Fig. 5.1.
Main circuits of the basal ganglia (motor and nonmotor loops). The basal ganglia (bold characters) include: (1) the corpus striatum divided into neostriatum (putamen, caudate nucleus, and nucleus accumbens as ventral striatum), globus pallidum or paleostriatum consisting of the medial segment (GPi), the lateral segment (GPe), and the ventral pallidum (VP), (2) substantia nigra, (3) ventral tegmental area (VTA) and (4) subthalamic nucleus (STn). To dissociate excitatory (glutamate) from inhibitory (GABA) projections, the former are represented with bold arrows. The main input structure of the basal ganglia is the striatum, receiving excitatory projections from many cortical areas: the putamen is involved in the motor loop, the caudate in the associative loop, and the nucleus accumbens in the limbic loop. The main outputs are GABAergic neurons in medial globus pallidus and substantia nigra pars reticulata. There are two main pathways, direct and indirect (passing through the GPe and STn) which connect the striatum with these output nuclei of the basal ganglia. While neurons giving rise to these pathways are morphologically similar and receive similar inputs from cerebral cortex, they are connectionally and neurochemically distinct. In particular, D1 and D2 dopamine receptors (D1r and D2r) are segregated respectively to direct and indirect striatal pathway neurons. These outputs are directed to thalamic nuclei providing feedback to frontal cortical, to lateral habenula providing projections to the limbic system, as well as to two brainstem regions: superior colliculus and pedunculopontine nucleus involved in attention, locomotion, and eye movements. The substantia nigra pars compacta provides dopaminergic fibers (DA) to the dorsal striatum while the VTA provides DA fibers to the ventral striatum. The thalamus provides cholinergic fibers to the straitum
1.2 Parkinson’s Disease
Parkinson’s disease is mainly an age-related sporadic disorder, but an early-onset version is known to be transmitted in an autosomal dominant or recessive manner (6). Parkinsonian symptoms have been described following methyl-phenyl-tetrahydropyridine (MPTP) intoxication (7).
Whatever the etiology, primary symptoms are caused by the degeneration of neurons in SNc and the ventral tegmental area (VTA) (5, 8), resulting in affected ascending dopaminergic tracts and, consequently, decreased dopamine concentrations in striatum, nucleus accumbens, and prefrontal cortex. Dopamine loss in the putamen is mostly responsible for motor deficits, including akinesia (difficulty in initiating movement), bradykinesia (slowing of movements), muscle rigidity, tremor at rest, and micrographia (smaller handwriting). Major neuropsychiatric signs include apathy with or without depression (9, 10).
Dopamine loss in caudate is responsible for cognitive disturbances, together with and degeneration of ascending noradrenergic, serotoninergic, and cholinergic tracts (11). The patients have a dysexecutive syndrome, defined as deficient planning of organized sequence of events and concept shifting, as demonstrated in the following tests: odd-man-out (12, 13), poisoned food (14), trail-making (15–17), covered maze (18), and Wisconsin card sorting (13, 16, 17, 19–25). Memory impairment is another prominent feature, revealed in memory quotient (MQ) subtests such as digit span (13, 15) and story recall (13, 15, 22, 26, 27) as well as spatial memory in the Rey complex figure (13, 15, 27) and paired-associate verbal learning (13, 15), though the latter is not always affected (26, 27). A dissociation between impaired recall and normal recognition was demonstrated for verbal (24) and visuospatial (23) material. Spatial dysfunctions are observable in copies of the Rey complex figure (27), block design (15), route-walking (28), and body orientation (29). Procedural learning is deficient in the following tests: pursuit rotor (30), inverted reading (31), prism adaptation (32), finger sequences (33, 34), hand-tracing (35), Tower of Hanoi (36, 37), and Tower of London (38, 39).
1.3 Huntington’s Disease
Huntington’s disease is characterized by degenerating neurons in the caudate and the putamen, particularly medium spiny neurons containing GABA, whereas larger cholinergic neurons tend to be relatively spared (40, 41). To a lesser degree, there is degeneration in the frontal cortex, substantia nigra, thalamus, subthalamic nuclei, and cerebellum. Motor dysfunctions in patients with Huntington’s disease include chorea, dystonia, tremor, gait disturbances, and rigidity (42, 43). The patients are marked at an early age by hyperactivity followed by hypoactivity and apathy.
