The most common primary brain neoplasm is glioblastoma multiforme, which is associated with a dismal prognosis. Despite the recommended treatment regimen of aggressive surgical resection, radiation, and chemotherapy, the median survival remains approximately only 14 months. Due to these minimal improvements in survival of patients despite recent advances in conventional treatments, new modalities such as immunotherapy are being investigated and studied. A hurdle to developing effective immunotherapy is the immunosuppressive characteristics that are the hallmark of malignant gliomas. Effective therapeutic strategies will require overcoming these mechanisms, by augmenting tumor antigen presentation, perhaps in a setting isolated from the tumor microenvironment. The heterogeneity of potential glioma antigens warrants potential targeting of multiple tumor-specific antigens, and discovery and investigation of additional antigens. This article describes the current strategies and principles of immunotherapy for malignant gliomas.
The most common primary brain neoplasm is glioblastoma multiforme (GBM), which is associated with a dismal prognosis. Despite the recommended treatment regimen of aggressive surgical resection, radiation, and chemotherapy, the median survival remains approximately only 14 months. Due to these minimal improvements in survival of patients despite recent advances in conventional treatments, new modalities such as immunotherapy are being investigated and studied. In this article the current strategies and principles of immunotherapy for gliomas is described.
The central nervous system (CNS) historically has been considered an immune-privileged organ where immune activity is significantly silenced. Several unique anatomic and physiologic characteristics limit immune surveillance and response functions in the brain, such as: (1) the CNS lacks a lymphatic system; (2) the brain is shielded from the peripheral circulatory system by the blood-brain barrier (BBB), and thus is isolated from most peripheral immune cells, soluble factors, and plasma proteins; (3) the high levels of immunoregulatory cells and factors in the brain further mute immune functions; and (4) cells of the CNS at baseline express very low levels of major histocompatibility complex (MHC) molecules responsible for antigen presentation to immune effector cells.
However, despite these factors minimizing CNS immune function, a highly adapted system of immune surveillance exists, and effective immune responses can occur in the CNS. Activity of both the complement system and the antigen-antibody system, including functional B cells, have been found within the CNS. During CNS insults, resident antigen-presenting cells (APC) of the CNS, microglia cells, undergo activation and upregulate both MHC and costimulatory molecules, and also contribute to both CD4+ and CD8+ specific T-cell responses. A small number of lymphocytes are found in normal, healthy brain, but both naïve lymphocytes and activated T cells have the ability to cross the BBB. Many different types of lymphocytes also infiltrate the CNS in the presence of a variety of CNS pathologies, including glioma. However, the magnitude and potency of these immune responses in the CNS still remains to be clearly elucidated.
Tumor-associated immunosuppression
Malignant brain tumors such as gliomas display an ability to evade and suppress the immune system. Gliomas can evade the immune system at different levels of antigen recognition and immune activation. First, by limiting effective signaling between glioma and immune cells, glioma cells evade immune detection. Many glioma cells express low levels of human leukocyte antigens (HLA) or express defective HLA. A recent report by Facoetti and colleagues described approximately 50% of 47 glioma samples displayed loss of the HLA type I antigen. Among these, 80% showed evidence of selective loss of HLA-A2 antigen. Of note, loss of HLA type I antigen was more common among higher grade tumors, suggesting a role of deficient antigen presentation in glioma progression.
Inhibition of antigen presentation by microglia and macrophages in the tumor microenvironment also contributes to the tumors’ ability to escape immune detection. In vitro, the presence of glioma cells induces monocytes to reduce their phagocytic activity. In addition, microglia found within glioma tissue appear to be deficient in proper antigen presentation for cytotoxic and helper T-cell activation. Schartner and colleagues demonstrated that MHC-II induction by stimulation was significantly less in microglia and infiltrating macrophages derived from gliomas than in those isolated from normal brain. The mechanism for this defective MHC-II response in tumor-associated microglia and macrophages remains unclear in regard to whether they result from a local immunosuppressive milieu, impaired signaling, or another unique inherent phenotype of tumor-associated microglia and macrophages. Stimulation of microglia in the presence of tumor cells reduces the secretion of proinflammatory cytokines, such as tumor necrosis factor (TNF)-α, but also increases the secretion of the inhibitory cytokine interleukin (IL)-10.
