Fig. 4.1
3D printer managed and operated in the medical library by the medical students at the University of Ottawa, Ontario, Canada. Shown from left to right, Aili Wang, Isabelle Castonguay, Dr. Ali Jalali (physician lead), Geneviève Morin and Talia Chung in the Health Sciences Library, University of Ottawa Faculty of Medicine (Photo: Dave Weatherall, used with permission)
3D printing requires that medical images, typically in DICOM format, be converted to an output recognized by the 3D printer. The most common output is the Standard Tessellation Language (STL) format. This material has been covered in Chap. 3 and is also described in a review article from Mitsouras et al. (2015).
Mitsouras’ paper is designed as an easy-to-read treatise that is also available as a freely downloadable article. The annual meeting of the Radiological Society of North America has had several hands-on 3D printing courses; two of these have comprehensive step-by-step guides to 3D printing software that are also available as free downloads from 3D Printing in Medicine (Chepelev et al. 2016; Giannopoulos et al. 2015). The ensemble of these three articles and Chap. 3 will provide the reader with a sound foundation of methods and software manipulations typically encountered when using a medical image to make a handheld model.
As the program grows, it is important to establish key stakeholders centered on the patient. While this chapter is written from the perspective of physician leads, leadership can come from engineers and physicists with knowledge of the anatomy. As noted earlier, the referring clinicians who perform interventions must form a partnership with technical experts (whether they be physicians or engineers), and there is a large role for radiology technologists, other individuals to perform segmentation, individuals that include students to operate the printers and clean/prepare the models, and a specific individual to take the role as quality lead.
Segmentation requires experience and a meticulous approach and can be performed by physicians and technologists. While most models are generated from either CT or MRI, cases may require co-registration if both modalities are required to delineate specific pathology. While the typical diagnostic images are frequently usable for segmentation, repeated studies with external patient markers and specific volumetric sequences in the case of MRI or thin axial slices in the case of CT imaging may be needed. While it is not desirable, particularly in children and young adults, repeating an imaging study is sometimes the best pathway to optimum care to ensure segmentation accuracy, based on the spatial resolution needed, or the mitigation of artifacts in the initial images.
Once the lab includes a larger-format printer, the device itself requires engineering support; this will ensure adequate functioning, including regular maintenance and rigorous quality assurance to ensure prints that adhere to institution- and disease-specific error tolerance thresholds. While quality assurance is discussed in detail below, specific quality guidelines have not been established. However, recommendations are now emerging from societies such as the RSNA Special Interest Group to enhance quality of 3D models. In practice, tolerance thresholds for model accuracy are typically established upon discussion with the radiologists and interventionalists with specific disease pathophysiology and patient in mind. For example, a tumor with a 20 mm resection margin with no neurological involvement may be subject to less stringent criteria than an intracranial intraparenchymal mass in close proximity to major vasculature. Regardless of the accepted tolerance threshold and 3DP technology, regular maintenance and vigilant quality assurance are indispensable in ensuring 3DP program success.
As the lab progresses, undoubtedly the service line will receive requests to make more complicated models and to make changes to the anatomy (e.g., mirroring one side of the face) when helping to plan complex interventions. Thus, when formulating the hardware and software requirements for the lab, we present the following perspective for the common applications for medical 3D printing. These follow the outline from Christensen and Rybicki (2017), we have found that the most useful method to categorize the applications is by the intended use:
Group I . Anatomical Models. A model representing as-scanned anatomy. The intended use for anatomic models is procedural planning and/or a reference during the procedure, for education including simulation and for informed consent. The defining characteristic of Group I models is that what is printed is intended to exactly reproduce the anatomy captured by the medical imaging device.
Group II . Modified Anatomical Models. A modified model of anatomy, simple surgical planning performed digitally to further enhance the model, significantly modified models. For Group II models, the intended use is enhanced surgical planning and guidance, but unlike Group I models, the anatomy being held in the end users hand is purposely modified from the patient anatomy.
Group III . Virtual Surgical Planning with Templates. This group refers to complex surgical planning done digitally, for example, 3D-printed templates/models/guides produced which are intended to guide the digital plan on the patient in the operating room. The intended use for Group III models is to augment the surgical procedure with specific preplanned steps which are carried out in surgery using 3D-printed guides or templates.
