(A1) Inattention: six (or more) of the following symptoms persisting for at least 6 months to a degree that is maladaptive and inconsistent with developmental level
• Often fails to give close attention to details or makes careless mistakes in schoolwork, work or other activities
• Often has difficulty sustaining attention in tasks or play activities
• Often does not seem to listen when spoken to directly
• Often does not follow through on instructions and fails to finish schoolwork, chores or duties in the workplace (not due to oppositional behaviour or failure to understand instructions)
• Often has difficulty organising tasks and activities
• Often avoids, dislikes or is reluctant to engage in tasks that require sustained mental effort (such as schoolwork or homework)
• Often loses things necessary for tasks or activities (e.g. toys, school assignments, pencils, books or tools)
• Is often easily distracted by extraneous stimuli
• Is often forgetful in daily activities
(A2) Hyperactivity–impulsivity: six (or more) of the following symptoms persisting for at least 6 months to a degree that is maladaptive and inconsistent with developmental level
Hyperactivity
• Often fidgets with hands or feet or squirms in seat
• Often leaves seat in classroom or in other situations in which remaining seated is expected
• Often runs about or climbs excessively in situations in which it is inappropriate (in adolescents or adults, may be limited to subjective feelings of restlessness)
• Often has difficulty playing or engaging in leisure activities quietly
• Is often “on the go” or often acts as if “driven by a motor”
• Often talks excessively
Impulsivity
• Often blurts out answers before questions have been completed
• Often has difficulty awaiting turn
• Often interrupts or intrudes on others (e.g. butts into conversations or games)
Other criteria for diagnosis
(B) Some hyperactive–impulsive or inattentive symptoms that caused impairment were present before age 7 years
(C) Some impairment from the symptoms is present in two or more settings (e.g. at school [or work] and at home)
(D) There must be clear evidence of clinically significant impairment in social, academic or occupational functioning
(E) The symptoms do not occur exclusively during the course of a pervasive developmental disorder, schizophrenia or other psychotic disorder and are not better accounted for by another mental disorder (e.g. mood disorder, anxiety disorder, dissociative disorder or personality disorder)
Latent class analysis has been used to address this question by examining the clustering of the two domains within families. While there was some evidence for the ADHD subtypes of ADHD-I and ADHD-CT to co-occur above chance levels within a family, suggesting the familial influences are shared across the two subtypes (Faraone, Biederman, & Friedman, 2000; Todd et al., 2001), evidence also exists for the segregation of subtypes across families, suggesting distinct familial influences to the subtypes (Smalley et al., 2000; Stawicki, Nigg, & von Eye, 2006; Takeda et al., 2010). This idea that familial influences are specific to each subtype was reflected in the results of a meta-analysis (Stawicki et al., 2006). Despite inconsistent results across individual studies, the pooled data consistently suggested the occurrence of subtype-specific transmission to some degree, but the effect is small, and especially in families of children with ADHD-CT, non-specific transmission also occurs. The authors claim that the much-debated relation between the two DSM-IV ADHD subtypes is best modeled via two processes transmitting in families: one process is distinct for the two syndromes, and the other is shared.
By looking at the two symptom domains separately, twin studies have been able to address whether the hyperactivity–impulsivity and inattentiveness reflect the same or separable aetiological pathways. An early twin study indicates both substantial genetic overlap and some specificity to the two domains, with a report of genetic correlations of 0.58 for teacher ratings and 0.60 for parent ratings for author-derived domain-specific subscales based on DSM-III criteria (Sherman, Iacono, & McGue, 1997). This was followed by a report suggesting that the genetics of the ADHD symptom domains of hyperactivity, inattentiveness and, separately, impulsivity could be reduced to that of a single genetic factor (Nadder, Rutter, Silberg, Maes, & Eaves, 2002). A later, and larger, twin study, using parent ratings on the Conners Parent Rating Scale (CPRS), reflecting DSM-IV criteria for the subtypes, substantiated these findings with genetic correlations of 0.62 for boys and 0.57 for girls (McLoughlin, Ronald, Kuntsi, Asherson, & Plomin, 2007). The overlapping 95 % confidence intervals for the estimates of genetic correlation for two sexes suggests the proportion of genes shared between inattentiveness and hyperactivity–impulsivity is the same between the two sexes (see the section “Gender Differences” below for a further discussion).
One issue remaining with the above studies is the possibility of a “shared method variance”, where genetic correlations are artificially inflated by the use of ratings for both behaviours from a single rater. Studies that have used latent factor analysis to examine the aetiology of a “shared behavioural view” of the two symptom domains have generally reported the opposite effect; by removing rater-specific effects (which are reflected as error in multivariate twin models), the genetic correlation between the two symptom domains rises to 0.83 in a model which includes A, C and E influences (Wood, Rijsdijk, Asherson, & Kuntsi, 2010) or 0.74 when the nonsignificant C component is dropped from the same model, using the same rater measures, on the same sample (McLoughlin, Rijsdijk, Asherson, & Kuntsi, 2011). The overlapping 95 % confidence intervals for these two models suggest that the estimates are not significantly different, but instead highly similar.