In the presence of glioma, T cells, specifically the CD4+ population, both in peripheral blood and in the tumor microenvironment, have depressed function. Innate helper T cells (CD4+) display weak proliferative responses and dramatically lowered synthesis of the T H 1 cytokine IL-2.
The degree of infiltrating lymphocytes found within gliomas has been found to correlate with patient survival. However, other studies have failed to reproduce this finding. Despite the presence of large numbers of glioma-infiltrating lymphocytes, the function and activity of these lymphocytes is difficult to predict. In fact, Hussain and Heimberger demonstrated that the vast majority of CD8+ T cells found within gliomas are not activated. One hypothesis states that these are actually naïve T cells passively infiltrating the tumor across a compromised BBB caused by the tumor; alternatively, they may represent a population that once was active but has been subsequently rendered inactive by the host of immunosuppressive mechanisms found in the tumor microenvironment.
In patients with malignant glioma, a subpopulation of T lymphocytes termed T-regulatory cells (CD4+CD25+ cells; T regs ), which suppresses activity of effector T cells, is increased. By downregulating the production of key cytokines, such as IL-2 and interferon (IFN)-γ from target lymphocytes, they potently inhibit T-cell activation, proliferation, and differentiation. T regs also induce lymphocytes to secrete predominantly T H 2 cytokines, such as IL-10, and TGF-β2, which in turn continues to propagate the regulatory phenotypes of T lymphocytes. In vivo experiments have demonstrated significantly improved survival after depleting T regs in brains of mice harboring GL261 gliomas by injecting an antibody targeting against T regs (anti-CD25+ mAb).
Secretion of various immune inhibitory cytokines and molecules by glioma cells also plays a large role in glioma-associated immunosuppression. IL-10 is also selectively secreted by invasive glioma. Malignant glioma cells produces large amounts of prostaglandin E2, which in turn inhibits IL-2 activation of lymphocytes. High levels of expression of TGF-β2 are present in malignant glioma. TGF-β2 mRNA is present in samples of human glioblastoma but not in normal adult brain. TGF-β2 has previously been referred to as glioblastoma cell-derived T-cell suppressor factor (G-TsF) because of its potent immunosuppressive activity, particularly in inhibition of cytotoxic T cells. In addition, inhibition of signaling through the TGF-β2 pathway by antisense RNA in the C6 rat glioma model significantly prolonged survival, and at times eradicated the tumor entirely. These experiments are strongly supportive of the key role TGF-β2 plays in the immunosuppression that seems essential for the survival of glioblastoma cells.
In addition to the secreted immunosuppressive factors, expression of molecules that induce apoptosis of immune effectors, such as Fas ligand, galectin-1, and B7-H1, contribute to the immunosuppressive activity of gliomas. Fas ligand (FasL, CD95L) and its receptor Fas (CD95) are important mediators of apoptosis in the immune system, particularly of CD8+ cytotoxic lymphocytes. High expression of FasL by human glioma cells naturally has been found to be associated with low levels of T-cell infiltration, suggesting that FasL expression by tumor cells may contribute to T-cell depletion in tumors by increased T-cell apoptosis. However, the clinical significance of levels of FasL expression remains to be determined. Galectin-1 induces apoptosis in a variety of immune cell types through an alternative signaling pathway from Fas/FasL. Overexpression of galectin-1 by gliomas likely also contributes to increased apoptosis of T cells by gliomas, serving as another method of evasion from antitumor activity of T lymphocytes. Higher levels of expression of galectin are found in higher grade astrocytomas. B7-H1 is a potent immunosuppressive surface molecule that induces T-cell apoptosis by the PD-1 signaling pathway. A subset of gliomas with particularly strong immunoresistant phenotypes has high levels of B7-H1 expression on the surface. In addition to the expression of apoptotic molecules targeted at T lymphocytes on glioma cells, microglia in presence of glioma have elevated levels of FasL and B7-H1.
Iatrogenic factors may cause a systemic immunosuppression in patients with glioma. Corticosteroids given for tumor-associated edema may cause inhibition of cytokine production and sequestration of CD4+ T cells. Recent evidence suggests, however, that at therapeutic doses corticosteroids may not interfere with immunotherapy. Chemotherapeutic agents, such as temozolomide, for example, can cause lymphopenia, particularly of the CD4+ population, which may compound immunotherapeutic modalities that depend on CD4+ T cell response. Other agents such as rapamycin inhibit production of the proliferative cytokine IL-2.