These three groups are also used to organize workflow, elaborate on post-processing, and to put into framework important regulatory considerations. A common “first model” or experimental scenario begins with a CT scan of bone that is used to design Group I models, printed on a single-spool FDM printer. The CT scan undergoes 3D visualization using tools that are familiar to the radiologist. However, since these tools often do not have STL output or have an STL output that requires additional modifications before printing, out the outset, freeware for computer-aided design (CAD) is typically used to generate a printable STL file. The bone is very amenable to thresholding methods from noncontrast CT scans, simplifying the process of 3D printing.
While the barriers for a rudimentary 3DP program are relatively low, success is predicated upon clinical applications. One highly useful, early strategy for a developing in-hospital 3D printing lab is partnership between radiologists and one group of specialist physicians. Within that specialty, complex cases frequently require detailed discussions, supported by precise measurements of anatomical relationships as well as consideration of disease pathophysiology. The radiologist often prepares 3D visualization to augment these conversations, and very often 3D printing can further clarify complex anatomy. For example, in a relationship with orthopedic surgeons, specific models of bone tumors of the pelvis will prove useful to delineate surgical planes and help foster a relationship and confidence in the models for procedure planning.
A logical next step for a growing 3D printing lab is to add a second, relatively low budget vat polymerization system. With these systems, the scope of models will increase, and a modest-sized build tray will not be prohibitively expensive (Fig. 4.2). The acquisition of a vat polymerization system certainly could come before a FDM printer, but by the time both are in the lab, additional human resources will be necessary to manage the printers, clean the models, and manage orders from referring clinicians. With these experiences in hand, larger printers should then be considered, and with this comes a wide scope of services and collaborations within the institution and beyond. At present, there are a limited number of hospitals with advanced resources, making them desirable for partnerships as medical 3D printing continues its exponential growth.


Fig. 4.2
3D Printing at the Applied Imaging Science Laboratory , Boston, MA. From left to right: Anji Tang, Dimitrios Mitsouras PhD, Elizabeth George, MD
Typically derived from CT or MRI, Group I 3D models are designed to depict the anatomic information contained in the medical images, without alteration, and are generally used for surgical planning. Examples include a newborn with double outlet right ventricle (Medical Modeling 2012) or craniomaxillofacial models where tissues are not altered, for example, to plan complex procedures including congenital deformity corrections and secondary reconstruction following trauma (D’Urso et al. 1999; Yoo et al. 2015; Christensen et al. 2004). Orthopedic (Brown et al. 2003) and cardiovascular (Giannopoulos et al. 2016) applications include 3D-printed models for visualization and physical, hands-on simulation. The common denominator for Group I models is that 3D printing extends current 3D visualization and the model does not differ from the patient’s anatomy. Following the Modeling Flow Map of Christensen and Rybicki (2017), we maintain that steps A–E, including “3D Printing Preparation,” should use systems (software and some hardware) that are cleared by the FDA for their intended use. This includes not only the imaging hardware/software but also the software used to segment the medical images from their original 2D state into a 3D dataset usable for 3D printing (i.e., an STL or 3MF file) (FDA 2014a, b). Regarding the steps “Build Prep—Support, Slicing” to the end of the pathway when models are used by healthcare professionals, the FDA has commented that work in hospital is not under these auspices, and they do not contain what the FDA considers to be medical devices (Yoo et al. 2015).
