h1 class=”calibre8″>6 Atlanto-occipital Injuries
Abstract
Injuries to the upper cervical spine are common after high-energy trauma and are associated with high morbidity and mortality. Rapid diagnosis and appropriate treatment of these injuries lead to improved mortality and patient outcomes. Injuries to the atlas, occipital condyles, and the craniocervical junction, including the atlanto-occipital articulation, are the focus of this chapter. The relevant anatomy, diagnosis, classification systems, and treatment options are discussed.
Keywords: atlas fracture, occipital condyle fracture, Jefferson fracture, atlanto-occipital dissociation, craniocervical dissociation
6.1 Introduction
Injuries between the occiput and C2 constitute “upper cervical spine injuries,” and these are common after high-energy trauma. The magnitude and the direction of the impact force may vary, from direct axial loading, to bending in the sagittal and coronal plane, or rarely, in rotation. The complex anatomy of the craniocervical junction and the variable mechanism of injury lead to a wide spectrum of injuries. The upper cervical spine allows for approximately 50% of the total cervical spine rotation, and 20% of the anterior, posterior, and lateral bending.
This chapter will focus on injuries of the atlas, the occipital condyle, and the craniocervical junction. Injuries to the axis and the atlantoaxial articulation will be discussed elsewhere. These areas are anatomically and biomechanically related, and injury to one area may occur concomitantly with injury to another.
6.2 Anatomy
6.2.1 Occiput
The occipital bone forms the foramen magnum. The occipital condyles are semilunar projections from the inferior surface of the occiput that lie within the concavities of the lateral masses of the atlas, forming a shallow ball-and-socket configuration. The atlanto-occipital joint articulation is stabilized by the alar ligaments, which are paired, cord-like structures that project laterally from the tips of the odontoid process to the inner aspect of each occipital condyle, and by the tectorial membrane, which is the cranial continuation of the posterior longitudinal ligaments to the anterior aspect of the foramen magnum. The tectorial membrane and the paired alar ligaments are crucial for craniocervical stability. 1 The alar ligaments are slack when the head is in mid position. As the head rotates toward one direction, the alar ligament contralateral to the direction of rotation tightens, while the ipsilateral ligament slackens. Together with the tectorial membrane, the alar ligaments also limit flexion, but play no role in limiting extension.
Anteriorly, the well-developed atlanto-occipital membrane, an extension of the anterior longitudinal ligament, limits extension. Structures such as the apical ligaments, the occipitocervical membrane, and the atlanto-occipital joint capsules provide little intrinsic stability. Normally, the atlanto-occipital joints have a congruous relationship, and more than 2 mm of diastasis suggests a ligamentous disruption and unstable injury 2 (▶ Fig. 6.1c).
Fig. 6.1 Axial computed tomography (CT) scan of the atlas (a) shows the vertebral arteries (white circle) and the transverse alar ligament (black lines). Coronal image (b) shows the relationship of the occipital condyles (OC), atlas lateral masses, and axis. Parasagittal image (c) demonstrates the condyle–C1 interval (thin white arrows). Mid-sagittal view (d) shows the basion–dens interval (black arrowheads), the basion–axis interval (white line).
6.2.2 Atlas
The atlas is a ring-shaped structure with large lateral masses and thin anterior and posterior connecting arches (▶ Fig. 6.1a). The lateral masses of the atlas articulate superiorly with the occipital condyles, and inferiorly with the lateral masses of the axis (▶ Fig. 6.1b). In the coronal plane, the atlas lateral masses are trapezoid in shape. The articulation of atlanto-occipital joint is sloped from inferior medially to superior laterally. The articulation of the atlantoaxial joint is sloped from superior medially to inferior laterally. This configuration results in transferring of axial loading forces onto the atlas lateral masses to be directed laterally, causing the typical “burst” pattern of injury.