Immunotherapy
Challenges in management of malignant gliomas have been rooted in inevitable recurrences of the tumor despite aggressive therapy. This challenge highlights the diffusely infiltrative nature of the tumor with evidence of microscopic disease that has already spread diffusely beyond the tumor mass at the time of clinical presentation.
The development of a successful mode of therapy requires systemic efficacy throughout the brain, with the ability to target tumor cells left behind after surgical resection and conventional adjuvant therapies. Such systemic therapy must also be highly specific for infiltrating tumor cells. Immunotherapy represents an attractive modality, with the potential to harness the potency, specificity, and memory of the immune system against infiltrating glioma cells.
Strategies in antiglioma immunotherapy broadly include cytokine therapy, passive immunotherapy, and active immunotherapy. Cytokine therapy is based on the concept that administration of immunomodulatory cytokines will activate the immune system. Passive immunotherapy includes serotherapy, in which monoclonal antibodies are given to aid immune recognition of tumor and deliver toxins to tumor cells, and adoptive therapy, which involves tumor-specific immune cells that are expanded ex vivo and reintroduced to the patient. Active immunotherapy involves generating or augmenting the patient’s own immune response to tumor antigens, typically by administering tumor antigens or professional APCs.
Cytokine Therapy
Cytokines have a potent capacity for immunomodulation, and immunotherapy with cytokines has been applied in oncology against a variety of tumors with variable success. In vitro experiments have established the efficacy of cytokines in eliciting antitumor activity of immune effector cells. Different strategies for delivery of cytokines to the CNS have been explored, including injection/infusion of recombinant cytokines, vectors containing cytokine encoding genes, cells that secrete cytokines, or cytokines linked to toxins.
The first clinical trial using cytokine immunotherapy employed intratumoral IFN-α in addition to surgery and radiotherapy, and compared the trial group with a group who received surgical resection, radiation, and chemotherapy. Although the investigators reported encouraging results, conclusions drawn regarding efficacy of IFN-α were limited due to flaws in study design. Farkkila and colleagues published the results of their trial using IFN-γ neoadjuvant and adjuvant to radiotherapy. Although the therapeutic regimen was well tolerated, there was no statistically significant difference in overall survival between the trial and control groups. Studies that followed using systemic or intrathecal administration of IFN-α, IFN-γ, or IL-2 continued to find no significant improvement in survival while patients in the treatment arm encountered considerable toxicities. Current approaches are looking to targeted delivery of cytokines to reduce systemic toxicity and increase the effective cytokine concentrations within the tumor.
Viral vectors have been used for local delivery of cytokines, with evidence of glioma-specific responses. Strategies using intratumoral implantation of various cell types genetically modified to produce cytokines have produced more encouraging results. Injection of IL-2–secreting allogeneic fibroblasts into GL261 tumors in mice significantly delayed tumor development when injected before the tumor cells, and prolonged survival in mice with established tumors. Neural stem/progenitor cells have many qualities that make them attractive candidates for carriers for delivery of cytokines. These cells self replicate, have prolonged survival, and migrate further than do viral vectors. Injection of neural stem/progenitor cells transfected to produce IL-2, IL-4, IL-12, and IL-23 into animals with established gliomas improved survival.
Alternative vehicles for intratumoral injection include liposomes and biopolymer microspheres. Injection of liposomes containing a plasmid with the IFN-β gene into GL261 gliomas in mice induced a robust activation of natural killer cells, IFN-β expression by tumor cells, significant infiltration of cytotoxic T cells, a 16-fold reduction in mean tumor volume, and a complete response in 40% of animals. These effects were much more robust than those seen with exogenous administration of IFN-β. These infiltrative T cells as well as systemic T cells were isolated from treated mice, and were shown to have specific antitumor responses. Biopolymer microspheres containing IL-2 are also effective in generating a specific response when injected into tumors of mice and rats.
Cytokines have also been used to deliver toxins such as Pseudomonas exotoxin. Malignant glioma cells and medulloblastoma cells express high levels of the IL-4 receptor (IL-4R) on their surface not found on normal brain cells. This overexpression has been used for selective delivery of toxins. Cytotoxic effects of Pseudomonas exotoxin conjugated to IL-4 against glioma and medulloblastoma cells have been demonstrated in culture. In a clinical trial of intratumoral injection of IL-4–pseudomonas exotoxin for patients with high-grade glioma, 6 of 9 patients enrolled showed evidence of tumor necrosis, and there was no significant toxicity found in any patient. Current clinical trials are continuing to evaluate this modality’s efficacy and maximum tolerated dose.
