Fig. 7.1
Anatomy of the brain (From http://www.stanford.edu/group/hopes/basics/braintut/ab5.html, with permission from The Huntington’s Disease Outreach Project for Education at Stanford.)
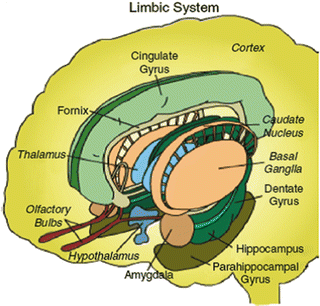
Fig. 7.2
Limbic system. (From http://www.stanford.edu/group/hopes/basics/braintut/ab5.html, with permission from HOPES.)
The ventromedial prefrontal cortex, with its extensive connections with the limbic system, may link the conscious to the unconscious and ascribe meaning to perceptions by associating them with a meaningful whole. The ventral tegmental pathway can also be activated by various substances including alcohol, amphetamines, exogenous and endogenous opiates, barbiturates, caffeine, marijuana, and nicotine.
All of these pleasure centers are interconnected and innervate the hypothalamus, particularly the lateral and ventromedial nuclei. The hypothalamus then activates the ventral tegmental area, as well as the autonomic and endocrine functions through the pituitary gland.
Aversive stimuli that provoke fight or flight responses activate the brain’s punishment circuit [the periventricular system (PVS)] to cope with unpleasant situations. This circuit includes the hypothalamus, the thalamus, and the central gray substance surrounding the aqueduct of Sylvius. Some secondary centers of this circuit are found in the amygdala and the hippocampus. The cholinergic punishment circuit stimulates the secretion of adrenocorticotropic hormone (ACTH) as well as the adrenal medulla and sympathetic outflow; ACTH in turn stimulates the adrenal cortex to release adrenocortical hormones. Stimulation of the punishment circuit can inhibit the pleasure circuit; thus fear and punishment can drive out pleasure.
The behavioral inhibition system (BIS), associated with the septohippocampal system, the amygdala, and the basal nuclei, receives inputs from the prefrontal cortex and transmits its outputs via the noradrenergic neurons of the locus ceruleus and the serotonergic fibers of the medial raphe nuclei. Serotonin may also play a major role in this system. The BIS is activated when both fight and flight seem impossible and the only remaining behavioral option is to submit passively.
When a sensory stimulus is perceived by the cortex to indicate a danger, it is routed first to the thalamus. From there, the information is sent out over two parallel pathways: the thalamo-amygdala pathway (the “short route”) and the thalamo-cortico-amygdala pathway (the “long route”). The short route quickly activates the central nucleus of the amygdala. Then the information that has been processed by the cortex through the long route reaches the amygdala and modifies its response dependent on the cortical evaluation of the threat. This cortical evaluation involves the following steps: (1) The various modalities of the perceived object are processed by the primary sensory cortex. Then the unimodal associative cortex provides the amygdala with a representation of the object. (2) The polymodal associative cortex conceptualizes the object and transmits the information to the amygdala. (3) This elaborated representation of the object is then compared with the contents of explicit memory available through the hippocampus, which also communicates closely with the amygdala.
The hippocampus is also involved in the encoding of the context associated with a fearful experience, i.e., memory. The amygdala conveys the gratifying or aversive nature of the experience through connections to the nucleus accumbens, the ventral striatum, the septum, the hypothalamus, the nuclei of the brainstem, and the orbitofrontal, cingulate, piriform, and other parts of the cortex. The combination of stimuli from the amygdala with working memory in the dorsolateral prefrontal cortex may constitute the experience of emotion. The basal ganglia have close connections with the amygdala and are involved with the voluntary expression of emotions. The amygdala has outputs to the nuclei of the sympathetic nervous system in the brainstem and the hypothalamus, controlling the pituitary gland and the endocrine system.
The anterior cingulate gyrus of the frontal lobe seems to be important in emotions and cognition. The subgenual anterior cingulate, together with the rostral cingulate, is considered to be the emotional sector of the anterior cingulate gyrus and it subserves autonomic arousal, reward mechanisms, and emotions, particularly anxiety and sadness in close coupling with the amygdala (Grady and Keightley 2002; Pezawas et al. 2005).
The dorsal portion of the anterior cingulate, called the cognitive cingulate, is involved with error monitoring and selecting among competing responses. Orbitofrontal cortex plays an important role in decision making in the context of emotional situations. Ventrolateral prefrontal cortex, together with the subgenual cingulate, plays a role in responding to reward contingencies (Fig. 7.3).
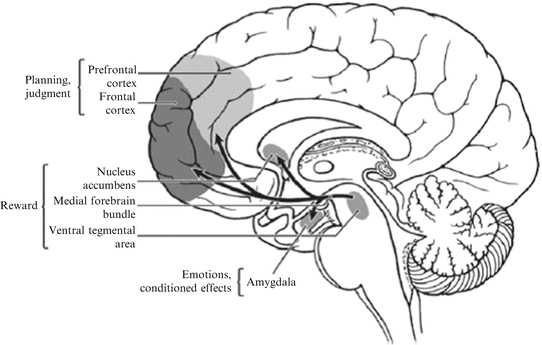
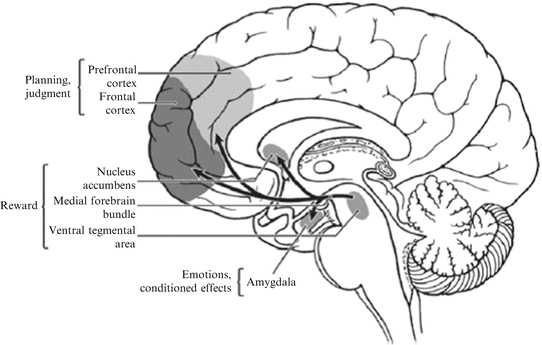
Fig. 7.3
Schematic diagram of the human brain that highlights some of the main brain areas and neurotransmitter pathways implicated in reward processes. (“Addiction and the brain: the role of neurotransmitters in the cause and treatment of drug dependence”—Reprinted from, CMAJ 20-Mar-01;164(6), Page(s) 817–821 by permission of the publisher. ©2001 Canadian Medical Association.)