The most robust finding across definitions of symptoms (DSM-III vs. DSM-IV), raters (parents vs. teachers vs. shared views) and ages (middle childhood vs. early adolescence) is that while approximately 50 % of the genes are shared between hyperactivity–impulsivity and inattentiveness, around 50 % of the genes are domain specific, suggesting that the two behavioural domains represent at least partially separable impairments. Thus, as the fifth version of the DSM (DSM-V) is finalised, it seems prudent, and likely, that the two symptom domains will remain conceptualised as distinct but correlated (American Psychiatric Association, 2012).
Gender Differences
The prevalence of ADHD is significantly increased in boys over girls (Polanczyk et al., 2007), raising the possibility that the aetiology differs by gender. Two types of gender-specific variance may be considered here: qualitative sex differences, where different genes and/or different environmental influences underlie ADHD in males and females, and quantitative sex differences, where the actual genetic and environmental influences are the same across genders, but they account for different standardised portions of the phenotypic variance. An investigation of a large population-based sample from the Twins Early Development Study (TEDS) followed up from early to middle childhood (at 2, 3, 4, 7 and 8 years of age) yielded no evidence of meaningful qualitative sex effects in the aetiology of ADHD (Kuntsi, Rijsdijk, Ronald, Asherson, & Plomin, 2005; McLoughlin et al., 2007). As concerns quantitative sex effects, with the exception of one study reporting sibling interaction effects for girls but not boys on parent-assessed hyperactivity–impulsivity using the multidimensional peer nomination inventory (MPNI) in 122 12-year-olds (Vierikko, Pulkkinen, Kaprio, & Rose, 2004), studies report the same significant variance components for males and females (Derks, Dolan, Hudziak, Neale, & Boomsma, 2007; Kuntsi et al., 2005; Larsson, Larsson, & Lichtenstein, 2004; Nadder et al., 2002; Rietveld, Hudziak, Bartels, van Beijsterveldt, & Boomsma, 2004; Rietveld, Posthuma, Dolan, & Boomsma, 2003; Ronald, Simonoff, Kuntsi, Asherson, & Plomin, 2008; Vierikko et al., 2004), although findings can differ across gender in the relative importance reported for each variance component. Some studies report either the same point estimates for males and females (within the same symptom domain and using the scale/informant (Kuntsi et al., 2005)) or a 10 % of less difference in variance components for girls and boys (Nadder et al., 2002; Rietveld, Posthuma et al., 2003; Ronald et al., 2008; Saudino, Ronald, & Plomin, 2005). Yet, others report greater differences of up to 25 % (Rietveld, Posthuma et al., 2003) or 32 % (Larsson et al., 2004). Even in our previous systematic review of methodologies (Wood, Buitelaar et al., 2010), it was not clear what may have lead some studies to find larger sex-specific differences than others. For example, the measure used to assess symptoms does not seem to have a systematic effect, as Rietveld et al. report differences of 25 % in heritability for attention problems assessed using mother reports from the child behaviour checklist (CBCL) at 7 years, but not on attention problems using the same scale and informant at 10 or 12 years of age, within the same sample (Rietveld, Hudziak, Bartels, van Beijsterveldt, & Boomsma, 2003). For now, it seems that the aetiology of ADHD in boys and girls is not different at a clinically meaningful level.
The Heritability of ADHD Symptoms Across the Lifespan
ADHD was initially believed to be a childhood disorder but is now recognised as frequently spanning from preschool age into adolescence and adulthood (Asherson & Gurling, 2012; Merwood & Asherson, 2011). Quantitative genetic research can make an important contribution to aetiological investigations of the stability of ADHD symptoms across the lifespan. A longitudinal analysis of a large population-based sample from TEDS suggested that preschool ADHD symptoms, as rated by parents, are moderately stable (r = 0.5) across the ages of 2, 3 and 4 (Price et al., 2005). Another study indicated that genetic influences were found to underlie 91 % of the stable variance in ADHD symptoms across the ages of 3–12 (Rietveld et al., 2004). We extended the longitudinal analysis of the TEDS sample into middle childhood by including children at the ages of 2, 3, 4, 7 and 8, replicating the findings of the previous study (Kuntsi et al., 2005). Thus, parent-rated ADHD symptoms were reported to be moderately stable over time and mainly due to shared genetic influences. Yet, the analyses indicated also additional genetic influences emerging in middle childhood that were not shared with those acting at an earlier age (Kuntsi et al., 2005). Extending the findings to early adolescence, a 5-year follow-up study of 1,480 Swedish twin pairs assessed at ages 8–9 and 13–14 reported stability in ADHD symptoms was estimated as 74 % genetically mediated (Larsson et al., 2004). Non-shared environmental factors only became important at ages 13–14. Subsequently, twin studies have shifted their focus from genetic and environmental influences on composite measures of ADHD symptoms to the genetic and environmental aetiology of the covariance between hyperactivity–impulsivity and inattention over time. In the Swedish study of 1,480 twin pairs, which focused on dimensional scale measures of hyperactivity–impulsivity and inattention derived from the DSM-IV ADHD checklist at the ages 8–9, 13–14 and 16–17, the symptoms of hyperactivity–impulsivity declined with increasing age, whereas no decline in inattention symptoms was observed (Larsson, Lichtenstein, & Larsson, 2006). Stable genetic influences acted mainly across subtypes and ages, although some age- and symptom dimension-specific effects were found. Recent analyses on the TEDS sample support these cross-sectional results with longitudinal findings suggesting that the proportion of the phenotypic correlation due to shared genetic factors remains similar in middle childhood (67 %) as in early adolescence (63 %; Greven, Asherson, Rijsdijk, & Plomin, 2011). The longitudinal models further suggested that hyperactivity–impulsivity in middle childhood predicts inattentiveness in adolescence, but that the relationship is unidirectional, with the reverse not being the case (Greven, Asherson et al., 2011). The mode of inheritance for the longitudinal association between the domains was complex: Only 30 % of the genes accounting for the covariance between hyperactivity–impulsivity and inattentiveness in middle childhood accounted for the covariation between the two behavioural domains in adolescence (Greven, Asherson et al., 2011).