Anterograde amnesia is one of the earliest and most severe cognitive symptoms seen in this dementing disease (44). MQ subtests are affected, including digit span, story and figure recall, and paired-associate verbal learning (45). Procedural learning is impaired in pursuit rotor (46), mirror reading (47), Tower of Hanoi (44), and serial reaction time (48) tests. In addition, well-learned motor habits are affected, as in automobile simulations (49). Affected IQ subtests include block design and object assembly (spatial functions), digit symbol (sensorimotor speed), and picture arrangement (abstract thinking and judgment) (50, 51).
1.4 Focal Lesions
In patients with caudate lesions, common symptoms include abulia and behavioral disinhibition, as defined by overactivity of thought, speech, or movement (52). In patients with lesions of the putamen and pallidum nuclei, common motor symptoms include dystonia and parkinsonism, together with apathy and obsessive-compulsion (53). Focal caudate lesions impaired recall but not recognition of verbal material, copy and recall of the Rey complex figure, Wisconsin card sorting, and Porteus maze tests (54). A single case study of bilateral globus pallidus lesions with frontal lobe hypometabolism revealed impaired spatial functions and procedural learning in the Tower of London (55).
2 Experimentally Induced Lesions of Basal Ganglia
We describe experimentally induced lesions of basal ganglia to specify tests liable to be affected in genetically modified animals with basal ganglia disorders. Unless otherwise specified, all experiments cited in this section were conducted in rats of different strains.
2.1 Neurologic Signs
A compulsory approaching syndrome has been described in cats with bilateral electrolytic caudate lesions (56), characterized by stereotyped and persistent following and attempts at physical contact with other cats, persons, and objects, sometimes by grasping or jumping on them. Two pathological reflexes were also noted: rooting (continued contact with any object touching the snout) and limb placing (continued paw contact on a horizontal surface). Hindlimb clasping has been described after striatal and SNc lesions caused by systemic 3-nitropropionic acid (3-NP), an effect potentiated by systemic MPTP (57).
2.2 Sensorimotor Integration
The injection of 6-hydroxydopamine (6-OHDA), destroying dopamine terminal fibers in the lateral neostriatum, decreased sensitivity to von Frey hair stimulation, implying a motor response to tactile stimulation (58). 6-OHDA-induced lesions in the medial neostriatum had no effect.
2.3 Motor Activity
Quinolate- and 3-NP-induced striatal lesions increased motor activity in the open-field test (59). However, quinolate-induced reduction of time spent in moving has also been reported, presumably owing to differences in lesion severity or in the postsurgery period (60). Motor activity is more closely related to the mesolimbic system, as hypoactivity occurs after 6-OHDA injections into ventral tegmental area or nucleus accumbens (61). Hypoactivity is a prominent feature of systemic MPTP in rats (62) and mice (63), but intranigral MPTP caused hyperactivity, presumably because of a compensatory response of the remaining neurons, whereas 6-OHDA had no effect (64).
2.4 Motor Coordination
Deficits in the rotorod test (based on locomotion on a rotating bar) were observed after systemic MPTP in rats (62) and mice (65). Systemic MPTP also slowed crossing of a stationary beam in mice (66). Unilateral 6-OHDA injections in medial forebrain bundle are sufficient to cause rotorod deficits, defined by latencies before falling (67) and abnormal stepping movements (68), as well as slowing stationary beam walking (69).
2.5 Conditioned Reflexes
2.6 Bar-Pressing
2.7 Spatial Learning: Morris Water Maze
One of the most commonly used tests to assess spatial learning in rodents is the Morris water maze (75–77). When the platform is submerged, the task can be solved with extra-maze cues (place learning). A probe trial is given with the platform removed, to determine to what extent the animal learned its specific location. When the platform is visible, the task can be solved by local cues (visuomotor guidance).
Electrolytic neostriatal lesions impaired submerged and visible platform subtasks (78). Submerged platform and probe tests were impaired by quinolinate-induced neostriatal lesions sparing fibers of passage (79). Submerged and visible platform tests were deficient after electrolytic lesions in dorsomedial but not dorsolateral striatum (80). However, smaller electrolytic lesions in dorsomedial striatum did not affect either task (81), whereas visible platform performance was deficient after dorsolateral lesions caused by the neurotoxic substance ibotenate (82).
