Transient/fluctuating hypoperfusion
pMR
• DWI-PWI “mismatch” estimates penumbra
• CBV-CBF, DWI-FLAIR mismatches estimate penumbra
DSA
• Vessel “cut-off,” meniscus sign, tapered/”rat-tail” narrowing
• “Bare” area of unperfused brain
• Slow antegrade or retrograde filling
• Delayed intraarterial contrast washout
• Luxury perfusion
“Blush” around “bare area”
“Early draining” veins
Differential Diagnosis
Stroke “mimics” with restricted DWI include infection, status epilepticus, and acute hypoglycemia. These typically affect cortex in a nonvascular distribution while sparing the underlying subcortical WM.
Normal circulating blood is always slightly hyperdense compared with brain on NECT. A hyperdense vessel sign can be simulated by elevated hematocrit (all the vessels appear dense, not just the arteries), arterial wall microcalcifications, and hypodense brain parenchyma (e.g., diffuse cerebral edema).
Subacute Cerebral Infarcts
Terminology
Strokes evolve pathophysiologically with corresponding changes reflected on imaging studies. Although there are no firm divisions that demarcate the various stages of stroke evolution, most neurologists designate infarcts as acute, subacute, and chronic.
“Subacute” cerebral ischemia/infarction generally refers to strokes that are between 48 hours and two weeks following the initial ischemic event.
Pathology
Edema and increasing mass effect caused by cytotoxic edema become maximal within 3-4 days following stroke onset. Frank tissue necrosis with progressive influx of microglia and macrophages around vessels ensues with reactive astrocytosis around the perimeter of the stroke. Brain softening and then cavitation proceeds over the next two weeks.
Most thromboembolic strokes are initially “bland,” i.e., nonhemorrhagic. Hemorrhagic transformation of a previously ischemic infarct occurs in 20-25% of cases between two days and a week after ictus (8-27). Ischemia-damaged vascular endothelium becomes “leaky,” and blood-brain barrier permeability increases. When reperfusion is established—either spontaneously or following treatment with tissue plasminogen activator—exudation of red blood cells through the damaged blood vessel walls causes parenchymal hemorrhages. Petechial hemorrhages are more common than lobar bleeds and are most common in the basal ganglia and cortex.
Clinical Issues
Hemorrhagic transformation itself, when small, generally does not cause clinical deterioration. Hemorrhagic transformation is actually related to favorable outcome, probably reflecting early vessel recanalization and better tissue reperfusion.
Imaging
General Features
There are significant variations within the subacute time period. Early subacute strokes have significant mass effect and often exhibit hemorrhagic transformation, whereas edema and mass effect have mostly subsided by the late subacute period.
CT Findings
On NECT, the wedge-shaped area of decreased attenuation seen on initial scans becomes more sharply defined. Mass effect initially increases, then begins to decrease by 7-10 days following stroke onset. Hemorrhagic transformation develops in 15-20% of cases and is seen as gyriform cortical or basal ganglia hyperdensity (8-28A).
CECT follows a “2-2-2” rule. Patchy or gyriform enhancement appears as early as two days after stroke onset, peaks at two weeks, and generally disappears by two months.
SUBACUTE STROKE
Pathology
• Edema, mass effect initially increase
• Vessel damage → hemorrhagic transformation in 25%
CT
• Hypodensity sharply defined
• Gyriform enhancement 2 days to several weeks
MR
• T1WI
Iso- to hypointense
May see T1 shortening (cortex, basal ganglia)
• T2WI
“Fogging effect” (isointensity)
± early wallerian degeneration
• FLAIR
Hyperintensity corresponds to final infarct
• T2*
“Blooming” hemorrhagic transformation
Prominent medullary veins
• DWI
Pseudonormalization
T2 “shine-through”
• T1 C+
Enhances (gyriform, even ring-like)
Differential Diagnosis
• Neoplasm
• Infection
Cerebritis
Encephalitis
MR Findings
Signal intensity in subacute stroke varies depending on (1) time since ictus and (2) the presence or absence of hemorrhagic transformation.
T1WI
Nonhemorrhagic subacute infarcts are hypointense on T1WI and demonstrate moderate mass effect with sulcal effacement. Strokes with hemorrhagic transformation are initially isointense with cortex and then become hyperintense (8-29).
T2WI
Subacute infarcts are initially hyperintense compared with nonischemic brain. Signal intensity decreases with time, reaching isointensity at 1-2 weeks (the T2 “fogging effect”) (8-30). Early wallerian degeneration can sometimes be identified as a well-delineated hyperintense band that extends inferiorly from the infarcted cortex along the corticospinal tract.