IL-13R is similarly overexpressed in malignant glioma, but not in normal brain cells. IL-13 conjugated to Pseudomonas exotoxin also is well tolerated when given intratumorally for patients with recurrent, malignant glioma. Recent efforts have focused on optimizing the specificity and strength of the interaction between IL-13 and IL-13R of glioma cells, as well as novel modes of delivery of the interleukin-toxin. Pseudomonas exotoxin conjugated to TGF-α has also resulted in improvement in survival of mice bearing tumor xenografts, with greater improvements seen in mice that express the epidermal growth factor receptor (EGFR). In a phase 1 trial, 2 patients received intratumoral infusions of TGF-α conjugated to the Pseudomonas exotoxin, and showed radiographic response with relative safety.
Overall, immunotherapy with cytokines has demonstrated relative safety, with variable efficacy. Thus, given the relative nonspecificity of cytokine therapy, it may ultimately prove most useful as an adjunct to other types of therapies, and potential use of cytokines as adjunct to chemotherapy, termed chemoimmunotherapy, is an active area of development.
Passive Immunotherapy
Serotherapy
Passive immunotherapy includes serotherapy and adoptive immunotherapy. Serotherapy uses monoclonal antibodies to effect an antitumor response or to achieve very specific delivery of toxins, chemotherapy, or radiotherapy to the tumor cells. An important determinant of its success is the identification of “tumor antigens,” specific antigens expressed on tumor cell surfaces but not on normal brain parenchyma. In human gliomas, antigens of interest have been those with quantitative differences between glioma and normal cells. Targeted glioma antigens have included tenascin, EGFR and its mutated form EGFRvIII, chondroitin sulfate, vascular endothelial growth factor (VEGF) receptor, neural cell adhesion molecule (NCAM), and hepatocyte growth factor/scatter factor.
Tenascin is an extracellular matrix protein that is strongly expressed in gliomas but not in normal brain. Tenascin is readily identified immunohistochemically by monoclonal antibody (mAb) 81C6. Systemic administration of 131 I-conjugated 81C6 mAb to mice with human glioblastoma xenografts prolonged survival, with evidence of localization of the radioisotope to the tumor. Clinical trials of 131 I-conjugated 81C6 mAb given intrathecally have shown relative safety of the technique at low radiation doses, with dose-limiting neurotoxicity and hematologic toxicity at higher dose. Several phase 1 and 2 trials studying 131 I-conjugated 81C6 mAb injected into the surgical resection cavity in humans with glioblastoma have shown improved survival in patients with malignant gliomas relative to those receiving traditional therapies.
Similar to tenascin, EGFR is specifically overexpressed by glioma cells, and signaling through EGFR is believed to play a key role in survival, proliferation, and progression of gliomas. In a clinical trial by Kalofonos and colleagues, patients with high-grade gliomas with evidence of localization of markers linked to anti-EGFR mAb to tumor radiographically were treated with 131 I-conjugated mAb to EGFR injected intravenously or infused into the internal carotid artery. Six of 10 treated patients showed clinical response lasting 6 months to 3 years, with no major toxicity. In another trial, increasing doses of murine anti-EGFR mAb, EMD55900 (mAb 425) was given in a single intravenous injection to 30 patients with malignant gliomas. In 73% of patients, there was evidence of significant binding of EMD55900 to the tumor. Similar binding was observed by Crombet and colleagues. In a phase 1/2 study in which patients were given repeated infusions of EMD55900, toxicity was minimal, but no significant therapeutic benefit was found, as 46% of patients had progressed at 3 months. Yet another trial using EMD55900 was stopped due to high levels of toxicity of inflammatory reactions. Another phase 1/2 trial used a humanized anti-EGFR antibody, h-R3, designed to inhibit the kinase activity of the receptor for patients with malignant glioma, and demonstrated no high-grade toxicity and overall 37.9% response rate with stable disease in 41.4% patients at median follow-up of 29 months. Several other phase 1, 2, and 3 trials have been conducted using EMD55900 conjugated to 125 I. These trials have demonstrated that the conjugated mAb effectively localizes to the GBM and is well tolerated. A phase 2 trial in which patients received radiolabeled mAb following standard surgical resection and radiation therapy demonstrated a median survival of 15.6 months. A similar study reported median survival of 13.5 months. Phase 3 trials are currently ongoing.