ADHD and Co-occurring Disorders and Traits
An important contribution of quantitative genetic studies has been to understand the presentation of ADHD outside of the core symptom domains of inattentiveness and hyperactivity–impulsivity. A particular advantage of quantitative genetic studies over that of epidemiological approaches is that they allow us to look beyond rates of co-occurrence and examine aetiological reasons for any comorbidity. That is, twin studies allow us to understand the extent to which behaviours co-occur because of shared genetic influences, shared environmental contributors and/or rater biases. This can have potentially important clinical implications; clinicians can better understand the likely presentation of the ADHD patient as a whole and thus design treatment plans aimed at treating all behaviours that affect functioning, with more tailored treatments.
Oppositional Behaviour
Of all the disorders and problem behaviours that co-occur with ADHD, the symptoms of oppositional defiant disorder (ODD) and conduct disorder (CD) are the most common. At the phenotypic level, the co-occurrence is observed whether considering clinical samples, where 30–60 % of children diagnosed with ADHD obtain also a diagnosis of CD (Abramowitz, Kosson, & Seidenberg, 2004), or symptoms of ADHD and ODD or CD in the general population (see Jensen, Martin, & Cantwell, 1997 for a review).
Supporting the idea that familial influences between ADHD, ODD and CD are at least partially shared, a family study reported that relatives of each ADHD proband subgroup were found to be at significantly greater risk for ADHD and ODD than relatives of controls (Faraone et al., 2000). Rates of CD and antisocial personality disorder (ASPD) were elevated only among relatives of probands with ADHD and co-occurring CD, and the co-aggregation of ADHD and the antisocial disorders could not be accounted for by assortative mating (marriages between ADHD and antisocial spouses). In this study, both ADHD and antisocial disorders occurred in the same relatives more often than expected by chance alone (Faraone et al., 2000).
Subsequent twin studies have examined the extent to which ADHD behaviours and oppositional behaviour have shared unique genetic and environmental influences. In a twin study of individuals aged 5–17, all genetic influences on symptoms of CD were found to be shared with those on ADHD symptoms (Thapar, Harrington, & McGuffin, 2001). Yet, ADHD and CD symptoms were distinguished by an additional environmental factor influencing CD. A liability threshold model of the relationship between “pure ADHD” and co-occurring ADHD–CD predicted co-occurring ADHD–CD to lie on a continuum with ADHD, representing a graver subtype in terms of genetic loading as well as clinical severity (Thapar et al., 2001). Further evidence for a genetic overlap between ADHD and disruptive behaviour comes from two studies, which reported substantial genetic correlations between ADHD and ODD/CD symptoms in children aged 9–10 (r g = 0.43, Tuvblad, Zheng, Raine, & Baker, 2009) and adolescents between the ages of 8 and 16 (Nadder et al., 2002). As well as a genetic overlap, these two studies reported unique genetic and environmental factors specific to ADHD and specific to ODD/CD symptoms.
Studies that have separated the behavioural domains of ADHD have further substantiated these findings, reporting genetic correlations of between 0.64 and 0.82 between the three ADHD behaviours of hyperactivity, inattentiveness and impulsivity with DSM-IV-assessed symptoms of ODD/CD in probands between the ages of 8 and 16 (Nadder et al., 2002). Although it is possible that shared method variance contributed to many of these high correlations assessed “within rater”, we showed that the hyperactive–impulsive domain shared 94 % of its underlying genetic aetiology with oppositional behaviour, and the inattentive domain 56 %, by using a “shared behavioural view” of the three behaviours (inattentiveness, hyperactivity–impulsivity and oppositional behaviour) assessed by the CPRS (Wood et al., in press). The genetic overlap between hyperactivity–impulsivity and inattentiveness was smaller than that between oppositional problems and hyperactivity–impulsivity. Thus, there seems to be a specific link between hyperactivity–impulsivity and oppositional behaviour, which may represent a common underlying liability, suggesting that in middle childhood oppositional problems and hyperactivity–impulsivity share the same risk factors and cannot be separated on an aetiological basis (Wood et al., 2010; Wood et al., in press), whereas the inattentive domain is more distinct. ADHD may denote a risk factor for ODD/CD as the co-occurrence of the two phenotypes across time, from childhood into adolescents, is governed by a common set of genes, but ODD/CD is also influenced by additional genetic factors. More longitudinal studies will offer the opportunity to test this empirically.