7.2.2.2 Memory and Cognition
The dorsolateral prefrontal cortex seems to play an important role in reasoning. It stores the memories needed for doing tasks (working memory). The concept of working memory posits that a limited-capacity system temporarily stores information and thereby supports human thought processes. One prevalent model of working memory consists of three components: a central executive, a verbal storage system (“phonological loop”), and a visual storage system (“visuospatial sketchpad”) (Baddeley 2003). It has been proposed that the phonological loop evolved to facilitate the acquisition of language. Visuospatial working memory predicts success in fields such as architecture and engineering. The phonological loop is associated with the left temporoparietal region and activates the Wernicke’s and Broca’s areas. The visuospatial working memory is an associated analogous area in the right hemisphere and the visual cortex. The central executive, including the reasoning and decision-making function, is probably associated with the frontal lobes.
Declarative memory, that is, the memory of facts and events, seems to be a function of the hippocampus and its connections with the cortex. The hippocampus seems to connect various memory traces in the sensory and association cortices into discrete “episodes” that also include emotion-associated inputs from the limbic system. The hippocampus seems to enable us to “play a scene back” by reactivating this particular activity pattern in the various regions of the cortex. The hippocampus plays an essential role in the consolidation of short-term memory into long-term memory, which may represent various cortical regions activated during an event becoming so strongly linked with one another that they would no longer need the hippocampus to act as their link. Thus, information that has been encoded in long-term memory no longer requires the intervention of the hippocampus. This is the case in particular for general knowledge (semantic memory), which is associated with the activation of the frontal and temporal cortices. The activity in the temporal lobe may correspond to the activation of the fact in question, while the activity in the frontal cortex may correspond to its reaching consciousness. Spatial memory, unlike semantic or episodic memory, appears to be confined to the right hippocampus. Procedural memory, such as how to walk or ride a bike, seems to be stored in motor areas, cerebellum, amygdala, and the basal ganglia, particularly the striatum (Barnes et al. 2005).
7.2.3 Neurotransmitters and Brain-Derived Neurotrophic Factor (BDNF)
7.2.3.1 Neurotransmitters
Nerve transmission occurs when neurotransmitters bind to specific receptors in the postsynaptic neuron. In addition to potentially causing an action potential, neurotransmitters play a modulating role in the excitability of the neuron depending on specific receptor activation. Many chemicals serve the role of neurotransmitters, including acetylcholine, biogenic amines (dopamine, norepinephrine, serotonin, and histamine), amino acids [γ-aminobutyric acid (GABA), glycine, glutamate, aspartate], and neuropeptides (corticotropin-releasing hormone, corticotropin, ACTH, endorphins, substance P, somatostatin, bradykinin, vasopressin, angiotensin II).
There are two types of neurotransmitter receptors: ligand-gated receptors and G-protein-linked receptors. Stimulation of a ligand-gated receptor enables a channel in the receptor to open and permits the influx of chloride and potassium ions into the cell. The positive or negative charges that enter the cell either excite or inhibit the neuron. Ligands for these receptors include excitatory neurotransmitters, such as glutamate and aspartate. Binding of these ligands to the receptor produces an excitatory postsynaptic potential (EPSP). Binding of inhibitory neurotransmitter ligands, such as GABA and glycine, produces an inhibitory postsynaptic potential (IPSP). These ligand-gated receptors are also known as ionotropic or fast receptors.
G-protein-linked receptors are indirectly linked to ion channels through a second messenger system involving G proteins and adenylate cyclase. These receptors modulate the actions of the excitatory and inhibitory neurotransmitters such as glutamate and glycine. G-protein—linked receptors are known as metabotropic or slow receptors and examples include GABA-B, glutamate, dopamine (D1 and D2), and the 5-hydroxytryptamine (5-HT) receptors 5-HT1A, 5-HT1B, 5-HT1D, 5-HT2A, and 5-HT2C.
Several different neurotransmitters can be released from a single nerve terminal, including neuropeptides and biogenic amines. Neuropeptides can act as co-transmitters or primary neurotransmitters. As co-transmitters, they bind to specific presynaptic or postsynaptic receptors to alter the responsiveness of the neuronal membrane to the action of other neurotransmitters, such as norepinephrine and serotonin.
Glutamate is a pivotal amino acid in the brain. It is derived from α-ketoglutarate, which is one of the intermediates in the Krebs cycle. Glutamatergic neurons are extensively distributed in the brain, comprising more than 50 % of the neurons. Glutamate is an excitatory neurotransmitter. There are four types of glutamate receptors: the NMDA (N-methyl-D-aspartate) receptor, the AMPA (α-amino-3-hydroxy-5-methyl-4-isoxazole propionic acid) receptor, the kainate receptor, and the metabotropic receptor. The NMDA receptor is regulated by at least five binding sites, that is, those for (1) glutamate, (2) glycine, (3) magnesium, and (4) zinc, and a site that binds to (5) phencyclidine (PCP). The NMDA receptors have a capacity for long-term potentiation (LTP), an activity-dependent increase in synaptic efficiency that is crucial for learning and memory. The NMDA receptors are most densely concentrated in the cerebral cortex, especially the hippocampus, the frontal lobes, the amygdala, and the basal ganglia.