The impact of dopamine is illustrated by findings of impaired place learning after 6-OHDA lesions in SNc combined with desipramine-pretreatment to prevent noradrenaline depletion (83). Likewise, submerged platform and probe tests were impaired with desmethylimipramine pretreatment and 6-OHDA injections into subiculum and CA1 subfield of hippocampal formation, though the response of swimming toward the visible goal was not impaired (84). In contrast, visuomotor guidance was deficient after 6-OHDA injections into SNc, together with a working memory version with different platform locations (64). After a noradrenaline uptake blocker, intraventricular (85, 86) and intracisternal (87) 6-OHDA injections in the neonatal period impaired place learning in the juvenile-adult stage.
MPTP administered intranasally (88) or into the SNc (64, 89) impaired water maze working memory. Moreover, MPTP injections in SNc impaired a version with a constant starting place (90). On the contrary, intranasal (88) or intra-SNc (90–92) MPTP had no effect in the standard version with variable starting places and a constant platform location. However, visuomotor guidance was deficient after MPTP administered through intranasal (88) or intra-SNc (91–93) routes. Thus, 6-OHDA and MPTP cause deficits in the visible platform task, but only 6-OHDA seems to affect place learning, attributable to differences in lesion severity or in behavioral procedures.
Chronic peripheral injection of the D2 receptor antagonist, haloperidol, impaired submerged platform and probe tests, but performance in these tests was not impaired by similar administration of the atypical antipsychotics risperidone and clozapine (94). The D2 receptor antagonist sulpiride, injected in posteroventral striatum between training and probe trial, disturbed retention (95).
In addition to the nigrostriatal system, the mesolimbic system appears to be involved. Place learning was slowed after ibotenate-induced lesions of nucleus accumbens (96), but not after electrolytic (97) or lidocaine-induced (98) lesions restricted to its medial (shell) part. The injection of haloperidol in the nucleus accumbens impaired place learning without affecting visuomotor guidance (99). The injection of sulpiride into the nucleus accumbens between training and probe trial disturbed retention (100). The injection of NMDA and AMPA receptor antagonists into the nucleus accumbens impaired the probe trial without affecting acquisition (101).
2.8 Spatial Learning: Left-Right Discrimination and Alternation
The basal ganglia exert an influence on left-right discrimination learning, in which rats find food with only one arm. The acquisition and reversal of left-right discrimination was impaired by electrolytic (102, 103) or kainate-induced (104) caudate lesions. Deficits in spatial reversals were further documented after electrolytic lesions in anterior striatum (105) and caudate (106). However, Schwartzbaum and Donovick (107) found no effect on acquisition and reversal of this task after electrolytic caudate lesions. Pisa and Cyr (108) found impaired reversal but not acquisition, following ibotenate-induced medial striatal lesions, whereas lateral striatal lesions had no effect. Left-right discrimination learning was impaired after ibotenate-induced lesions of the nucleus accumbens (96). When a water tank was used, quinolinate-induced dorsal striatal lesions impaired left-right discrimination and reversal learning (79). Likewise, left-right reversal learning was deficient after 6-OHDA lesions in dorsal striatum (109).
The acquisition of left-right alternations was slowed after electrolytic anterior neostriatal lesions (105). The performance of learned alternation was likewise impaired by kainate-induced anteromedial neostriatal lesions (110). However, Colombo et al. (111) found no effect on learned alternation performance after electrolytic caudate lesions. The acquisition and performance of alternating bar-presses were impaired after electrolytic caudate lesions (112), showing similarity to performance deficits after electrolytic anteromedial neostriatal lesions (113).