EGFRvIII is a constitutively active mutant form of EGFR, and as a tumor antigen that likely has a large role in tumorigenicity, is an attractive target for serotherapy. Y10, a mAb developed against a murine homologue of EGFRvIII, can generate antibody-dependent cell-mediated cytotoxicity in vitro. In vivo, a single dose of Y10, injected intratumorally into an intracranial murine B16 melanoma transfected to produce EGFRvIII, prolonged survival by 286%. This mechanism of this effect was Fc receptor-dependent. Systemic injections of the anti-EGFRvIII mAb 806 into mice with U87 glioma xenografts significantly reduced tumor volume and increased survival.
Antibodies can provide specific targeted delivery of chemotherapeutics or toxins as well. Mamot and colleagues used fragments of mAbs binding both EGFR and EGFRvIII conjugated to immunoliposomes containing the cytotoxic drugs doxorubicin, vinorelbine, and methotrexate, and observed delivery of these drugs to glioblastoma cells in vitro. These investigators observed intracellular accumulation in glioma cells of the drug at high rates, beginning at 15 minutes. The toxicity to the tumor cells was also higher when immunoliposomal formulation was given, compared with the same drugs given without the liposomes. The same group followed this study with experiments in xenograft models, showing superior efficacy in slowing tumor growth of EGFR-targeted immunoliposomes containing cetuximab. Antibodies have also been conjugated to several different toxins, with varying results. The specificity of delivery of therapeutic agents by mAb to tumor-specific antigens holds great potential for limiting therapeutic toxicity in immunotherapy against gliomas.
Adoptive immunotherapy
Adoptive immunotherapy augments the antitumor response with the reintroduction of immune effector cells that have been isolated from patients and expanded ex vivo. Expansion of cells ex vivo permits amplification under controlled conditions isolated from the immunosuppressive tumor microenvironment. Most adoptive immunotherapeutic strategies have used harvested lymphocytes stimulated with IL-2, without the presence of specific antigens. This process creates lymphokine-activated killer cells (LAKs). Others have used tumor-infiltrating lymphocytes, neural stem cells (discussed earlier), tumor-draining lymph node T cells, and non-MHC-restricted, cytotoxic T-cell leukemic cell lines.
Jacobs and colleagues reported the first clinical trial studying immunotherapy with LAKs. LAKs and IL-2 were infused directly into the tumor bed of patients with malignant glioma, with minimal toxicity, and mean progression-free survival in this small cohort was 25 weeks.
Other trials using this technique found a small benefit in patient survival, but demonstrated dose-limiting neural toxicity related to cerebral edema, due to IL-2. A recent study by Dillman and colleagues reported a median survival of 17.5 months in glioblastoma patients who had LAKs placed in the resection cavity compared with 13.6 months in controls. In mouse models, LAKs coated with a bispecific anti-CD3 and antiglioma antibodies increased the LAK activity of peripheral blood lymphocytes against the xenograft gliomas. The application of these coated LAKs in clinical trials showed promising results. Infusion of coated LAKs into the tumor bed in human malignant gliomas resulted in either partial or complete regression tumor radiographically in 8 of 10 patients. None of the 10 patients suffered tumor recurrence during the 10- to 18-month follow-up, and 9 of the 10 control patients given untreated LAK cells developed a recurrent tumor within 1 year.
Tumor-infiltrating lymphocytes (TILs) found within glioma tissue contain a higher proportion of cytotoxic (CD8+) T cells compared with the composition of the peripheral blood lymphocyte population. Presuming their recognition and activity to one or more tumor antigens, combined with the fact that they are readily expanded in culture, has made TILs potential candidates for adoptive immunotherapy. In vitro, when compared with LAKs, TILs are much more cytotoxic to glioma cells.