Excess glutamate has been shown to be neurotoxic, and an inability to remove glutamate by glial cells, thus eventually resulting in a hypofunction of the glutamatergic neurons, may underlie the cognitive deficits in schizophrenia as well as in certain dementias.
Nitric oxide may be synthesized and released when the NMDA receptor is stimulated by glutamate, enhancing neurotransmitter release from adjacent synapses and playing a role in LTP. Granule cells of the dentate gyrus of the hippocampus are rich in nitric oxide synthetase.
γ-Aminobutyric acid (GABA) is derived from glutamate and is distributed extensively throughout the brain, playing an inhibitory role. Following its release, GABA can be taken up by the neurons or by astrocytes. There are two basic GABA receptors, GABA-A and GABA-B. Stimulation of the GABA-A receptor increases the permeability to chloride ion, resulting in a hyperpolarization of the neuron or inhibition. The GABA-A receptor has three basic binding sites: for GABA, for benzodiazepine, and for barbiturates. The GABA-B receptor is a G-protein-related receptor that increases the efflux of K+ from the cell, causing hyperpolarization. The principal effect of GABA-B agonism is muscle relaxation.
Dopaminergic neurons are widely distributed throughout the brain in three pathways: the nigrostriatal, the mesocorticolimbic, and the tuberohypophysial. There are two primary dopamine receptor types, D1 and D2, both of which act through G proteins. D2 receptors often function as autoreceptors, providing negative feedback.
The mesolimbic and mesocortical dopaminergic systems play an important role in motivation, by attaching cognition of incentive to stimuli. Cocaine increases dopaminergic activity in the mesolimbic areas by inhibiting dopamine reuptake in the ventral tegmental area and the nucleus accumbens. Amphetamine seems more generalized in its action, not only by inhibiting reuptake but also by releasing dopamine from most brain regions. Both cocaine and amphetamine produce feelings of psychological energy and arousal, and cause diminished appetite and sleep. Both cocaine and amphetamine can cause visual and tactile hallucinations and paranoia.
Perception of time intervals may be mediated by dopaminergic spiny neurons located in the striatum of the basal ganglia. Marijuana slows subjective time by lowering the amount of dopamine available, whereas cocaine and methamphetamine accelerates the sense of time by increasing dopamine availability.
Dopaminergic neurons are important in pleasure and reward systems as well as in regulation of movement. They are also important in sustaining attention and concentration.
Overstimulation of D2 receptors in the mesolimbic and mesocortical systems may result in psychotic symptoms, as D2 agonists such as amphetamines can cause them and antagonists like haloperidol can reverse them. An overstimulation of striatal D2 receptors and an understimulation of cortical D1 receptors have been postulated for schizophrenia.
There is a significant relationship between dopamine and GABA neurons. In general, GABA acts to reduce the firing of the dopaminergic neurons in the tegmentum and substantia nigra. It forms the basis for benzodiazepine augmentation in the treatment of psychosis. In addition, benzodiazepines may be helpful in cases where there is an overactivity of dopamine in the motor striatum such as Huntington’s chorea or tardive dyskinesia. The feedback inhibition from the GABA neurons of the globus pallidus and putamen to the dopaminergic neurons of the substantia nigra is an important modulating force on the activity of the dopamine neurons.
The natural brain amine phenylethylamine (PEA), which is also found in chocolate, has been associated with sexual attraction and emotional infatuation. Phenylethylamine concentrations are high in the nucleus accumbens and the frontal and cingulate cortices. Levels spike during orgasm and ovulation. Phenylethylamine is very similar to amphetamine in chemical structure and likewise may act by causing dopamine release, but endorphin release may also be a significant effect.
Histamine is synthesized in the tuberomammillary nucleus of the posterior hypothalamus. These neurons project diffusely to most cerebral areas including the suprachiasmatic nucleus (SCN) and have been implicated in regulating circadian rhythms, ACTH secretion, cardiovascular control, thermoregulation, food intake, and memory formation. Outside of the central nervous system (CNS), histamine is stored in mast cells, whose release causes the allergic reaction. There are three types of histamine receptors: H1 receptors, which are widely distributed in the body; H2 receptors, which are distributed in the stomach and the heart; and H3 CNS autoreceptors. There is close interaction between histamine and serotonin, as both are concerned with circadian rhythms and feeding behavior, and in the CNS there is a stimulatory effect of endogenous serotonin on histamine release.
Serotonin is synthesized from the amino acid, L-tryptophan, which readily crosses the blood–brain barrier unlike serotonin itself. Most L-tryptophan that crosses the blood–brain barrier becomes serotonin. Meals high in branch-chained amino acids and L-tryptophan or in carbohydrates increase insulin secretion, which facilitates the transport of the branch-chained amino acids into muscle cells, thereby reducing the competition for L-tryptophan for the large neutral amino acid transporter that will transport it across the blood–brain barrier. This increase in serotonin often results in drowsiness. The pineal body has the richest concentration of serotonin in the brain, but the pineal serotonin is used for synthesis of melatonin rather than as a neurotransmitter. Serotonin neurotransmitter neurons are located in the raphe nuclei. The caudal nucleus projects largely to the medulla and spinal cord for the regulation of pain perception. The rostral nucleus projects extensively to the limbic system and the cerebral cortex. In the limbic system, the serotonergic receptors are co-localized with norepinephrine receptors, and serotonin and norepinephrine may work in conjunction in the regulation of arousal. At least 15 subtypes of serotonin receptors have been identified.