The cruciate ligament lies behind the dens and consists of the transverse ligament and fibers that attach cranially to the basion and caudally to the axis. The transverse ligament, which passes around the dens at its waist, attaches to the tubercles on the medial side of each atlas lateral mass (▶ Fig. 6.1d). The atlantoaxial articulation allows for 45 degrees of axial rotation in each direction, limited in part by tightening of the opposite alar ligament. It accounts for 50% of the rotation of the cervical spine and allows for 20 to 30 degrees of flexion-extension. 3,4 Werne et al have shown the atlanto-occipital articulation allows for 15 degrees of flexion-extension, 8 degrees of lateral bending, and 0 degrees of axial rotation. 5,6
Steel’s “rule of thirds” states that at the level of the atlas, the odontoid process takes up one-third of the total space, the spinal cord takes up one-third of the space, and the cerebrospinal fluid space constitutes the remaining one-third. 7
6.2.3 Axis
The axis has a large projection, called the dens or odontoid process. The dens lies behind the anterior arch of the atlas, and the transverse ligament wraps behind the dens, providing stability and prevents anterior-to-posterior atlantoaxial shear.
6.2.4 Vertebral Arteries
The vertebral arteries are paired vessels that typically arise from the right and left subclavian arteries. Rarely, the vertebral artery could arise directly from the aortic arch itself. 39 The vertebral arteries ascend cephalad to enter the transverse foramina typically at the C6 vertebral segment. In 12% of the cases, the vertebral arteries enter the foramen at other vertebral levels. The vertebral artery is divided into following four segments:
The V1 (extraosseous) segment courses cephalad from the subclavian arteries to enter the transverse foramen of the C6 vertebra.
The V2 (foraminal) segment ascends vertically through the C6 to C3 transverse foramina, turns laterally to exit the C2 foramen, then turns medially once it exits above the C1 foramen.
The V3 (extraspinal) segment begins as the artery exits from the C1 foramen and ends when it penetrates the dura. The vertebral artery forms a prominent groove along the posterior arch of the atlas.
The V4 (intradural) segment courses anteromedially through the foramen magnum and joins the contralateral vertebral artery to form the basilar artery at the pontomedullary junction.
Burst fractures of the atlas with displacement could be associated with injuries to the V3 (extraspinal) segment of the vertebral artery. Ponticulus posticus is an osseous anomaly of the atlas, characterized by the presence of an arcuate foramen. This is a poorly recognized anatomic anomaly that is present in up to 15.5% of the patients; its proper recognition is important to avoid injury to the vertebral artery during exposure or placement of C1 lateral mass screws. 40
6.3 Physical Exam
Upper cervical spine injuries often occur as result of high-energy trauma resulting in skull impaction. Partial or total loss of consciousness is often reported. Alert patients may complain of upper cervical pain to movement or palpation. Various neurological syndromes have been described in patients with upper cervical spine injuries, especially involving cranial nerve VI and the lower cranial nerves IX, X, XI, and XII. Full neurological assessment should be performed according to the American Spinal Injury Association (ASIA) guidelines.
6.4 Radiographic Exam
Upper cervical spine injuries are best evaluated with computed tomography (CT) imaging with sagittal and coronal reconstruction. CT scan has been shown to be timely, cost effective, and more sensitive and specific in high-risk trauma patients. 2 On CT imaging, the atlanto-occipital joint should be congruous, and greater than 2 mm of diastasis of the articulation should prompt concern for atlanto-occipital dissociation (AOD). Pang et al reported an average of 1.28 mm of condyle–C1 interval (CCI) in normal children, and none of the CCIs exceeded 1.95 mm. 8,37 The Harris’ “rule of twelves” indicates that the distance from the tip of the dens to the basion (basion–dental interval) should be less than 12 mm, and the basion–axial interval, which is the distance from the basion to the posterior axial line, should also be less than 12 mm. Violation of this rule should prompt concern for AOD. 9,10
The atlantodental interval (ADI) is the distance between the anterior atlas arch and the odontoid process. In adults, ADI less than 3 mm is considered normal, while in children, less than 5 mm is normal. ADI greater than this indicates potential disruption of the transverse ligament and potential atlantoaxial instability.