2.9 Spatial Learning: Other Mazes
The acquisition of place learning in a radial arm water maze was deficient after quinolate-induced striatal lesions (59). The acquisition of egocentric place learning in the radial arm maze for food reward was deficient after electrolytic caudate lesions (114). The same lesions impaired learning to find food pellets in adjacent arms of this maze (111, 115). Moreover, kainate-induced lesions in the dorsomedial and dorsolateral striatum impaired acquisition of a 14-unit T-maze (116). However, no effect on the Hebb-Williams maze was found after electrolytic caudate lesions (112). Spatial delayed response, a task rarely used in rodents, was impaired in monkeys after systemic MPTP (117),
2.10 Nonspatial Discrimination Learning
In monkeys, visual discrimination learning between objects was impaired after ventral putamen lesions (118). Concurrent visual discrimination learning was likewise impaired after ibotenate-induced lesions affecting the tail of the caudate nucleus and the ventrocaudal putamen (119). Various results have been found in rodents. Electrolytic caudate lesions slowed acquisition of light-dark discrimination learning for food reward (107), though Mikulas (120) found that such lesions impaired this task only when the light stimulus was used as reinforcement, concordant with an exaggerated photophobic response. Reversal learning of light-dark discrimination was impaired after electrolytic caudate lesions in cats (73). Likewise, a tactile discrimination task was impaired after electrolytic caudate lesions (111). However, there was no effect on visual pattern discrimination after kainate-induced anteromedial neostriatal lesions (110). Quinolinate-induced lesions of dorsolateral striatum impaired acquisition (121) and performance (122) of a compound stimulus discrimination learning task requiring bar-pressing. In contrast, dopamine does not seem to be involved in appetitive discrimination learning, as systemic pimozide (123) and chlorpromazine (124) had no effect on light-dark or pattern discrimination learning, respectively.
In a water escape paradigm, pattern discrimination learning was impaired after electrolytic caudate lesions (125). Neither medial nor lateral neostriatal lesions slowed acquisition of brightness discrimination in active avoidance of electric shock (126). In contrast, performance of this task was deficient after instrastriatal injections of the D2 receptor blocker raclopride but not the D1 receptor blocker SCH23390 (127).
3 Genetically Modified Mice for Parkinson’s Disease
Several Parkinson-related genes have been cloned, such as SNCA, PARK2, UCHL1, and DJ1 (6), from which murine genetic models have been developed (129–131). SNCA transgenic mice (Table 5.1) overexpress α-synuclein, a major component of Lewy bodies, which also contain parkin, ubiquitin, and UCHL1. Although the initial report was negative (132), mice with the SNCA A53T transgene driven by the murine Prp promoter had decreased dopamine transporter (DAT) binding in the dorsal striatum and nucleus accumbens (133). However, other mutated SNCA models have no apparent nigro-striatal pathology. Mice with the SNCA A30P transgene driven by the murine Prp promoter had normal tyrosine hydroxylase (TH) staining in SNc and striatum (134). Mice with the SNCA A30P transgene driven by the hamster Prp promoter had normal TH-immunoreactive cell numbers in SNc and normal striatal TH-immunoreactivity and dopamine concentrations (135). Likewise, mice expressing SNCA A30P driven by murine Thy1 (136) had unchanged dopamine concentrations in the striatum and frontal cortex (137). Because of chosen promoters, α-synuclein accumulation is widespread in these models, as well as SNCA A53T transgenes driven by murine Prp (138) and Thy1 (139) promoters.
Table 5.1
Dopaminergic pathologies with motor activity and coordination in mice overexpressing mutated or wild-type SNCA
Transgene manipulation and promoter | Dopamine phenotype | Motor activity and coordination | References |
---|---|---|---|
A30P mutation Murine Prp | None | ↓ Rotorod | Yavich et al. (134). |
A30P mutation Murine Prp | ? | None | Lee et al. (132). |
A30P mutation Hamster Prp | None | ↓ Rotorod | Gomez-Isla et al. (135). |
A30P mutation Murine Thy1 | None | ? | Neumann et al. (137). |
A53T mutation Murine Prp | ? | ↓ Rotorod | Gispert et al. (138). |
A53T mutation Murine Prp | ↓ DAT binding in dorsal striatum | None | Lee et al. (132). Unger et al. (133). |
A53T mutation Murine Prp | ? | ↓ Open-field activity | George et al. (163). Giasson et al. (160). |
A53T mutation Murine Thy1 | ? | ↓ Rotorod | van der Putten et al. (139). |
Y39C mutation Murine Th | ↓ TH+ SNc neurons and striatal terminals | ? | Zhou et al (141). |
Synthetic deletion mutation Rat Th | ↓ Dopamine concentrations | ↓ Open-field activity | Tofaris et al. (142). |
Synthetic deletion mutation Rat Th | ↓ SNc neurons | ↓ Open-field activity | Wakamatsu et al. (143). |
G88C+G209A mutation Rat Th | ↓ Dopamine concentrations | ↓ Inverted grid | Richfield et al. (140). |
Wild-type Rat Th | None | None | Richfield et al. (140). |
Wild-type Murine Thy1 | None | ↓ Stationary beam | Fleming et al. (166). Rockenstein et al. (146). |
Wild-type Human PDGFB | ↓ TH+ terminals in striatum | ↓ Rotorod | Masliah et al. (144). |
Wild-type Murine Mbp | ? | ↓ Rotorod | Shults et al. (145). |
Conditional wild-type SNCA Murine Cam | None | ↓ Rotorod | Nuber et al. (147). |
Models with artificial (nondisease-related) mutations have been generated with the more dopamine-specific Th promoter (Table 5.1). Aged mice with the SNCA G88C+G209A transgene driven by the rat Th promoter had reduced dopamine concentrations, though at a younger age DAT binding was enhanced (140). In contrast, mice with the SNCA Y39C transgene driven by the murine Th promoter had normal TH+ SNc neuronal numbers and striatal terminals (141). Expression of truncated α-synuclein seems to cause an important impact. An artificial SNCA 120 mutant expressing human truncated α-synuclein without the final 20 amino acids under control of the rat Th promoter had reduced striatal dopamine concentrations (142). Moreover, deletion SNCA mice with the A53T mutation yielding truncated human α-synuclein under control of the rat Th promoter had reduced SNc dopamine neuronal numbers (143).