Saris and colleagues studied the antitumor activity of these TILs in a murine glioma model (GL261). TILs were incubated with enzymatically digested GL261 cells and IL-2, and then infused intraperitoneally into mice harboring gliomas in the liver or brain. The infusion reduced the number of liver metastases relative to that in animals receiving saline or IL-2 alone, but did not lengthen the survival of animals with GL261 tumors in the brain, leading the investigators to conclude that the inefficacy of TIL therapy in the brain reflects the unique challenges of the immunosuppressive tumor microenvironment, and that more efficient delivery systems need to be evaluated. Subsequent studies, however, have reported success with TIL in treatment of intracranial gliomas in vitro. Several clinical pilot studies have described the feasibility of reinfusion of IL-2 and autologous TILs expanded in vitro to patients systemically and locally, with little toxicity. Evidence for efficacy of such technique is currently lacking. However, TILs display many dysfunctional functions that contribute to the failure to generate an effective antitumor response in vivo: altered cellular signaling, decreased proliferation, defective cytokine secretion, decreased cytotoxic capacity, and a predisposition toward apoptosis. Despite their defects, the superior specificity of TILs over LAKs and early clinical success with TIL strategies warrant further investigation.
Plautz and colleagues employed a novel technique using the site of tumor-rich vaccine injection. In a phase 1 clinical trial, 12 patients with astrocytoma, anaplastic glioma, or GBM were initially were given these T lymphocytes from injection site–draining lymph nodes after activation and expansion ex vivo. Partial regression was observed in 4 patients, and no long-term toxicity was seen during the 2-year follow-up period.
The use of MHC nonrestricted, cytotoxic T-cell lines has also been explored. TALL-104, one such clone, was established from a patient with acute T-lymphoblastic leukemia, and is able to distinguish between tumor and normal cells with MHC nonrestricted tumoricidal activity. Transfer of TALL-104 cells into tumor sites of U87 xenografts in mice significantly reduced tumor growth and prolonged survival, by the dual mechanisms of direct tumoricidal action and recruitment of endogenous antitumor activity.
Geoerger and colleagues subsequently showed evidence of significant cytotoxic activity of TALL-104 cells against several human glioblastoma and medulloblastoma cell lines in rat models, and stressed the importance of local, as opposed to systemic, administration of TALL-104 cells. Preclinical studies have characterized the cytotoxic activity, trafficking patterns, viability of TALL-104 cells under different conditions, and activity against brain tumor versus normal brain cells have concluded that TALL-104 cells are appropriate for human clinical trials. TALL-104 implantation therapy shows killing of glioma cells, but not of normal brain cells, through a mechanism mediated by specific cytokine release, and their activity is not altered by presence of radiotherapy or corticosteroids. Recent studies have further elucidated the wide array of mechanisms of cytotoxic activity against tumor cells by TALL-104 cells.
Unfortunately, expression of tumor antigens, such as EGFRvIII, is not uniform among cells of a given tumor. Thus, adoptive immunotherapy targeting a single tumor-specific antigen can be compounded by variants in the target, allowing evasion from immune-mediated killing. This lack of efficacy has been seen with adoptive T cell therapy directed at melanoma-specific antigens, with the result of preferential survival of tumors with antigenic variants. Therefore, it is likely that the most successful adoptive cell transfer therapies will target multiple tumor antigens. Adoptive immunotherapy, like cytokine therapy, although not fully effective by itself, may become an important adjuvant to standard treatments and other immunotherapies for primary brain tumors.
Active Immunotherapy
Active immunotherapy involves priming or augmenting patients’ immunity in vivo by vaccination against tumor antigen. Tumor vaccines for malignant glioma have been the focus of great interest in recent years. Successful development of glioma vaccines, however, requires proper presentation of tumor antigens and induction of effective, durable antigen-specific T cell immune response. Early efforts in active immunotherapy used vaccines containing autologous tumor cells as a source of tumor antigens, given with various cytokines for immune stimulation. Despite evidence of safety and feasibility of such techniques, challenges remain due to innate poor antigen-presenting capacity of tumor cells, with low levels of expressed costimulatory molecules.
To augment the process of antigen presentation, professional APCs have also been used in glioma vaccines. Recent interest has turned to dendritic cells (DCs), which have an abundant expression of costimulatory molecules and have great capacity for activating T lymphocytes. DCs are exposed to tumor antigens, then used to initiate an antitumor response in the patient’s endogenous T cells. More specifically, autologous DCs are obtained from peripheral blood mononuclear cells or bone marrow, primed to maturation, exposed to whole tumor cell lysate or tumor antigen ex vivo, and reintroduced to the patient. In vitro experiments have established the ability of DCs exposed to tumor antigens in inducing T cell proliferation and generating cytotoxic responses.