The suprachiasmatic nucleus (SCN) of the hypothalamus regulates the mammalian circadian clock. It is richly innervated by serotonergic input from the dorsal raphe nucleus. When light is present, the release of melatonin by the SCN is inhibited. Serotonin also seems to inhibit the responsiveness of the SCN to light.
Serotonin plays an important role in sleep, satiety, and mood. Serotonergic hypofunction in certain areas may underlie depression, aggression, and impulsive behavior.
About 95 % of serotonin in the body is outside of the CNS, mainly in the intestines. There are seven families of serotonin receptors, among which 5-HT1 and 5-HT2 receptors are mostly in the brain, and 5-HT3 and 5-HT4 receptors are mostly in the gut.
When stretch receptors on the gut wall are stimulated, serotonin, which enhances peristalsis, is released. Vagus nerve stimulates serotonin release in the gut. Current antidepressants that block reuptake of serotonin at synapses (SSRIs) often have gastrointestinal side effects.
Serotonin syndrome is a potentially life-threatening adverse reaction to drugs that increase serotonin levels in CNS and the body. Signs of excess serotonin range from tremor and diarrhea in mild cases to akathisia, clonus, delirium, neuromuscular rigidity, and hyperthermia in life-threatening cases. Patients usually manifest mydriasis, hyperactive bowel sounds, and diaphoresis, and show flushing or normal skin color. Creatine phosphokinase (CPK) is usually normal. Serotonin syndrome usually develops rapidly following the ingestion of drug, often in an overdose or drug interaction (e.g., a serotonin reuptake blocker with a monoamine oxidase inhibitor). Management of the syndrome involves the removal of the precipitating drugs, supportive care, control of agitation, prescribing serotonin antagonists (e.g., cyproheptadine), the control of autonomic instability, and the control of hyperthermia. Benzodiazepines are helpful for muscular rigidity and agitation. Olanzapine and chlorpromazine parenterally may also be helpful (Boyer and Shannon 2005; also go to http://www.benbest.com/science/anatmind/anatmd10.html).
Norepinephrine (noradrenaline)-containing neurons arise mainly from the locus ceruleus in the pons. They project extensively to the cortex, hippocampus, thalamus, and midbrain. Norepinephrine tends to increase the level of excitatory activity within the brain, and seems to be particularly involved in attention, arousal, and anxiety. Norepinephrine is the neurotransmitter of the sympathetic nervous system, and its activation, as in anxiety, has major effects on heart rate and blood pressure. There are benzodiazepine receptors in the locus ceruleus, as well as opiate receptors and α2-autoreceptors, that all reduce its firing. The beta-blocker propranolol also has been used to treat anxiety. By blocking the adrenergic inputs to the amygdala, beta-blockers may inhibit the formation of traumatic memories. Cortisol stimulation of the locus ceruleus due to chronic stress exacerbates norepinephrine stimulation of the amygdala.
Acetylcholine is abundant in the interpeduncular nucleus located near the substantia nigra, and all the interneurons in the striatum and the nucleus accumbens are cholinergic. The septum provides cholinergic fibers to the septal hippocampal tract. The primary cholinergic input to the cerebral cortex comes from the basal nucleus of Meynert, which also innervates the basolateral amygdala, the basal ganglia, and the reticular nucleus of the thalamus. Cholinergic transmission is important in cognition and sleep, and is the main neurotransmitter in the parasympathetic nervous system as well as in muscles. There are two types of cholinergic receptors: fast-acting nicotinic receptors and slow-acting muscarinic receptors. The presynaptic parasympathetic fibers and fibers innervating the voluntary muscular system are nicotinic, which are antagonized by curare. Atropine, which blocks the muscarinic receptors, can cause memory loss, and there seems to be a deficiency of muscarinic cholinergic transmission in patients with Alzheimer’s disease. Postganglionic parasympathetic fibers are also muscarinic.
Oxytocin is a nonapeptide released from the paraventricular nucleus of the hypothalamus through the posterior pituitary. It is a central mediator of pro-social behavior. Oxytocin is also released during stress and is an important modulator of anxiety and fear response (McCarthy et al. 1996).
7.2.3.2 Significance of Neurotransmitters in Psychiatry
Functional changes in neurotransmitters have been implicated in many psychiatric syndromes. Most antidepressant drugs enhance the functional availability of biogenic amines (dopamine, serotonin, norepinephrine) in the synapse either through reuptake blockade, blocking degradation, or direct agonistic action. Most antipsychotic drugs, particularly the first generation antipsychotics block the dopamine D2 receptors in the brain. Benzodiazepines, the principal antianxiety drugs, are GABA agonists.
7.2.3.3 Brain Derived Neurotrophic Factor (BDNF)
BDNF is a protein secreted in many cells by the endoplastic reticulum. It is encoded in BDNF gene, and it acts on neurons of the CNS and the peripheral nervous system, helping to support the survival of existing neurons and enhancing neurogenesis and the growth and differentiation of new neurons and synapses (Acheson et al. 1995; Huang and Reichardt 2001). BDNF is widely distributed—in the kidneys, retina, and motor neurons as well as in the brain. In the brain, it is particularly active in the hippocampus, cortex, and basal forebrain—areas vital to learning, memory, and higher thinking. BDNF plays an essential role in long-term potentiation (LTP) and the formation of long-term memory (Bekinschtein et al. 2007, 2008a, b, 2013, 2014; Yamada and Nabeshima 2003).