Nonmutated SNCA transgenic mice are available (Table 5.1). Mice expressing the wild-type SNCA transgene driven by the human PDGFB promoter had fewer striatal dopaminergic terminals (144). Mice expressing wild-type SNCA driven by the murine Mbp promoter had lower TH-immunoreactivity in SNc and striatum (145). Mice with wild-type SNCA driven by the rat Th promoter had normal dopamine concentrations and DAT binding (140). Likewise, mice expressing wild-type SNCA driven by murine Thy1 have no apparent nigral pathology (146). Moreover, conditional wild-type SNCA under control of the murine Cam (calcium/calmodulin-dependent protein kinase IIα) promoter had no significant reductions in nigral TH+ dopamine neurons or striatal dopamine concentrations and DAT binding (147).
In view of disease-related loss-of-function Park2 mutations (6), six parkin-deficient models have been developed (Table 5.2) with different exon deletions (148–152). One Park2 null mutant had downregulated striatal DAT levels but normal TH-immunoreactivity in SNc and striatum as well as normal dopamine concentrations in the brainstem and striatum (149). The same negative result regarding nigral and striatal TH-immunoreactivity applies to a second model (153), as well as nigral TH-immunoreactivity in a third model, together with normal nigral TH+ neuronal numbers, striatal dopamine concentrations, and striatal D1 and D2 receptor binding (148).
Table 5.2
Dopaminergic pathologies with motor activity and coordination in Park2 null mutants
Exon deletion | Dopamine phenotype | Motor activity and coordination | References |
---|---|---|---|
2 | ↓ DAT | None | Perez and Palmiter (150) |
3 | None | ↓ Open-field activity | Zhu et al. (153). |
3 | ↑ Extracellular dopamine | ↓ Stationary beam | Goldberg et al. (148). |
3 | ↓ DAT | ↓ Open-field activity | Itier et al. (149). |
3 | ? | ? | Stichel et al. (151). |
7 | None | None | von Coelln et al. (152). |
Mice with the UCHL1 I93M transgene driven by the human PDGFB promoter had reduced nigral TH+ neurons and striatal TH+ fiber terminals (154). Although not related to Parkinson mutations, Pitx3 is a transcription factor selectively expressed in midbrain and the lens (155). Pitx3-deficient aphakia (ak) mice had reduced dopamine concentrations in the striatum as a result of SNc degeneration (155–158) and reduced stimulation-induced dopamine outflow in the striatum (159). But, unlike affected humans, Pitx3/ak mutant mice are blind.
3.1 Neurologic Signs
Because of widespread α-synuclein accumulation, SNCA A30P/haPrp mice are susceptible to paralysis, which is not a part of the Parkinsonian symptomatology (135). The same applies to the SNCA A30P/raThy1 (137) model and two SNCA A53T/muPrp models (132, 160). To our knowledge, hindlimb clasping has not been described in Parkinson models, although this sign was observed after systemic 3-NP and potentiated by systemic MPTP (57).