Autism Spectrum Disorder
Clinical and population-based studies also suggest a high degree of overlap between ADHD, or symptoms of the disorder, and autism spectrum disorder (ASD) in children (Clark, Feehan, Tinline, & Vostanis, 1999; Goldstein & Schwebach, 2004; Ronald, Edelson, Asherson, & Saudino, 2010; Saudino et al., 2005; Sturm, Fernell, & Gillberg, 2007), which continues until adulthood, although the size of the correlation between traits may be smaller by then (Reiersen, Constantino, Grimmer, Martin, & Todd, 2008). A recent review concluded that 30–80 % of children with ASD meet ADHD criteria and that 20–50 % of children with ADHD meet ASD criteria (Rommelse, Franke, Geurts, Hartman, & Buitelaar, 2010). The first twin study to investigate the relationship between symptoms of ADHD and ASD reported a significant association between the CBCL attention problem scale and Social Responsiveness Scale (SRS) scores (Constantino et al., 2004). Although these bivariate analyses revealed that SRS scores are affected by phenotypic influences from the CBCL Social Problem syndrome, 44 % of the causal influences on SRS scores were independent from those on CBCL syndromes and genetic in nature. Since that first study, genetic overlap between ADHD and ASD has been shown in several samples. In 2-year-old twins, the genetic association was low to moderate (r g = 0.27), when controlling for socio-economic status and cognitive abilities (Ronald et al., 2010), but a greater degree of genetic overlap (r g = 0.57) has been reported in 8-year-old twins (Ronald et al., 2008). In a young adult twin sample aged between 18 and 33 years and drawn from the general population, a strong genetic correlation (r g = 0.56–0.86) was also observed between self-rated ASD and self-rated ADHD (Reiersen et al., 2008). The evidence for a genetic overlap between ADHD and ASD was further substantiated by a recent twin study, which estimated the probability of receiving a diagnosis of ADHD among MZ co-twins of children with ASD at 44 % (Lichtenstein, Carlstrom, Rastam, Gillberg, & Anckarsater, 2010). In addition, this study also reported that a substantial proportion of the genetic variance for ASD is shared with other neuropsychiatric disorders, such as developmental coordination disorder (DCD) and tic disorder.
Overall, twin studies suggest significant shared genetic influences on ADHD and ASD from childhood into adulthood. However, no longitudinal study has been carried out to investigate developmental patterns in more detail. The low genetic correlations between the three impairment domains of social interaction, communication, and thinking and behaviour within ASD (Ronald, Happé, & Plomin, 2005) further emphasise the need for the heterogeneity within the disorders to be addressed in further detail in the future.
Reading Difficulties
The co-occurrence between ADHD and reading disability, whether defined as diagnostic categories or quantitative traits, is well documented (August & Garfinkel, 1990; Dykman & Ackerman, 1991; Trzesniewski, Moffitt, Caspi, Taylor, & Maughan, 2006; Willcutt & Pennington, 2000a, 2000b). Levels of co-occurence between reading difficulties and ADHD have been estimated to range from 25 to 40 % (August & Garfinkel, 1990; Semrud-Clikeman et al., 1992; Willcutt & Pennington, 2000b). Recently, the aetiology of the relationship between ADHD and reading disability was investigated in 457 twin pairs (Willcutt et al., 2010). The analyses suggested that reading disability and ADHD share a common cognitive deficit in processing speed and that this deficit is primarily due to common genetic influences that increase susceptibility to both disorders. Independently, reading disability was also associated with deficits on measures of phoneme awareness, verbal reasoning and working memory, whereas ADHD was independently associated with a heritable weakness in inhibitory control. The co-occurrence between these two disorders also reflects a strong phenotypic association between reading disability and ADHD inattention symptoms, which has been largely attributed to shared genes (Martin, Levy, Pieka, & Hay, 2006; Paloyelis et al., 2010; Willcutt & Pennington, 2000a; Willcutt, Pennington, & DeFries, 2000; Willcutt, Pennington, Olson, & DeFries, 2007). A large combined twin sample from the USA, Australia and Scandinavia was assessed over the first three school years for reading using the Test of Word Reading Efficiency (TOWRE) and for inattentiveness and hyperactivity–impulsivity using the Disruptive Behaviour Rating Scale (Ebejer et al., 2010). The study interleaved mean reading and inattention scores into a single longitudinal analysis to examine how the two variables interact with each other across time. The results showed that the genetic overlap between inattention and reading occurs in a “generalist” factor that affects both variables across the three school years, that is, in place from the first school year, and that does not change with development. Genetic correlations between reading and inattention scores have also been found to be higher than genetic correlations between reading and hyperactivity–impulsivity score in 12-year-old twins (inattentiveness r g = 0.31, hyperactivity r g = 0.16; Greven, Harlaar, Dale, & Plomin, 