Magnetic resonance imaging (MRI) focused toward the skull base or the craniocervical junction may be useful to rule out ligamentous injury when diagnosis is not clear. Indicators of unstable injury include significant prevertebral soft-tissue edema or hematoma, increased joint edema in atlanto-occipital or atlantoaxial joints, tectorial membrane disruption, transverse ligament or alar ligament disruption, or subarachnoid hemorrhage.
6.5 Occipital Condyle Injury
6.5.1 Introduction
Traumatic occipital condyle fracture (OCF) was first described by Sir Charles Bell in 1817 based on autopsy of a patient from a fall. 11 Plain radiographs rarely visualize OCFs. Improvement in advanced imaging and more prevalent use of CT imaging over the past two decades have led to an increase in detection of this injury. Except when associated with craniocervical instability, OCFs are relatively benign and are usually managed conservatively. OCFs occur in 1 to 4% of patients with traumatic brain injury 12 (▶ Fig. 6.2).
Fig. 6.2 Axial (a) and coronal (b) computed tomography (CT) scans show a type I right occipital condyle fracture in a 54-year-old male who was an unrestrained passenger in a motor vehicle crash. No ligamentous injury was noted on magnetic resonance imaging, and he was successfully treated with 6 weeks of hard cervical collar immobilization.
The most common mechanisms of injury are from impaction of skull onto the cervical spine or rapid head deceleration. OCFs should be suspected in patients with high-energy head trauma, altered mental status, upper cervical spine tenderness, lower cranial nerve deficits, and retropharyngeal hematoma or edema. Cranial nerve XII, the hypoglossal nerve, travels in the hypoglossal canal superior to the occipital condyle and may be injured with OCF.
6.5.2 Classification
The most commonly utilized classification system was proposed in 1988 by Anderson and Montesano that classifies OCF into following three types: 13
Type I fractures are comminuted fractures secondary to axial loading and impaction of the occipital condyle on the lateral masses of the atlas. These are stable (▶ Fig. 6.2).
Type II fractures are extension of a linear basilar skull base fracture.
Type III fractures are traumatic avulsion of a fragment via the tensile forces of the alar ligament. These may be potentially unstable and may be associated with craniocervical dissociation (CCD) (▶ Fig. 6.3).
Fig. 6.3 Sagittal reformatted computed tomography (CT) scan showing left (a) and right (b) images of a 44-year-old male who was brought in after automobile-versus-pedestrian accident, demonstrating type III occipital condyle fractures, and increased condyle-to-C1 interval. Axial (c) and coronal (d) images demonstrate bilateral occipital condyle fractures. The patient remained comatose and succumbed to his concomitant brain injury.
Type I fractures are stable and cervical collar immobilization is recommended for these. Type II fractures are typically stable, except in the rare instance when the entire condyle is detached, making the atlanto-occipital articulation unstable. Cervical collar immobilization is recommended for stable type II fractures, whereas halo vest should be considered for unstable type II fracture with incompetent alar ligament and displacement of atlanto-occipital alignment. Type III fracture should prompt evaluation for possible AOD with MRI of the craniocervical junction. Evaluation of the atlanto-occipital articulation is essential to determine the integrity of the alar ligaments. 1 Presence of AOD should prompt consideration for occiput-cervical instrumented fusion. Otherwise, a stable type III fracture could be treated with cervical collar immobilization.
In a review of current literature, Theodore et al identified 415 patients with OCF, with 84 type I, 125 type II and 207 type II unilateral OCFs, and 37 bilateral OCFs. They are relatively uncommon injuries, with various reports of 1 to 3% frequency in patients sustaining high-energy, blunt craniocervical trauma. 11,14,15
OCFs are typically diagnosed with CT imaging, as plain radiographs typically have low sensitivity. 11 Of the 415 patients with OCF described in the literature, clinical information was provided for 119 patients. Normal neurological exam was reported in 35 patients (30%). Loss of consciousness was reported in 36 patients (30%), and focal neurological deficit was reported in 48 patients (40%), which included cranial nerve deficits alone, cranial nerve deficit with limb weakness, mild to severe limb weakness without cranial nerve deficits, vertigo, hyperreflexia, and diplopia.