3.2 Motor Activity
As expected, SNCA A30P/muThy1 mice had lower vertical activity in the open-field than nontransgenic controls (161). However, their horizontal activity was paradoxically higher. SNCA A53T/raTh transgenic mice with truncated α-synuclein had reduced horizontal activity relative to controls (143), an effect reversed by l-dopa and D2 receptor agonists (162). Likewise, horizontal activity diminished in SNCA A53T/muPrp ( 163) and SNCA 120/raThy1 (142) models. On the contrary, SNCA Y39C /muTh mice had normal activity levels (141).
Two exon 3 Park2 null mutant models were hypoactive in the open-field (149, 153), but this was not the case with a third (148), or those with exon 2 (150) or 7 (152) deletions. These behavioral results are not surprising in view of the fact that none of these models has been shown to have reduced dopamine concentrations. In the only model of its kind tested to date, home-cage activity was reduced in aged UCHL1 I93M transgenic mice, while younger mice were characterized by higher activity (154).
3.3 Motor Coordination
As expected, several SNCA transgenic models have deficiencies in motor coordination (164). SNCA transgenic mice with A30P (134, 135, 161) and A53T (138, 139) mutations were deficient relative to nontransgenic controls on the rotorod, though the SNCA A30P /haPrp model was tested with the unusual procedure of reversing beam rotation (135). Likewise, rotorod deficits were reported in SNCA Y39C /muTh ( 141) and wild-type SNCA/muMbp ( 145) transgenic mice. Relative to wild-type and mutant mice with retinal degeneration, Pitx3/ak mice were deficient on the rotorod (165). On the contrary, SNCA A30P/muPrp and SNCA A53T/muPrp mice had normal rotorod scores (132), as did Park2 knockouts (148, 152, 153) and UCHL1 I93M transgenic mice (154).
Wild-type SNCA/muThy1 had more slips on a stationary beam (166). An exon 3 Park2 null mutant also had more slips on the stationary beam (148), but this was not the case with the exon 2 deletion (150). Park2 null mutants had normal suspended string (149) or bar (150) performance, attesting to normal muscle strength.
3.4 Spatial Learning
SNCA A30P/muThy1 mice had normal acquisition but poorer probe trials in the Morris task (161). The same pattern was displayed by conditional wild-type SNCA transgenic mice (147). An exon 3 deletion Park2 knockout model had deficient acquisition in the Morris water maze (153), but this was not the case with the exon 2 deletion (150). Relative to wild-type controls and mutant mice with retinal degeneration, Pitx3/ak mice were deficient in left-right discrimination in a water-filled T-maze (165).
3.5 Nonspatial Discrimination Learning
Light-dark discrimination learning was normal in Park2 null mutants (150).
3.6 Avoidance Learning
SNCA A30P/muThy1 mice had poorer active avoidance learning (161). Relative to wild-type controls and mutant mice with retinal degeneration, Pitx3/ak mice were deficient in passive avoidance learning (165). In contrast, passive avoidance retention was paradoxically improved in Park2 null mutants (150).
4 Genetically Modified Mice for Huntington’s Disease
Transgenic and knock-in models expressing mutant human HD are valuable models of Huntington’s disease (167). Two of the best characterized are R6/1 and R6/2 transgenic mice, expressing mutant exon 1 of the HD gene with CAG repeats, causing polyglutamatine amplification, larger in the latter (168). Other transgenic models include mice expressing HD with 16, 48, and 89 CAG repeats (169), the YAC128 mouse containing a yeast artificial chromosome with full-length human mutated HD (170, 171), and the HD-like rat producing truncated chimeric human/rat huntingtin (172). Knock-in murine models include Hdh Q71,Hdh Q92, Hdh Q94, Hdh Q111, Hdh Q140, and Hdh 150, where the number corresponds to CAG repeats added to human/murine chimeric exon 1 (173–175). Hdh Q92 and Hdh Q111 mice contain human/murine chimeric exon 1 with 92 and 111 CAG repeats, respectively (176–178). Hdh 150 mice contain murine exon 1 with 150 CAG repeats (179, 180).
4.1 Neurologic Signs
When lifted by the tail, R6/1 and R6/2 mice display hindlimb clasping, with earlier onset in the latter, presumably owing to a greater number of polyglutamatine repeats (168). Paw clasping was also noted in HD transgenic mice with 48 and 89 but not with 16 CAG repeats (169). Hdh 150 mice exhibit paw and body clasping, the latter defined by paws pressed next to the body (179, 180). No clasping was reported in Hdh Q111 (176) or Hdh Q71 and Hdh Q94 (173, 174) mice with fewer polyglutamatine repeats.