2011) and a sample of twins aged 8–18 (inattentiveness r g = 0.46, hyperactivity r g = 0.24; Willcutt et al., 2010). We substantiated the proposition that the overlap between reading and ADHD is largely driven by shared genetic influences (45 %) and non-shared environmental factors (21 %), not shared with IQ and hyperactivity–impulsivity in population-based twin study. In our study, only 11 % of the covariance was due to genetic effects common with IQ (Paloyelis et al., 2010). We recently replicated these population-based findings in a clinical sample. An investigation of familial relationships between ADHD, reading difficulties and IQ in an ADHD and control sibling-pair sample of 6- to 19-year-olds revealed moderate familial correlations between ADHD, reading difficulties and IQ (r f = 0.3–0.5) and concluded that 53–74 % of the overlapping familial influences between ADHD and reading difficulties were not shared with IQ (Cheung et al., 2012). Although research in this field has started to incorporate the heterogeneity of ADHD, it has largely ignored the heterogeneity within reading disability itself. An exception is a recent study on the TEDS sample, which reported that word decoding rather than reading comprehension was differentially related to the ADHD dimensions, showing a lower genetic correlation to hyperactivity–impulsivity than to inattentiveness (Greven, Harlaar et al., 2011). Overall, the studies consistently suggest that the inattentiveness dimension of ADHD seems to drive most of the genetic overlap with reading.
The Broader Behavioural Profile Accompanying ADHD
Other co-occurrences have been less well studied and are an important avenue for further research in order to fully understand the likely presentation of ADHD when it reaches the clinic. The DSM-IV broadly divides childhood psychopathologies into those that are “externalising” and those considered “internalising”. ADHD falls into the former category, and a multivariate analyses of 1,162 twin pairs and 426 siblings found support for the separation of latent internalising and externalising behaviour factors, but a significant degree of aetiological overlap between the two, with 75 % of the genes and 74 % of the child-specific environmental factors shared (Cosgrove et al., 2011). Although there are also reports of substantial genetic overlap between DCD and ADHD, with the greatest overlap being between DCD and the hyperactive–impulsive domain of ADHD symptoms (Martin, Piek, & Hay, 2006), there is much scope for twin studies to continue to characterise, and explain at the aetiological level, the full presentation of ADHD in the clinic.
Currently, the strongest evidence supports that the overlap between ADHD and the co-occurring behaviours of oppositionality and ASD largely results from a shared genetic aetiology, with up to 100 % of the genes underlying pervasive oppositional behaviours and 60 % of those underlying ASD traits being shared with hyperactive–impulsive behaviours. However, these conclusions arise from data collected in middle childhood, and a more longitudinal examination of genetic overlap remains important. In addition, how much this reflects rater artefacts, such as halo effects where the teacher’s rating of ADHD behaviours are influenced by other traits manifest in the child (Abikoff, Courtney, Pelham, & Koplewicz, 1993; Jackson & King, 2004), warrants further research. If the shared genetic aetiology between ADHD behaviours and symptoms of other conditions is shown to be robust over raters, assessment methods and time, research needs to examine at what point in the pathways from genes to behavioural symptoms the features coalesce; a fruitful avenue of research to address this issue may be in developing biomarkers of these traits specific to each behavioural domain.
The Aetiological Architecture of Cognitive and Brain Processes in ADHD Endophenotypes: Definitions and Approaches
Quantitative genetic methods are immensely useful for the study of endophenotypes or intermediate phenotypes, as we hope to illustrate in this review of the relevant findings on ADHD. Endophenotypes refer to heritable, quantitative traits that are thought more directly to index underlying biology and risk for a disorder (Gottesman & Gould, 2003). In a recent conceptual analysis of the endophenotype concept, Kendler and Neale (2010) emphasise the distinction between liability index (or “risk indicator”) and mediation models, where only the latter makes the assumption that the causal pathway from genetic risk to disorder passes exclusively through the endophenotype. In this review we will use the term endophenotype, as in much of the research on ADHD conducted to date the mediational role of candidate endophenotypes has typically not been explicitly demonstrated, even if implicitly assumed. Yet, we also join Meyer-Lindenberg and Weinberger (2006) in emphasising how “genes do not encode for psychiatric phenomena (e.g. hallucinations and panic attacks)”. If an endophenotype is shown not to mediate the genetic risk on disorder (reflecting pleiotropic effects on endophenotype and disease risk), this raises the question of which as-yet-to-be-measured endophenotypes are the correct intermediate phenotypes instead.