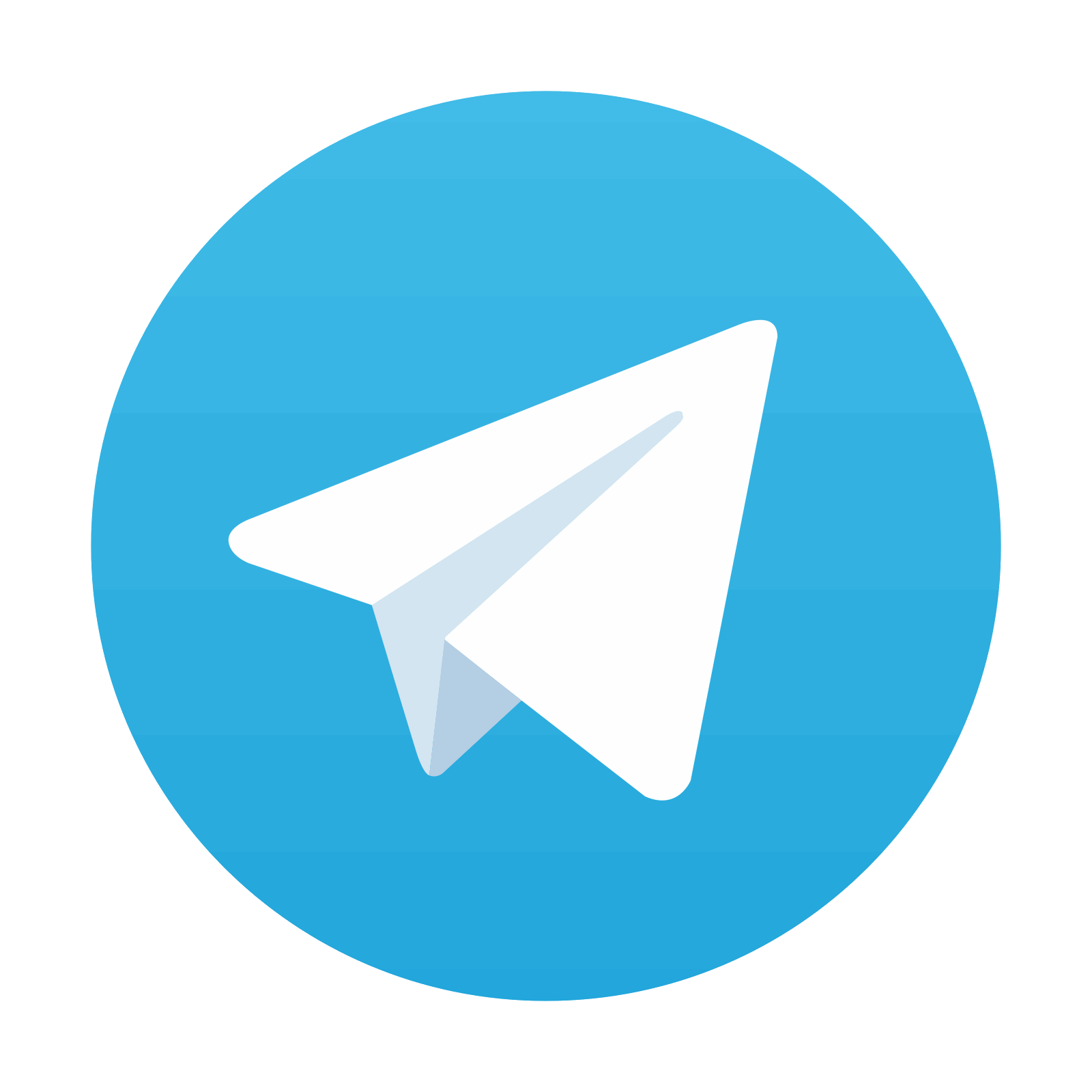
Stay updated, free articles. Join our Telegram channel

Full access? Get Clinical Tree
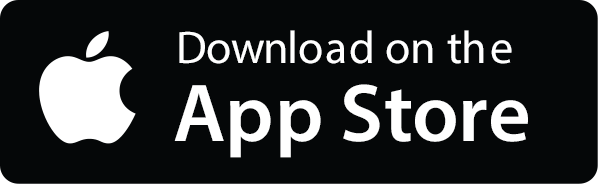
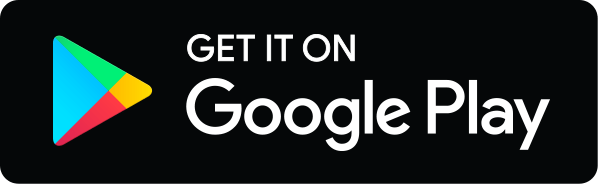