To our knowledge, the compulsory approaching syndrome seen after electrolytic caudate lesions (56) has not been reported in Huntington mouse models, nor have rooting and limb placing reflexes been reported.
4.2 Motor Activity
The time-related biphasic response seen in Huntington’s disease (42) was reproduced in R6/1 mice, showing more open-field activity than wild-type at a young age but less activity at an older age (181). The finding of lower open-field activity in R6/1 mice was confirmed (182). Likewise, R6/2 mice were more active in the open-field than wild-type at a young age but less active at an older age (183). The same biphasic response was reported in the Hdh Q94 model, with more rears than controls at a young age and fewer rears at an older age (175). Hypoactivity in R6/2 mice was noted both in open-field and wheel-running assays (184). Similarly, HD 48 and HD 89 transgenic mice (185) as well as Hdh Q94 (175) and Hdh 150 (179) knock-in mice had lower open-field activity than nonmutants.
4.3 Motor Coordination
R6/1 mice were deficient relative to wild-type mice on stationary beam (186) and rotorod (182, 186, 187) tests. Likewise, R6/2 mice were slower than wild-type in traversing the stationary beam (188) and fell sooner from the rotorod (183, 184, 188). HD 48 and HD 89 transgenic mice also had rotorod deficits (185). Like R6/2 mice, Hdh 150 mice fell sooner from the rotorod and took longer to traverse the stationary beam (179, 180). The latter nevertheless did not fall sooner from a suspended wire, a sign of intact muscle strength (179). In contrast to the previous knock-in, Hdh Q92 mice were not deficient on the rotorod (178, 189), probably due to fewer polyglutamine repeats. Nevertheless, serial motor sequence learning with hole-pokes was impaired in Hdh Q92 mice (189). The rat model of HD also developed rotorod deficits (172).
4.4 Spatial Learning
R6/2 mice had impaired acquisition (183, 190, 191) of place learning in the Morris maze. R6/2 mice had normal probe trial performance at 3 weeks of age (190) but were impaired at 5 (183) and 7 weeks (191). Their visuomotor guidance was normal at 3 (190) and 5 (183) weeks. Spatial deficits in this model also extend to learned left-right alternation (190). Spatial deficits in R6/1 mice have been described in the Barnes circular maze, requiring hole-poking to escape from a brightly lit area (192).
4.5 Nonspatial Discrimination Learning
R6/2 transgenic mice have normal light-dark acquisition for food reward (190).
5 Summary
Experimentally induced lesions of basal ganglia cause behavioral anomalies, some of which have been described in genetically modified mice for Parkinson’s and Huntington’s disease. As with the human disease, lesions to dopaminergic systems and motor coordination deficits are found in some murine models of Parkinson’s disease, including transgenic mice expressing mutant and wild-type SNCA encoding α-synuclein, Pitx3-deficient aphakia (ak) mice, and Park2 null mutants deficient in parkin. As with Huntington’s disease, HD transgenic and knock-in mice with variable CAG repeats possess the same time-related biphasic response in motor activity. Possible cognitive deficits have only begun to be explored and should include spatial and pattern discrimination tests, as well as evaluation of classical conditioning, bar-pressing, and pathologic reflexes, since these have been found to be sensitive to experimentally induced lesions.
Acknowledgement
This research was funded by a grant from the Natural Sciences and Engineering Research Council of Canada to R.L.
References
1.
Kandel ER, Schwartz JH, Jessell TM (1991) Principles of Neural Science, 3rd edn. Appleton and Lange, Norwalk, CT
2.
DeLong MR (1993) Overview of basal ganglia function. In: Mano N, Hamada I, DeLong MR (eds) Role of the cerebellum and basal ganglia in voluntary movement. Elsevier, Amsterdam
4.