BDNF binds to at least two receptors, TrkB (pronounced “Track B”) and the LNGFR (for low-affinity nerve growth factor receptor, also known as p75) (Patapoutian and Reichardt 2001; Reichardt 2006). It may also modulate the activity of various neurotransmitter receptors, including the Alpha-7 nicotinic receptor (Fernandes et al. 2008).
There is evidence that BDNF expression is decreased in patients with depression or schizophrenia and that BDNF levels may predict the outcome of antidepressant treatment (Tadic et al. 2011; Thompson Ray et al. 2011).
In fact, most antidepressant drugs and electroconvulsive therapy eventually increase BDNF levels (Haghighi et al. 2013; Okamoto et al. 2008; Ryan et al. 2013; Sen et al. 2008). Exercise and caffeine also increase BDNF levels (Adlard et al. 2005; Costa et al. 2008a, b; Cotman and Berchtold 2002).
Traditional antidepressants require many weeks before therapeutic effects occur.
Ketamine intravenous administration in sub-anesthetic doses has been shown to result in rapid antidepressant response in a matter of hours in treatment resistant depressed patients. Ketamine is an NMDA receptor antagonist and participates in the regulation of glutamatergic transmission. There is robust evidence that the glial cells, astrocyte and satellite oligodendrocyte populations are reduced in prefrontal cortex and other cortical regions in postmortem tissue from individuals who had suffered from major depression and bipolar disorder and ketamine has been shown to be effective in both types of depression.
The glial loss in depression may reflect the convergence of many features of stress response in animal models, including immunologic attack on glial integrity due to elevated brain cytokine and cortisol levels, increased oxidative stress, and reduced levels of free radical scavengers including glutathione, and stress-induced glutamate release. Glial loss has a number of downstream consequences that may lead to the pathophysiology of major depression. Glial loss, produced by specific glial toxins, produces biochemical and behavioral signs of depression in animal models (Banasr et al. 2010; Sanacora and Banasr 2013). Because glia are centrally involved in glutamate inactivation, glial loss may elevate glutamate levels in both synaptic and extrasynaptic spaces. Overstimulation of presynaptic receptors has been shown to depress glutamate neurotransmission and compromise synaptic connectivity, consistent with the association of elevated anterior cingulate glutamate levels and reduced cingulate functional connectivity in major depression (Horn et al. 2010). A consequence of elevated extrasynaptic glutamate levels is overstimulation of extrasynaptic NMDA receptors. Overstimulation of these receptors has a number of downstream consequences including a reduction in BDNF levels, culminating in dendritic regression and activation of apoptosis. Thus, glial deficits in depression may contribute to abnormalities in cortical functional connectivity by compromising structural connectivity and dysregulating glutamate synaptic transmission. (Horn et al. 2010; Kavalali and Monteggia 2012; Krystal et al. 2013; Liu et al. 2013, 2012).
Thus, ketamine and other glutamatergic drugs might target rapid BDNF enhancement, which may result in rapid antidepressant action as well as cognitive enhancement (Krystal et al. 2013; Salvadore and Singh 2013).
There is a recent intriguing report indicating an increase in the methylation status (i.e., inactivation) of the BDNF gene in borderline personality disorder patients who had childhood abuse history, and that responders to dialectical behavioral therapy showed an eventual decrease in the BDNF methylation, while non-responders showed an increase in methylation (Perroud et al. 2013). Thus, the epigenetic changes of the BDNF gene through the experience of childhood abuse may be reversed through psychotherapy in adulthood.
7.2.4 The Brain in Psychiatric Syndromes
7.2.4.1 Prefrontal Cortex
In the prefrontal cortex, the ventromedial cortex seems affected by both depression and mania. It is located on either side of the center line separating the two hemispheres and is closely involved with the pleasure circuit. It also seems to be involved in switching from one kind of affect to another. The ventromedial cortex has extensive connections with the limbic system (Fellows and Farah 2003). The volume of the ventromedial cortex is decreased in chronic depressives, mostly owing to a decrease in the number of glial cells.
There seems to be a hyperactivity of the rostral and subgenual cingulate (Area 25) in depression, and hyperactivity of the rostral cingulate may be a predictor of the response to antidepressant therapy. Recently, a number of studies indicate that there is impairment of the connectivity and function of subcallosal anterior cingulate in major depression, PTSD, as well as anorexia nervosa, and deep brain stimulation of the area may be effective in a variety of conditions. (Davey et al. 2012; Grady and Keightley 2002; Holtzheimer et al. 2012; Lane et al. 2013; Lipsman et al. 2013; Pizzagalli et al. 2001; Siegle et al. 2012; Tripp et al. 2012). Anterior cingulate and amygdalar activation has been shown to predict the response to cognitive behavioural therapy in PTSD (Bryant et al. 2008), and CBT for depression has been shown to change the activity of anterior cingulate and medial prefrontal cortex (Yoshimura et al. 2014).
The dorsolateral prefrontal cortex (DLPFC) is involved in executive functions and working memory, which are particularly disturbed in schizophrenia. In schizophrenia, there is cortical thinning within the cingulate, occipital, and frontopolar cortices, and a reduction in overall brain volume. Functional imaging studies show a reduction in neuronal density and activity in the prefrontal cortex and hippocampus in schizophrenia (Jann, 2004).
7.2.4.2 Locus Ceruleus
The locus ceruleus, which supplies a majority of noradrenergic neurons in the brain, plays an important role in anxiety and in activating physiologic and behavioral arousal.