Taking a historical perspective, Meyer-Lindenberg and Weinberger (2006) also note how endophenotypes were “initially envisaged to be tools for gene discovery… [yet] the greater power of intermediate phenotypes lies in using genetic risk variants as tools for the discovery of the mediating neural mechanisms that bridge the gap from DNA sequence to pathological behaviour”. It is this focus on understanding the underlying risk pathways and the genetic architecture of the cognitive and brain processes, where quantitative genetic methods can make significant, unique contributions, by, for example, addressing multivariate questions about the genetic interrelationships between different candidate endophenotypes. Endophenotype research has the potential of marrying the genetic literature on ADHD (reviewed above) with the vast literature on neurocognitive impairments in ADHD.
We further join Kendler and Neale (2010) in emphasising the need to consider the relative reliability of endophenotype and disorder measures when assessing the strength of the genetic signal and hence whether an endophenotype is closer to gene action than the disorder. We have illustrated the effects of varying levels of test–retest reliability across several cognitive measures used in ADHD research on the initial, simple question of univariate heritability of the measured cognitive processes (Kuntsi et al., 2006). Test–retest reliability for a measure sets an upper limit for the MZ twin correlation and, in turn, for detectable heritability. Only after correcting for the measured test–retest unreliability did heritabilities for cognitive measures approach those obtained for ADHD (Kuntsi et al., 2006), and aggregating measures increased heritability over single measures (Wood, Asherson et al., 2010; Wood et al., 2011). Overall, the findings illustrate the difficulty in directly comparing individual figures for measures that vary in their reliability.
Cognitive Endophenotypes
A summary of findings from quantitative genetic studies on cognitive endophenotypes in ADHD, published in 2005, concluded that there was evidence of association with genetic liability to ADHD for executive function performance, visual attention, processing speed and response variability (Doyle et al., 2005). The more recent studies indicate further evidence of shared familial/genetic effects with ADHD for various executive functions, such as working memory, inhibitory and attentional control (Bidwell, Willcutt, Defries, & Pennington, 2007; Gau & Shang, 2010; Goos, Crosbie, Payne, & Schachar, 2009; Loo et al., 2008; Slaats-Willemse, Swaab-Barneveld, De Sonneville, & Buitelaar, 2007; Uebel et al., 2010; Willcutt et al., 2010), and for reaction time variability (RTV) (Andreou et al., 2007; Bidwell et al., 2007; Uebel et al., 2010), visual memory (Shang & Gau, 2011) and aspects of temporal information processing. Collectively, these studies have been important in establishing aspects of cognitive performance that share familial/genetic risk factors with ADHD.
Most recently, the field has started to move beyond the bivariate question of shared genetic risk factors between ADHD and a specific cognitive process towards multivariate questions about the overall genetic architecture underlying the multiple cognitive impairments in ADHD. In a large-scale investigation of ADHD and control sibling pairs (total n = 1,265 participants), we addressed the question of whether one or more familial factors underlie the slow and variable reaction times, impaired response inhibition and choice impulsivity associated with ADHD (Kuntsi et al., 2010). Two familial cognitive impairment factors emerged. The larger familial factor, accounting for 85 % of the familial variance of ADHD, captured 98–100 % of the familial influences on mean reaction time (RT) and RTV. This factor separated from a second familial factor that captured 62–82 % of the familial influences on omission and commission errors (CE) on a go/no-go task and accounted for 13 % of the familial variance of ADHD. Drawing on the arousal attention (Johnson et al., 2007; O’Connell et al., 2008) and developmental models of ADHD, we proposed that the first factor (RT) may represent bottom-up arousal dysregulation and the second factor (errors) top-down control of sustained attention and inhibition (Carr, Henderson, & Nigg, 2010). Most recently we have shown, with a large population twin sample, that the RTV–ADHD association reflects largely on genetic influences that RTV shares with inattention, whereas the CE–ADHD association reflects on influences that CE shares more evenly with both inattention and hyperactivity–impulsivity (although the associations with CE were lower overall (Kuntsi et al., 2013)). There were no significant shared genetic influences across RTV and CE, consistent with the aetiological separation between the two indices of cognitive impairments.
ADHD is also associated with lower IQ, which twin analyses indicate is largely due to shared genes (Kuntsi et al., 2004). Giving further insight into the overall genetic architecture of the impairments, our further multivariate model fitting analyses both on the ADHD and control sibling sample (Wood et al., 2011) and on population twin data (Wood et al., 2010) show that the aetiological influences that ADHD shares with those on IQ largely separate from the aetiological influences on ADHD and the other cognitive impairments, consistent also with a separate ADHD sibling study using different cognitive measures (Rommelse et al., 2008).