Hikosaka O, Sesack SR, Lecourtier L, Shepard PD (2008) Habenula: crossroad between the basal ganglia and the limbic system. J Neurosci 28:11825-11829PubMedCrossRef
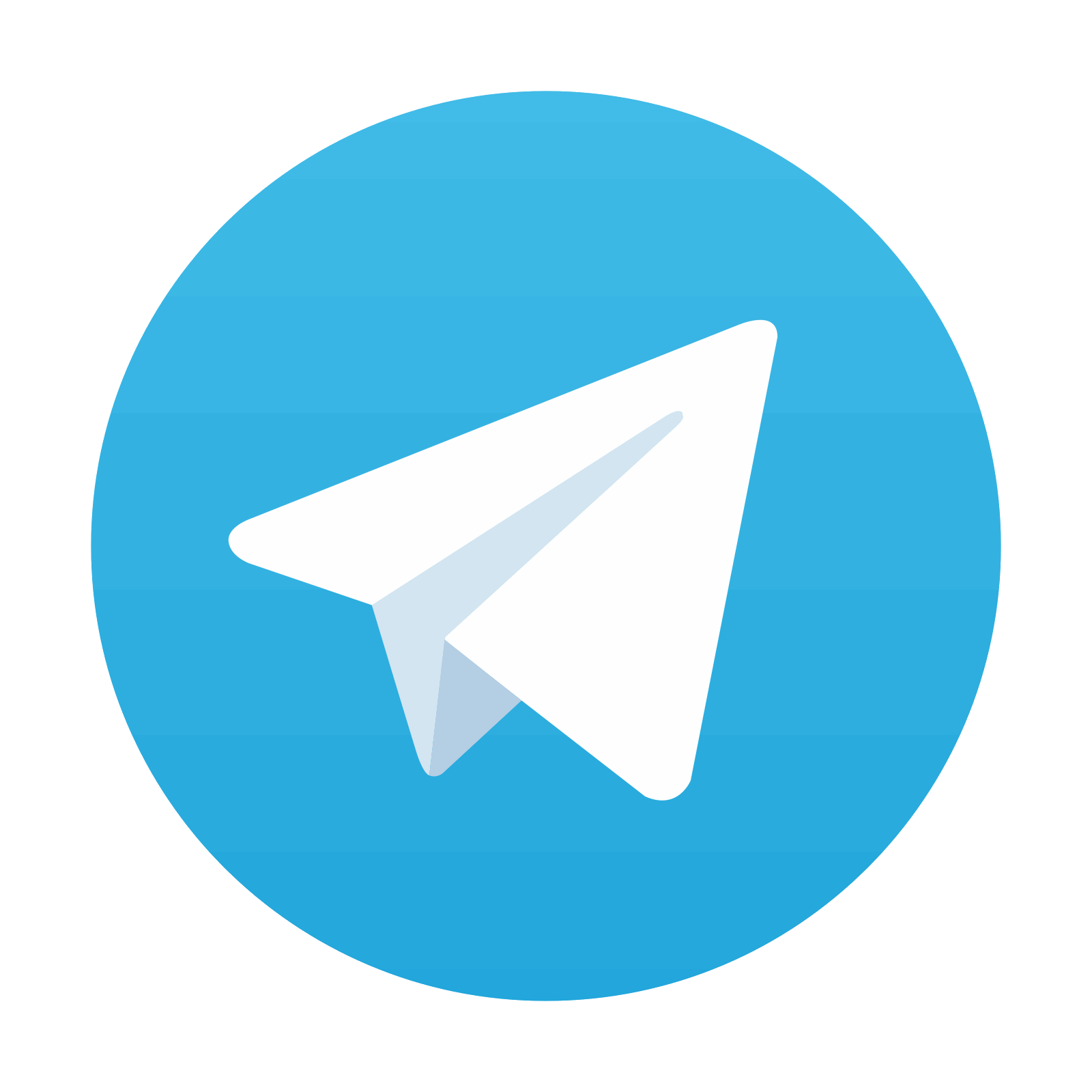
Stay updated, free articles. Join our Telegram channel

Full access? Get Clinical Tree
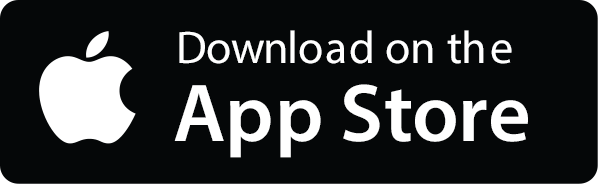
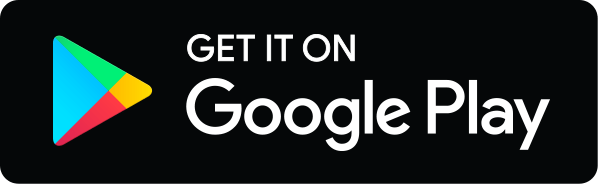