7.2.4.3 Basal Ganglia
The basal ganglia, including the caudate and substantia nigra and thalamus, show hyperactivity in obsessive-compulsive disorder (OCD), and dopaminergic corticostriato-thalamic circuit dysfunction has been postulated for this disorder (Graybiel et al. 2000). Provocation of OCD symptoms is associated with an increase in cerebral blood flow in the orbitofrontal cortex, anterior cingulate cortex, striatum, and thalamus (Lagemann et al. 2012; McGuire et al. 1994; Rauch et al. 1994). Hypofunction of serotonergic neurons arising from the rostral raphe nucleus may result in a lack of inhibitory effect on the putative OCD pathway. Successful treatment with pharmacotherapy or cognitive-behavioral therapy has resulted in reversal of some of the brain activation (Carey et al. 2004; Figee et al. 2013; Schwartz et al. 1996).
7.2.4.4 The Limbic System and the Amygdala
Intimately involved in all things emotional, the limbic system, and particularly the amygdala, is dysregulated in psychiatric syndromes. Functional imaging studies show an increase in activation of the limbic structures, including the amygdala, hippocampus, and adjacent temporal cortex, in anxiety states including phobia. As described below, a dysfunction of the circuit that connects the amygdala to the anterior cingulate gyrus may underlie anxiety and depressive syndromes.
7.2.5 Final Common Pathways: Genes, Memes (Memory), Stress, Brain Function, and Psychiatric Syndromes
The net result of activations of various parts of the brain manifests itself in the efferent pathways of behavior (motoric), in the hypothalamic–pituitary–adrenal axis (autonomic and endocrine), and in intracranial subjective cognitive and emotional experiences. When these manifestations are dysfunctional or disordered, a psychiatric syndrome results.
We are at the threshold of understanding the genetic mechanisms of emotions and psychosis, and of understanding how some of the genes might be turned on or off by experiential, environmental, and developmental factors(i.e., epigenetics) and how such experiential factors affect brain structures and function, resulting in normal emotions/behavior or a psychiatric syndrome.
As far as psychiatric diagnosis goes, the current state of affairs concerning genes can be summarized as follows: for each diagnostic category, there are many susceptibility genes, and a single gene or a few genes may code for the susceptibility for many different disorders.
7.2.5.1 Serotonin Transporter Gene, Depression, Anxiety, and Neuroticism
An example of a single gene that codes for the vulnerability to multiple psychiatric (and medical) conditions is the serotonin transporter gene (SERT) and its promoter region polymorphism (5-HTTLPR). SERT is highly evolutionarily conserved, and regulates the entire serotoninergic system and its receptors. DNA screenings of patients with autism, attention-deficit hyperactivity disorder, bipolar disorder, and Tourette’s syndrome have detected signals in the chromosome 17q region where SERT is located (Murphy et al. 2004a) .
The 5-HTTLPR polymorphism consists of short (s) and long (l) alleles, and the presence of the short allele tends to reduce the effectiveness and efficiency of SERT .
A variable number of tandem repeats in the serotonin (5-HT) transporter gene linked polymorphic region (5-HTTLPR), located at the SLC6A4 locus on chromosome 17 (17q11.1–17q12) influences the transcriptional activity and subsequent availability of serotonin [see National Center for Biotechnology Information (NCBI) map Web site for 5HTTLPR gene at http://www.ncbi.nlm.nih.gov/mapview/maps.cgi?taxid=9606&chr=17&MAPS=morbid,genec,ugHs,genes,pheno%5B24579425.12:27953447.37%5D-r&query=uid(7812322,5968)&QSTR=SLC6A4.] The 5-HTTLPR short allele “s” has reduced transcriptional efficiency compared with the long allele, and individuals carrying the “s” allele tend to show increased anxiety responses and seem to show an increased risk of depression (Lotrich and Pollock, 2004). The short-allele carriers show reduced gray matter in limbic regions critical for processing of negative emotion, particularly the perigenual cingulate and amygdala.
Functional magnetic resonance imaging (MRI) studies of fearful stimuli show a tightly coupled feedback circuit between the amygdala and the cingulate, implicated in the extinction of negative affect. Short-allele carriers showed relative uncoupling of this circuit, and the magnitude of coupling inversely predicted almost 30 % of variation in temperamental anxiety (Pezawas et al. 2005). Pezawas et al. (2005) showed that the short-allele carriers show reduced gray matter in limbic regions critical for the processing of negative emotions, particularly the subgenual cingulate and amygdala. They also show increased amygdala activation to fearful stimuli (Beevers et al. 2011; Bertolino et al. 2005; Hariri et al. 2002) . Thus, this gene seems to increase the sensitivity of the affected individual’s brain to negative affect and anxiety (Caspi et al. 2010; Sugden et al. 2010; Uher et al. 2011)
The short-allele carriers have also been reported to have an increased risk for irritable bowel syndrome (Yeo et al. 2004) and migraine (Gonda et al. 2007). 5-HTTLPR short-allele carriers with neuroticism have been found to be more likely to smoke, especially to reduce negative mood and to feel stimulated, and have the most difficulty in quitting smoking (Hu et al. 2000; Lerman et al. 2000). On the other hand, the long allele has been reported to be associated with increased cardiovascular disease and reactivity (Brummett et al. 2011).