EEG and MRI Endophenotypes
To measure the brain processes directly, brain imaging methods—such as electrophysiology or magnetic resonance imaging (MRI)—are required. Electroencephalography (EEG), and the derived application event-related potentials (ERP), enable a direct, non-invasive measurement of neural activations associated with a wide variety of brain states and processes, with excellent time resolution (Banaschewski & Brandeis, 2007). The cost-effectiveness of EEG measurement, the high heritability of EEG power and the opportunity to characterise the interplay of neural states and information processing further support the use of EEG for the study of endophenotypes in ADHD (Banaschewski & Brandeis, 2007; Tye, McLoughlin, Kuntsi, & Asherson, 2011). A recent systematic review of genetically informative EEG/ERP studies of ADHD concluded that there was emerging evidence for familial/genetic overlap with ADHD for EEG power (theta/beta) and alpha asymmetry and for specific ERP indices measuring attentional processing, inhibition and performance monitoring (Tye, McLoughlin et al., 2011). In addition, a recent twin study found substantial genetic overlap between ADHD and very-low frequency EEG activity (Tye, Rijsdijk et al., 2011). Findings also suggest that key ERP impairments are found in ADHD throughout development: Using identical measures, familial influences shared with ADHD were obtained for impaired attentional orienting (attenuated cue-P3) and performance monitoring (attenuated Ne and N2) in both child (Albrecht et al., 2008, 2010) and adult samples (McLoughlin, Asherson et al., 2011; McLoughlin et al., 2009).
MRI methods offer superior spatial resolution, but their main disadvantage for large-scale genetic studies is their high cost. Sibling studies suggest sensitivity to familial risk for ADHD for MRI measures that include volumetric reductions in cortical grey and white matter (Durston et al., 2004), activation in the ventral prefrontal cortex (Durston, Mulder, Casey, Ziermans, & Van Engeland, 2006) and functional connectivity between cognitive control regions (Mulder, Van Belle, Van Engeland, & Durston, 2011). Activation in the cerebellum was also sensitive to familial vulnerability to ADHD in one study (Mulder et al., 2008), whereas an earlier study found evidence of decreases in the cerebellar volume only in siblings with ADHD but not among unaffected siblings (Durston et al., 2004).
Overall, quantitative genetic research on ADHD using EEG and MRI approaches is at an early stage, with only a limited number of studies conducted to date and several of the studies incorporating relatively modest sample sizes. Larger samples will enable the application of more advanced model fitting to test more complex hypotheses.
A Summary of Molecular Genetic Studies on ADHD
The high heritability, as established by the quantitative genetic studies, has made ADHD a strong candidate for molecular genetic investigations. We provide a brief summary of key molecular genetic findings on ADHD; a detailed review is beyond the scope of this chapter.
Candidate Gene Studies
Candidate gene studies, where genes are a priori selected for an examination of associations with the ADHD phenotype based on inferred biological pathways from genes to behaviour or previous suggested associations, have provided some of the strongest evidence for molecular genetic associations with ADHD. The earliest reported associations were between ADHD and the dopamine D4 receptor (DRD4) and the dopamine transporter (DAT1) genes (Cook et al., 1995; LaHoste et al., 1996; Swanson et al., 1998), with a subsequent report of an association with the functionally associated dopamine D5 receptor (DRD5) gene (Lowe et al., 2004). The replication of associations with the seven-repeat allele of a Variable Number Tandem Repeat in DRD4 and a microsatellite upstream of DRD5 are of note as they are the only genes to reach significance in the first comprehensive meta-analysis and were associated with low p-values in the region of 5 × 10−8, suggesting confidence in the findings (Li, Sham, Owen, & He, 2006). Associations with DAT1 have been less replicated with both positive and null associations emerging, such that DAT1 did not show a significant association with ADHD in an initial meta-analysis (Li et al., 2006). However, this may have been due to heterogeneity across datasets as DAT1 did show a significant association with ADHD in the most recent published meta-analysis (Gizer, Ficks, & Waldman, 2009). Given that the current focus for molecular genetic associations is on replication, outside of these initial findings, we here focus on the efforts of meta-analytic approaches on candidate gene studies. Currently, the genes that are significantly associated with ADHD in the meta-analysis of candidate gene approaches remain as DAT1, DRD4 and DRD5 and include the serotonin transporter (5HTT), serotonin 1B receptor (HTR1B) and the synaptosomal-associated protein 25 isoform (SNAP25) genes (Gizer et al., 2009). Although it should be noted that effect sizes are small, with odds ratios ranging from 1.12 to 1.33 in a recent meta-analysis (Gizer et al., 2009), it is clear that these most promising genes are functionally linked through their roles in dopamine and serotonin metabolism, paving the way for promising work in the immediate future using gene enrichment, or pathway analysis, the bioinformatics tools necessary for which are just becoming more widely available.
Genome-Wide Approaches and CNVs
While enjoying a modest success in gene–phenotype associations, candidate gene studies to date can only account for a very small percentage of the genetic variance estimated to underlie ADHD. This is consistent with the “many genes of small effect” hypothesis suggesting that there are many gene regions that associate with ADHD that have yet to be examined. Here genome-wide approaches are promising. Hence, genome-wide approaches, which are by nature “hypothesis-free” or “hypothesis generating”, have generated interest. Two complementary approaches, linkage and association, examine the whole genome for associations with traits. Given how little we know about the psychological processes and biological origins underlying the behavioural symptoms of ADHD, and the small effect sizes associated with behavioural measures and candidate genes, it seems prudent to pursue efforts that systematically screen the genome for susceptibility variants. It is hoped that functional pathways from genes to ADHD can then be inferred and putative endophenotypes located along those pathways.