6.5.3 Treatment
Of the 415 patients with OCF reported in the literature, treatment information is offered on 259 patients. Of the 43 OCF patients who did not receive treatment, 9 patients developed cranial nerve deficits within days to weeks after injury. One hypoglossal nerve palsy resolved, two hypoglossal nerve deficits improved, three cranial nerve deficits persisted, and outcomes for three cases were not reported. Six additional patients were identified with untreated OCFs who developed delayed deficits or symptoms. 11
One hundred ninety patients with OCF were initially treated with cervical collar immobilization. Sixty-eight of those patients had complete recovery at last follow-up. Outcome following the treatment is inconsistently reported, but one patient had modest reduction in neck rotation after treatment, one had hypoglossal nerve deficit at last follow-up, two patients had persistent mild dysphonia, and three had persistent neck pain. Thirty-two patients with OCF were treated with halo/Minerva immobilization devices. One had slight improvement of Collet–Sicard syndrome, another had persistent trapezius weakness, two had chronic neck pain, and two had complete recovery at last follow-up. 11
Seventeen patients with OCF were treated with surgery. Fourteen of these underwent occiput-cervical internal fixation and fusion (1 unknown type, 2 type II, and 11 type III). Three of these patients underwent surgery for decompression of the brainstem (one type II and two type III injuries) in addition to internal fixation and fusion. One patient with delayed diplopia had symptom resolution after removal of fracture fragment, one patient with lower cranial nerve deficit and one with diplopia and hemiparesis remained unchanged several days after surgery. 11
Hanson et al retrospectively reviewed 95 patients with 107 OCFs. Three patients had type I fractures, 24 fractures in 23 patients were type II fracture, and 69 patients had 80 type III fractures (11 were bilateral). Unilateral injury was present in 77% of the cases. Eight patients had craniocervical instability and were treated surgically with occiput-cervical fusion, and four patients were treated with halo vest. Sixty patients (63%) had evidence of diffuse head injury or focal intracranial hematoma. Long-term outcomes were more correlated to associated traumatic brain injury, rather than the OCF itself. 16
Maserati et al reviewed their series of 100 patients with 106 OCFs. The incidence of OCF was 0.4%. Two patients who had AOD and one patient with C1–C2 fracture were treated surgically. The remaining patients were treated with rigid collar or counseling alone. No patients developed delayed craniocervical instability, nor delayed neural element compression, or cranial neuropathy. The authors conclude that further classification of OCFs is unnecessary, and that management should consist of occiput-cervical fusion or halo vest for cases demonstrating occipitocervical misalignment, and rigid collar immobilization for 6 weeks with radiographic and clinical follow-up for cases where misalignment is not present. 17
Patient outcomes are correlated with the presence of concurrent craniocervical trauma, neural element compression from fragments, or associated AOD. Overall, nonoperative treatment with external cervical immobilization is usually sufficient to promote bony healing, and resolution or improvement in lower cranial nerve deficits. Isolated bilateral OCF should prompt consideration of more rigid external immobilization, 11 while OCF associated with atlanto-occipital injury should prompt consideration for surgical stabilization or halo vest immobilization.
6.5.4 Conclusion
OCF is a relatively uncommon injury that requires CT imaging to evaluate. CT scan of the craniocervical junction should be considered in anyone who presents with high-energy trauma to the craniocervical junction, particularly with clinical presentation of neck pain, lower cranial nerve deficits, or decreased level or loss of consciousness. Treatment with cervical collar immobilization is typically sufficient for almost all types of OCF. Untreated OCF may rarely develop acute or delayed lower cranial nerve palsies, which typically resolve with collar immobilization. Bilateral OCF should prompt consideration for more rigid immobilization. OCF with evidence of instability, such as atlanto-occipital or atlantoaxial dissociation, should prompt consideration for surgical fixation and fusion. Neural element compression from avulsed and displaced fracture fragment should also prompt consideration for surgical decompression, fixation, and fusion.