FLAIR
Subacute infarcts are hyperintense on FLAIR (8-28B). By one week after ictus, “final” infarct volume corresponds to the FLAIR-defined abnormality.
T2* (GRE, SWI)
Petechial or gyriform “blooming” foci are present if hemorrhagic transformation has occurred in the infarcted cortex (8-28B). Basal ganglia hemorrhages can be confluent or petechial.
Prominent ipsilateral medullary veins on SWI in MCA territory strokes within 3-7 days of ictus are a significant predictive biomarker of poor clinical outcome. Prominent medullary veins in the contralateral (normal) hemisphere may indirectly reflect increased CBF and are associated with good clinical outcome.
T1 C+
The intravascular enhancement often seen in the first 48 hours following thromboembolic occlusion disappears within three or four days and is replaced by leptomeningeal enhancement caused by persisting pial collateral blood flow. Patchy or gyriform parenchymal enhancement can occur as early as two or three days after infarction (8-29)and may persist for 2-3 months, in some cases, mimicking neoplasm (8-31).
DWI and pMR
Restricted diffusion with hyperintensity on DWI and hypointensity on ADC persists for the first several days following stroke onset, then gradually reverses to become hypointense on DWI and hyperintense with T2 “shine-through” on ADC. pMR with arterial spin labeling shows crossed cerebellar diaschisis in 50% of patients with subacute ischemic strokes.
Chronic Cerebral Infarcts
Terminology
Chronic cerebral infarcts are the end result of ischemic territorial strokes and are also called postinfarction encephalomalacia.
Pathology
The pathologic hallmark of chronic cerebral infarcts is volume loss with gliosis in an anatomic vascular distribution. A cavitated, encephalomalacic brain with strands of residual glial tissue and traversing blood vessels is the usual gross appearance of an old infarct (8-32A).
Imaging
NECT scans show a sharply delineated wedge-shaped hypodense area that involves both GM and WM and conforms to the vascular territory of a cerebral artery. The adjacent sulci and ipsilateral ventricle enlarge secondary to volume loss in the affected hemisphere (8-32A). Dystrophic calcification occurs but is uncommon (8-33).
Wallerian degeneration with an ipsilateral small, shrunken cerebral peduncle is often present with large MCA infarcts. Look for atrophy of the contralateral cerebellum secondary to crossed cerebellar diaschisis.
Chronic infarcts older than 2-3 months typically do not enhance on CECT.
MR scans show cystic encephalomalacia with CSF-equivalent signal intensity on all sequences. Marginal gliosis or spongiosis around the old cavitated stroke is hyperintense on FLAIR (8-32B). DWI shows increased diffusivity (hyperintense on ADC).
Multiple Embolic Infarcts
Brain emboli are less common but important causes of stroke. Most consist of clots containing fibrin, platelets, and red blood cells. Less common emboli include air, fat, calcium, tumor, and foreign bodies (e.g., debris from metallic heart valves).
Cardiac and Atheromatous Emboli
Pathoetiology
Simultaneous small acute infarcts in multiple different vascular distributions are the hallmark of embolic cerebral infarcts (8-35). The heart is the most common source; cardiac emboli can be septic or aseptic (8-37). Peripheral signs of emboli, such as splinter hemorrhages, are sometimes present. Echocardiography may demonstrate valvular vegetations, intracardiac filling defect, or atrial or ventricular septal defect.
Ipsilateral hemispheric emboli are most commonly due to atheromatous ICA plaques. Many are clinically silent but convey a high risk for subsequent overt stroke.
Imaging
In contrast to large artery territorial strokes, embolic infarcts tend to involve terminal cortical branches. The GM-WM interface is most commonly affected.
NECT scans show low-attenuation foci, often in a wedge-shaped distribution. Calcified emboli (usually from heart valves or atherosclerotic plaques) are seen as small round or ovoid calcifications within sulci (8-34). These are important to identify, as they carry a high risk of recurrent stroke. Septic emboli are often hemorrhagic. CECT scans may demonstrate multiple punctate or ring-enhancing lesions.
MR scans show multifocal peripheral T2/FLAIR hyperintensities. Hemorrhagic emboli cause “blooming” on T2* sequences (8-36). The most sensitive sequence is DWI. Small peripheral foci of diffusion restriction in several different vascular distributions are typical of multiple embolic infarcts (8-37B). T1 C+ imaging may show multiple punctate enhancing foci. Septic emboli often demonstrate ring enhancement, resembling microabscesses.