A dietary deficiency in the serotonin precursor, tryptophan, has been shown to induce depression in healthy women with the 5-HTTLPR s/s, regardless of their family history of depression, while those with l/l were resistant to depression regardless of family history of depression (Neumeister 2003; Neumeister et al. 2006)
Caspi et al. have shown, in an elegant longitudinal study, that stress during the previous 2 years in adulthood and maltreatment in childhood interacted with the 5-HTTLPR status. Individuals with 2 copies of the short allele who also had the stressors had greatest amount of depressive symptoms and suicidality than heterozygous individuals, and those with only the long alleles had the least amount of depression(Caspi et al. 2002; Caspi et al. 2003; Enoch 2006; Machado et al. 2006; Stein et al. 2008).
Thus, the 5-HTTLPR short allele, in conjunction with childhood stress, may confer an individual with a trait of responding to later stress with increased anxiety, neuroticism, and subclinical depression (Gonda et al. 2005), which, in turn, may predispose the individual for later major depression, suicidality, bulimia (Ribases et al. 2008) and psychophysiologic disorders.
Studies in monkeys have shown that the anxiety-enhancing effect of the short allele is mitigated with good mothering in infancy (Barr et al. 2004; Suomi 2003, 2005).
5-HTTLPR may also determine the response to drugs. Depressed individuals with the short allele were found to respond better to antidepressants that are both serotonergic and noradrenergic (i.e., mirtazapine), rather than serotonin specific reuptake blockers. On the other hand, individuals with the long allele may have more side effects with exactly those drugs that are more effective for those with the short allele (Murphy et al. 2004b) . This is an elegant example of genetic polymorphism and resulting brain structural variations interacting with stress and producing susceptibility to anxiety and depression, and influencing the treatment choice.
There is evidence that 5HTTLPR polymorphisms may have differential effects on ethnicity, gender, and age. For example, females with the short allele may be more susceptible to depression (Beaver et al. 2012), and Caucasians with the short allele may be more susceptible to depression (van Ijzendoorn et al. 2012). The short allele interacted with environmental factors in the self esteem of adolescents (Jonassaint et al. 2012).
Why does a single gene code for so many traits, both adaptive and maladaptive, and vulnerabilities? One simple answer may be that the gene codes for one or more basic evolutionarily adaptive predispositions that, in combination with other factors, may determine the development and severity of a psychiatric syndrome. There is also the effect of other genes that may interact with the gene in question (epistasis) (Goenjian et al. 2012; Thaler et al. 2013).
When we look at the list of conditions affected by 5HTTLPR polymorphism, it seems clear that there is a continuum, from anxiety to adaptive/maladaptive behavior to phobia to major depression, and/or to physical symptoms (Fig. 7.4).
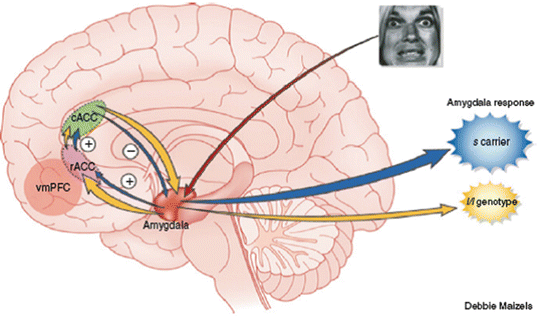
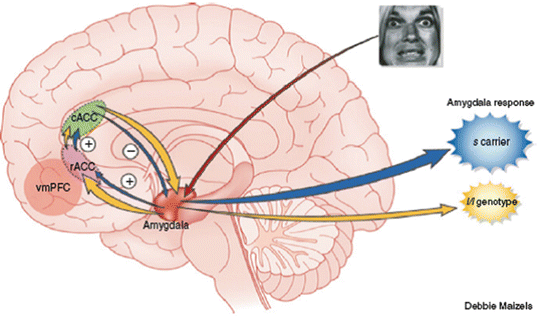
Fig. 7.4
Serotonin transporter promoter polymorphism (5-HTTLPR s/s) and emotional hypersensitivity to negative stimuli. Differences in processing of emotional stimuli between s allele carriers (darker arrows) and homozygous l allele carriers (light arrows). Negative emotional stimuli are evaluated by the amygdala (arrow from eyes) after preliminary analysis in the ventral visual pathway (not shown). Carriers of the s allele have markedly reduced positive functional coupling between the rostral anterior cingulate (rACC; lower oval) and the amygdala, which results in a net decrease in inhibitory feedback from the caudal anterior cingulate (cACC; upper oval), via connections between rACC and cACC (short upward arrows). Brain volume was also substantially reduced in s allele carriers in the rACC and, to a lesser extent, the cACC and amygdala. The consequence of these genotype-based alterations is an emotional hyperresponsivity to negative affective stimuli in s allele carriers (large dark cloud) compared with individuals lacking this allele (small lighter cloud), which may be related to an increased risk of developing depression. As found in a previous study, functional coupling between the vmPFC (light circle) and the amygdala was also increased in s allele carriers. (From Hamann, 2005. Reprinted by permission from Macmillan Publishers, Ltd: Nat Neurosci, copyright 2005.)
7.2.5.2 Monoamine Oxidase Gene
Similar interactions have also been reported with functional polymorphism in the gene encoding the monoamine oxidase A (MAOA), located on the X chromosome Xp11.4-11.3 (for maps of genes, go to http://www.ncbi.nlm.nih.gov/projects/mapview/). It encodes the MAOA enzyme, which metabolizes neurotransmitters such as norepinephrine (NE), serotonin (5-HT), and dopamine (DA), and renders them inactive. Genetic deficiencies in MAOA activity have been associated with aggression in mice and humans. Maltreated males with the low-MAOA activity genotype were more likely than non-maltreated males with this genotype to develop conduct disorder in adolescence and be convicted of violent crimes in adulthood. In contrast, among males with high MAOA activity, maltreatment did not confer significant risk for either conduct disorder or violent conviction (Caspi et al. 2002).