6.6 Atlas Fractures
6.6.1 Introduction
Atlas fractures account for 2 to 13% of acute injuries of the cervical spine and 1 to 2% of all spine injuries. Atlas fractures occur due to traumatic axial loading and 5 to 53% of these will have associated axial or other cervical spine injuries. 18 Approximately 21% of patients with an atlas fracture will also have an associated head injury. The majority of these fractures are a result of motor vehicle collisions and motorcycle collisions. However, diving into shallow waters, fall, and assault can also be the reasons for these injuries.
Atlas fractures are rarely associated with neurological deficit, and it is generally believed to be due to the large diameter of the spinal canal at this level and the orientation of the atlas lateral masses. Axial loading forces the lateral masses out laterally, away from the spinal cord, with resultant increase in the size of the spinal canal.
The mechanisms of injury are usually due to axial loading forces from blows to the cranium and hyperextension. The forces are resisted first by the anterior and posterior arches, then by the transverse ligament, and finally by the alar ligaments.
6.6.2 Classification
Because of the ringed anatomy of C1, a single fracture is highly unlikely. Displacement of the ring structure of the atlas requires at least a minimum of two fractures. Atlas fractures can be divided into several patterns based on location of the injury, mechanism of injury, and the integrity of the transverse ligament.
Type I injuries are isolated fractures of the posterior arch and are the most common injuries. This is typically due to hyperextension, with the posterior atlas arch being caught between the occiput and the axis arch. Fracture occurs at the weakest portion of the posterior arch at its attachment to the lateral masses. Note that the vertebral arteries course over the grooves on the posterior arch of the atlas, therefore a displaced fracture may cause vertebral artery injury.
Type II injuries are burst fractures of the atlas ring, classically referred to as “Jefferson fracture.” First described in 1920 by Sir Geoffrey Jefferson, this injury typically presents with four bony fractures, with two fractures through the anterior arch and two fractures through the posterior arch. 19 Jefferson described the mechanism of injury as direct vertical compression of the atlas between the occipital condyles and the axis lateral masses with the neck in neutral position. Fracture occurs at the weakest areas of the anterior and posterior rings: at their attachments to the lateral masses. The axial loading vector, along with the sloped orientation of the atlas lateral masses, force the C1 lateral masses laterally (i.e., away from the spinal cord). The transverse ligament, which is attached to the tubercles in the medial lateral masses, is progressively tensioned and fails secondary to midsubstance tear or avulsion from the bony tubercle (▶ Fig. 6.4).