Differential Diagnosis
The major differential diagnosis of multiple embolic infarcts is hypotensive cerebral infarction. Hypotensive infarcts are usually caused by hemodynamic compromise and tend to involve the deep internal WS zones. Parenchymal metastases have a predilection for the GM-WM interface, as do embolic infarcts, but generally do not restrict on DWI.
Fat Emboli
Fat embolism syndrome (FES) is an uncommon disorder that presents as hypoxia, neurologic symptoms, &/or a petechial rash in the setting of severely displaced lower extremity long bone fractures. The term cerebral fat emboli (CFE) refers to the neurologic manifestations of FES.
Pathoetiology
Two mechanisms have been proposed to explain the effects of FES: (1) Small vessel occlusions from fat particles and (2) inflammatory changes in surrounding tissue initiated by breakdown of fat into free fatty acids and other metabolic byproducts.
The pathologic hallmark of CFE is arteriolar fat emboli with perivascular microhemorrhages (8-38).
Epidemiology and Clinical Issues
The overall incidence of FES in patients with long bone fractures—most commonly the femoral neck—is 1-2%. FES also occurs with pelvic fractures, elective orthopedic procedures (e.g., total hip arthroplasty), cardiac surgery, anesthesia, and systemic illness (e.g., pancreatitis). FES in patients with bone marrow necrosis secondary to sickle cell crisis has also been reported.
CFE occurs in up to 80% of patients with FES. Signs and symptoms vary in severity and include petechial rash, headache, seizure, drowsiness, altered mental status, and coma. Focal neurologic deficits are less common. Onset is from two hours up to two days after trauma or surgery with a mean of 29 hours. However, many cases are subclinical and remain undiagnosed.
Imaging
Imaging findings reflect the effect of the fat emboli (i.e., multifocal tiny strokes and microhemorrhages) on brain tissue, not the fat itself. NECT scans are, therefore, usually normal.
MR shows numerous (average = 50) punctate or confluent hyperintensities in the cerebellum, basal ganglia, periventricular WM, and GM-WM junctions on T2/FLAIR (8-39A). DWI shows innumerable tiny punctate foci of diffusion restriction in multiple vascular distributions, the star field pattern (8-39B). The deep WS border zones are commonly involved.
Solitary or multiple small hypointense “blooming” foci can be identified in up to 1/3 of all FES cases on T2* GRE. SWI discloses innumerable (> 200) tiny “black dots” in the majority of patients (8-39C).
Differential Diagnosis
The major differential diagnosis of cerebral FES is multiple embolic infarcts. Multiple cardiac or atheromatous embolic infarcts rarely produce the dozens or even hundreds of lesions seen with CFE. Embolic infarct lesions tend to involve the basal ganglia and corticomedullary junctions more than the WM.
Multifocal “blooming” hypointensities on T2* can be seen with severe diffuse axonal injury (DAI)or diffuse vascular injury (DVI). As patients with CFE often have polytrauma, the distinction may be difficult on the basis of imaging studies alone. DAI and DVI tend to cause linear as well as punctate microbleeds.
Cerebral Gas Embolism
Pathoetiology
Minor amounts of air in the intracranial venous systems is usually iatrogenic, introduced during intravenous catheter placement.
Massive cerebral gas embolism (CGE) is a potentially catastrophic complication of central venous catheter (CVC) manipulation/disconnection and has been reported with cardiac procedures.
Other etiologies of arterial or venous air embolism include lung biopsy, craniotomy in the sitting position, and angiography. Penetrating trauma, decompression sickness, and hydrogen peroxide ingestion are other causes of gas embolism.
Clinical
Small amounts of CGE may be asymptomatic or mild and transient. In more severe cases, focal neurologic deficit, coma, seizures, and encephalopathy may ensue. Reported mortality of CGE associated with CVCs approaches 20%.
Imaging
Asymptomatic air following intravenous catheter placement is most commonly observed as an incidental finding, typically as dots of air in the cavernous sinus.
Intracranial air bubbles can be identified in 70% of symptomatic CGE cases, appearing on NECT as transient small intravascular rounded or curvilinear hypodensities, typically located at the depths of sulci (8-40A). Intraparenchymal air is less common.
Air is quickly absorbed and can rapidly disappear (8-41). If massive air embolism occurs, cerebral ischemia or diffuse brain swelling typically ensues (8-41).