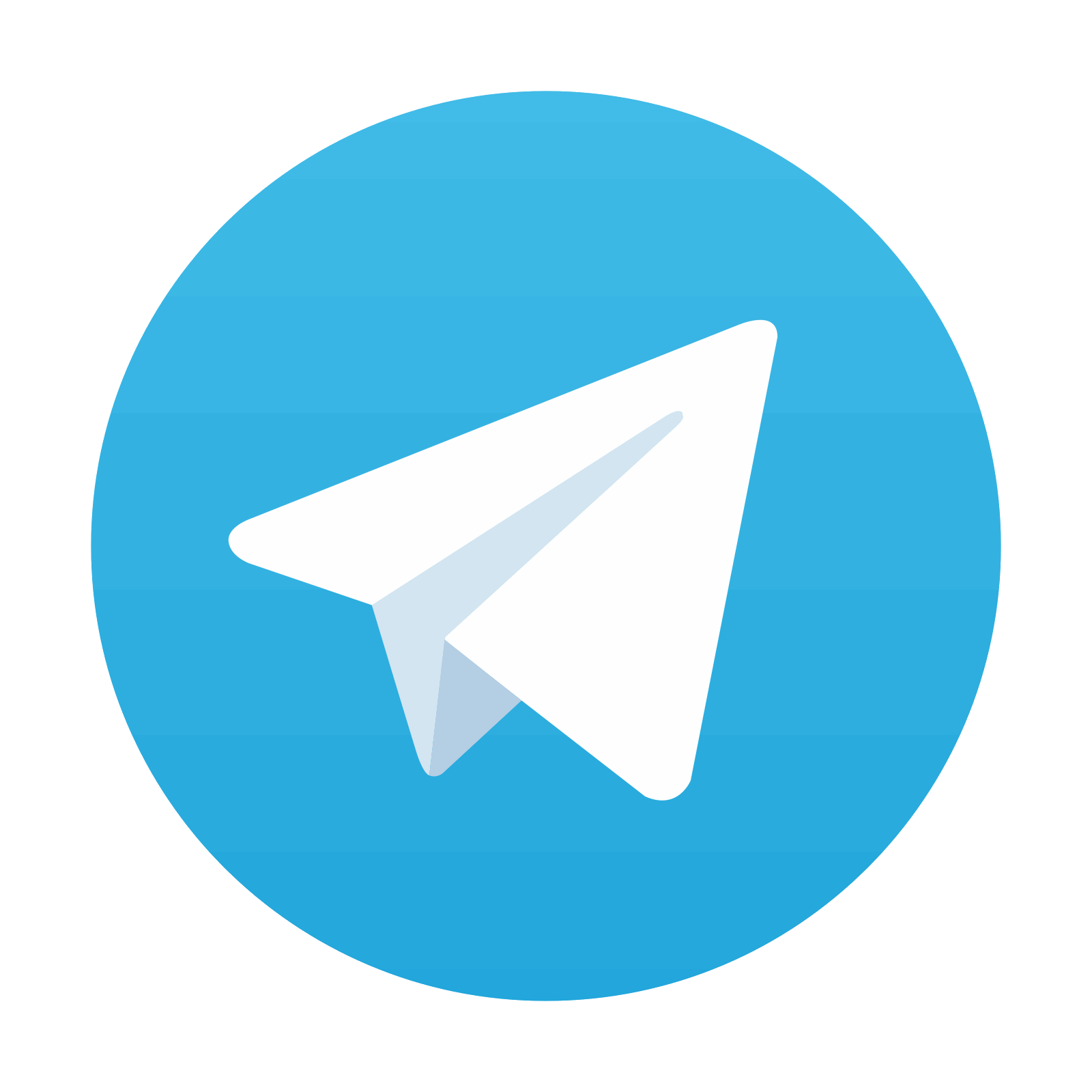
Stay updated, free articles. Join our Telegram channel

Full access? Get Clinical Tree
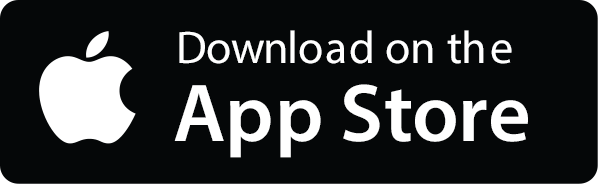
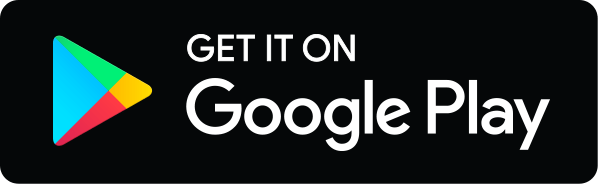