7.2.5.3 Stress and the Aging Process
Stress affects the aging process directly at the cellular level. Perceived stress and chronicity of stress was significantly associated with higher oxidative stress, lower telomerase activity, and shorter telomere length—known determinants of cell senescence and longevity. Telomeres are DNA–protein complexes that cap chromosomal ends, promoting chromosomal stability. When cells divide, the telomere is not completely replicated, leading to telomere shortening with every replication. In one study, women who had highest levels of perceived stress had telomeres that were, on the average, at least a decade older than women with lowest stress levels (Epel et al. 2004).
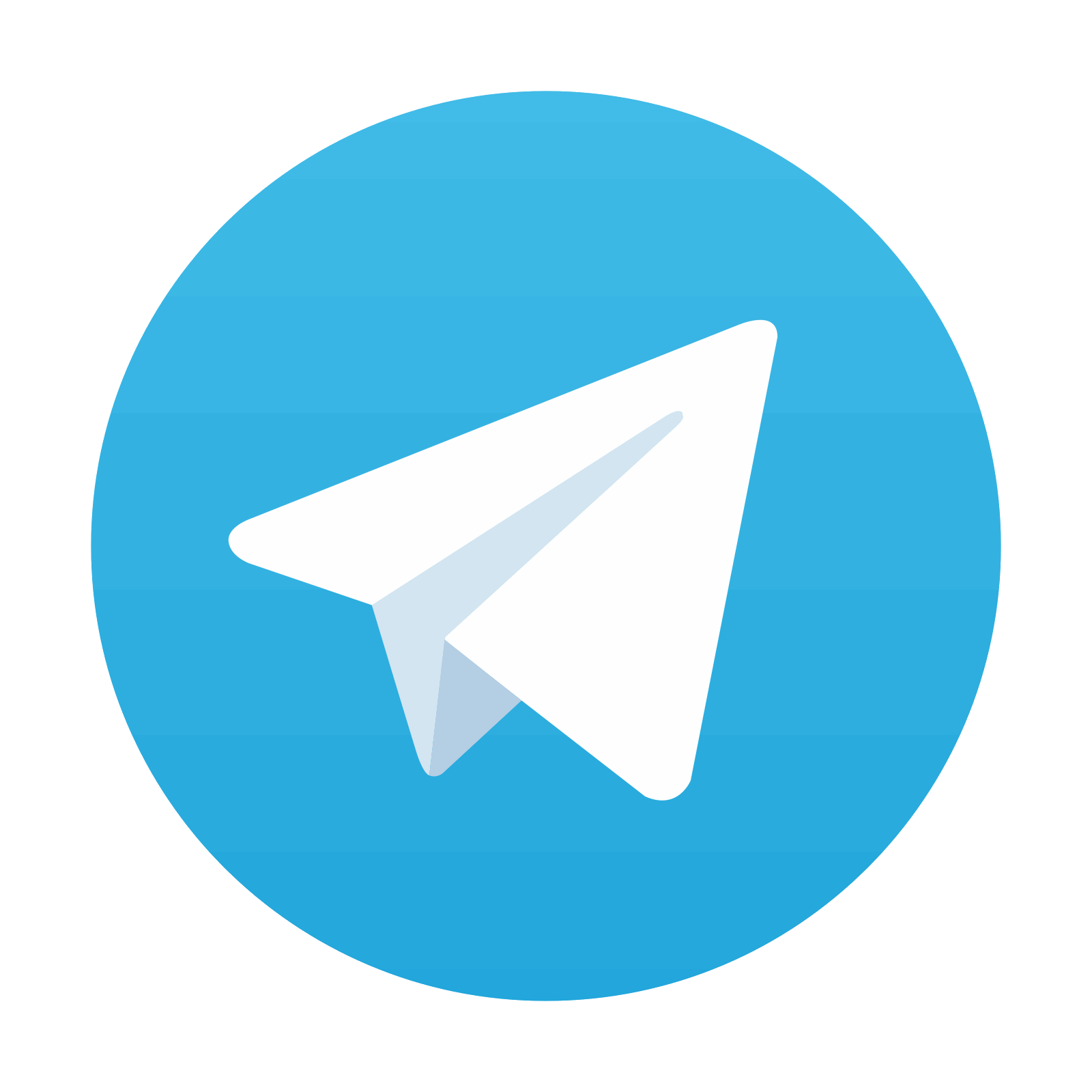
Stay updated, free articles. Join our Telegram channel

Full access? Get Clinical Tree
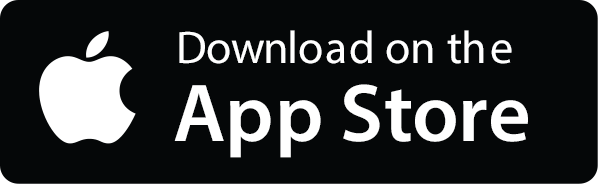
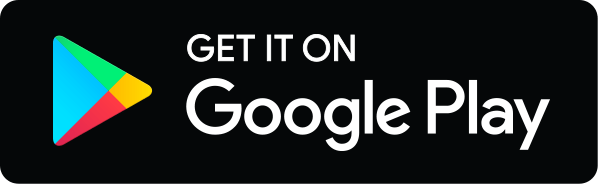