EMBOLIC INFARCTS
Cardiac and Atheromatous Emboli
• Small, simultaneous; multiple lesions
• Bilateral, multiple vascular distributions
• Typically involve cortex, GM-WM interfaces
• May be Ca⁺⁺
• ± punctate/ring enhancement
• Usually not hemorrhagic unless septic
Fat Emboli
• 12-72 hours after long bone trauma, surgery
• Less commonly from bone marrow necrosis (e.g., sickle cell crisis)
• Arteriolar/capillary fat emboli
• Cause multiple tiny microbleeds
• Multiple foci of restricted diffusion in star field pattern (bright spots on dark background)
• Microbleeds best seen on T2* SWI > > GRE
• Deep WM > cortex
Cerebral Gas Embolism
• Usually iatrogenic (procedural) or traumatic
• Can occur with hydrogen peroxide ingestion
• NECT may show transient round or curvilinear air densities in sulcal vessels
• Quickly absorbed, disappear
• If massive, lethal brain swelling ensues rapidly
Lacunar Infarcts
Terminology
The terms “lacuna,” “lacunar infarct,” and “lacunar stroke” are often used interchangeably. Lacunae are 3- to 15-mm, CSF-filled cavities or “holes” that most often occur in the basal ganglia or cerebral WM (8-42). They are often observed coincidentally on imaging studies in older patients but are not clearly associated with discrete neurologic symptoms, i.e., they are subclinical strokes. Lacunae are sometimes called “silent” strokes, a misnomer, as subtle neuropsychologic impairment is common in these patients.
Lacunar stroke means a clinically evident stroke syndrome attributed to a small subcortical or brainstem lesion that may or may not be evident on brain imaging. The term “état lacunaire” or lacunar state designates multiple lacunar infarcts.
Epidemiology and Etiology
Approximately 25% of all ischemic strokes are lacunar-type infarcts. Lacunae are considered macroscopic markers of cerebral small vessel (“microvascular”) disease. There are two major vascular pathologies involving small penetrating arteries and arterioles: (1) Thickening of the arterial media by lipohyalinosis, fibrinoid necrosis, and atherosclerosis, causing luminal narrowing and (2) obstruction of penetrating arteries at their origin by large intimal plaques in the parent arteries.
The MTHFR C677T genotype is correlated with lacunar stroke.
Pathology
Location
Penetrating branches that arise from the COW and peripheral cortical arteries are small end arteries with few collaterals, so lacunar infarcts are most common in the basal ganglia (putamen, globus pallidus, caudate nucleus), thalami, internal capsule, deep cerebral WM, and pons.
Size and Number
Lacunae are, by definition, ≤ 15 mm in diameter. Multiple lesions are common. Between 13-15% of patients have multiple simultaneous acute lacunar infarcts.
Gross and Microscopic Appearance
Grossly, lacunae appear as small, pale, irregular but relatively well-delineated cystic cavities (8-43). Brown-staining siderotic discoloration can be seen in old hemorrhagic lacunae. Microscopically, ischemic lacunar infarcts demonstrate tissue rarefaction with neuronal loss, peripheral macrophage infiltration, and gliosis.
Clinical Issues
Independent risk factors for lacunar infarcts include age, hypertension, and diabetes. Other contributing factors include smoking and atrial fibrillation.
Outcome of lacunar stroke is highly variable. Although most lacunae are asymptomatic, “little strokes” can mean “big trouble.” A single subclinical stroke—often a lacuna—is associated with increased likelihood of having additional “little strokes” as well as developing overt clinical stroke &/or dementia. Nearly 20% of patients > 65 years old with WM hyperintensities (WMHs) on T2/FLAIR MR will develop new lacunae within three years.
Between 20-30% of patients with lacunar stroke experience neurologic deterioration hours or even days after the initial event. The pathophysiology of “progressive lacunar stroke” is incompletely understood, and no treatment has been proven to prevent or halt progression.
Imaging
Imaging findings vary with whether the lacuna is acute or chronic.
Acute Lacunar Infarcts
Most acute lacunar infarcts are invisible on NECT scans. Acute lacunar infarcts are hyperintense on T2/FLAIR and may be difficult to distinguish from foci of coexisting chronic microvascular disease (8-45)(8-46A). Acute and early subacute lacunae restrict on DWI (8-46B) and also usually enhance on T1 C+.
DWI overestimates the eventual size of lacunar infarcts. Cavitation and lesion shrinkage are seen in > 95% of deep symptomatic lacunar infarcts on follow-up imaging.