Fig. 6.4 Axial computed tomography (CT) scans of a 25-year-old female who suffered occipital condyle fractures (a) and Jefferson burst fracture of the atlas (b). Sagittal (c) and right parasagittal (d) images show increased atlas–dens interval, ADI (black arrow), and increased condyle–C1 interval (white arrow). (continued)
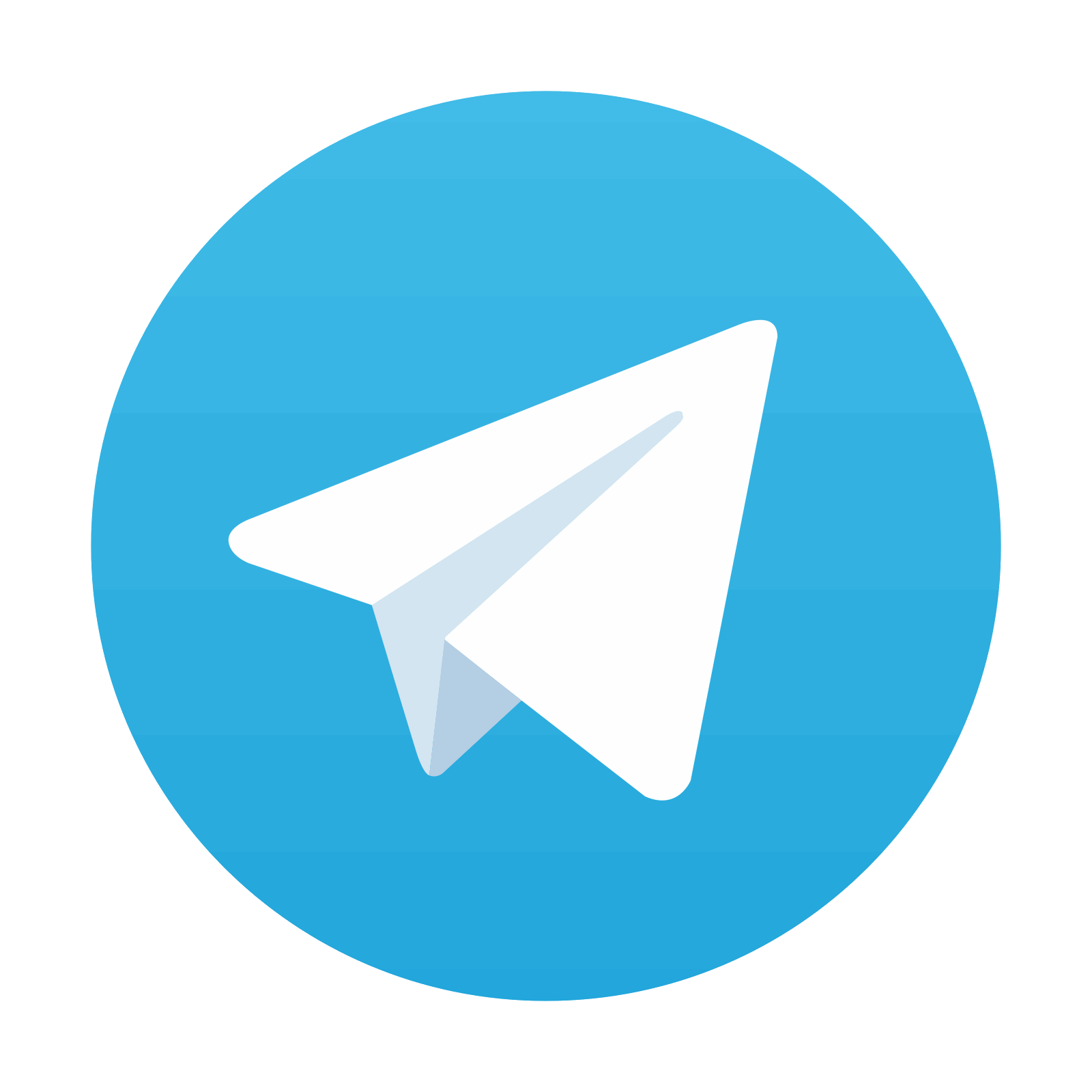
Stay updated, free articles. Join our Telegram channel

Full access? Get Clinical Tree
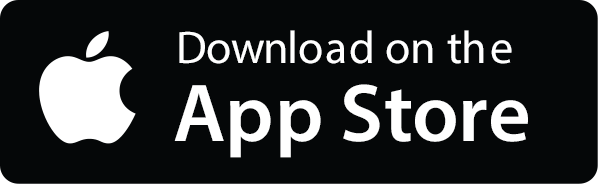
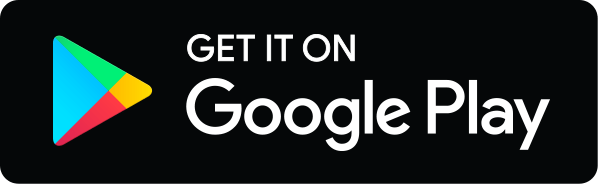