LACUNAR INFARCTS
Etiology and Pathology
• Macroscopic markers of small vessel disease
• Atherosclerosis, lipohyalinosis
• Along small penetrating arteries (few collaterals)
• Most common in basal ganglia, deep WM
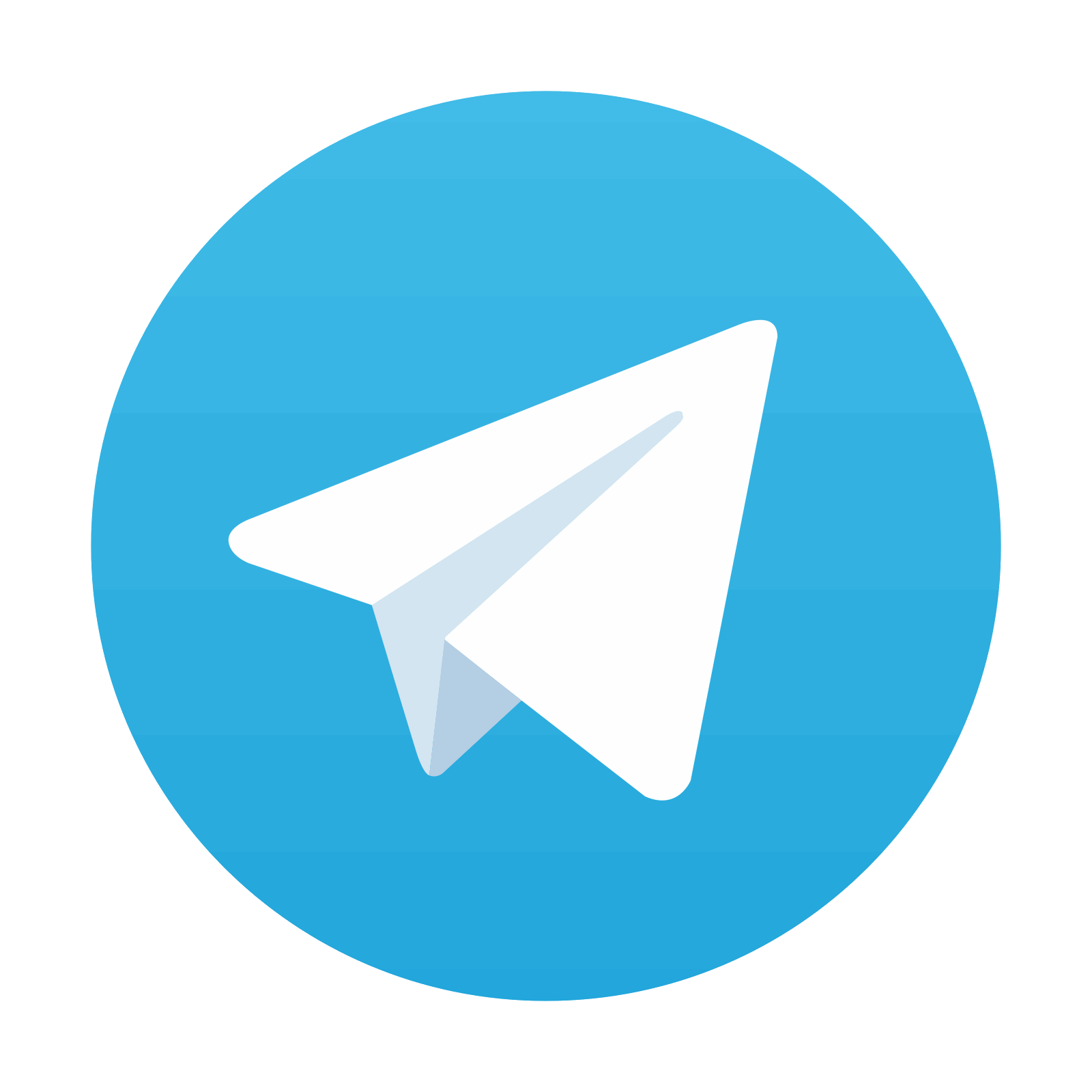
Stay updated, free articles. Join our Telegram channel

Full access? Get Clinical Tree
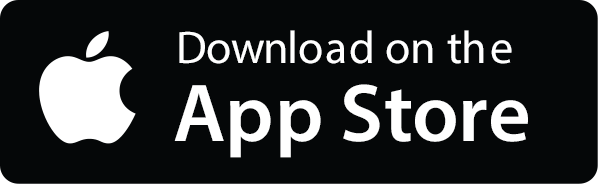
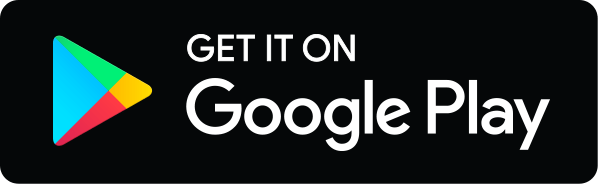