In clinical trials, DCs have been exposed to tumor antigens in a variety of ways, including whole tumor cells, isolated peptides, tumor lysates, or tumor RNA. An early phase 1 trial used peptide-pulsed DCs isolated from peripheral blood and showed the generation of robust T cell infiltration into the tumor. Early efforts by Kikuchi and colleagues employed DCs fused to glioma cells and injected intradermally for patients with malignant gliomas. No adverse reactions, but a partial response in only 2 of 8 patients, was observed. In a subsequent study by these investigators, IL-12 was added to the formulation and more robust response was seen: there was a 50% radiographic reduction of the tumor in 4 of 15 patients, with similar safety profiles. A complete regression of glioma was achieved in the murine GL261 model when a regimen of intrasplenic vaccination with DC/tumor fused cells, local cranial radiotherapy, and anti-CD134 mAb 7 was given. Other strategies of DC exposure to antigens have involved using tumor lysates. Reintroduction of DCs preloaded with total tumor RNA rather than tumor antigen also produced a strong cytotoxic T cell response against autologous glioma cells. These early clinical trials have yielded encouraging results regarding generating responses and improving survival in patients. Liau and colleagues proposed that the most promising patient subgroup for DC vaccine therapy may be patients with small, quiescent tumors with low expression of tumor TGF-β. The phase 2 randomized trial using tumor lysate pulsed DC vaccine is ongoing.
The use of unselected tumor extracts to prime DCs in such nonspecific approaches risks inducing autoimmunity against antigens of normal brain. In efforts to avoid this potential hazard, focus has turned to more specific approaches using tumor-specific antigens, such as EGFRvIII as targets for glioma vaccines. Preliminary studies using EGFRvIII peptide-pulsed DCs showed generation of cytotoxic activity against the U87 human glioma cell line. When the same vaccine was given to mice that subsequently received intracerebral injections of syngeneic melanoma expressing the murine homologue of EGFRvIII, animals that were immunized survived 6 times as long as those that were not immunized. Among immunized mice, 63% survived long term and 100% survived rechallenge with melanoma, indicating evidence of immunologic memory against the tumor.
A peptide-based vaccine targeting the tumor-specific mutated portion of EGFRvIII is also under investigation. A phase 1 trial with enrollment of 19 patients has been completed. The therapy was shown to be well tolerated, and treated patients had progression-free survival of 12 months and median overall survival of 18 months. Of note, recurrent tumors after the vaccine showed no expression of EGFRvIII. The phase 2/3 randomized trial of the EGFRvIII peptide vaccine with radiation and temozolomide is currently ongoing.
Another peptide-based vaccine currently under study is based on heat-shock protein gp96 and its associated peptides isolated from the patient’s autologous tumor acquired at time of surgery. Preliminary results of the ongoing phase 1/2 trial have demonstrated the vaccine to be well tolerated, with evidence of induction of tumor-specific responses.
Alternative active immunotherapeutic approaches have aimed at initiating an immune response to tumor in vivo through lymphocytes. In one such study, peripheral lymphocytes removed from patients with high-grade gliomas were cultured with irradiated tumor cells in the presence of bacillus Calmette-Guérin and IL-2, then systemically reinfused into patients, with minimal toxicity. Two of 9 patients experienced increased survival with evidence of tumor regression, 1 had tumor regression but not increased survival, and the remaining 6 had neither.
Infectious agents have also been used to induce an antigen-specific immune response to gliomas. These vaccines contain viral or bacterial vectors carrying tumor antigen genes, and the concept involves that an immune response to the highly immunogenic infectious agent should augment the response to the tumor antigen as well. Such an approach in animal models using Listeria monocytogenes have shown efficacy against extracranial but not intracranial tumor, suggesting potential for improved efficacy in gliomas with improved delivery systems to the CNS. In a phase 1 trial, recombinantly modified vaccinia virus Ankara carrying the cDNA for a melanoma antigen has proven safe, and capable of eliciting a strong response against the virus but not against the antigen.
Immunotherapy
Challenges in management of malignant gliomas have been rooted in inevitable recurrences of the tumor despite aggressive therapy. This challenge highlights the diffusely infiltrative nature of the tumor with evidence of microscopic disease that has already spread diffusely beyond the tumor mass at the time of clinical presentation.
The development of a successful mode of therapy requires systemic efficacy throughout the brain, with the ability to target tumor cells left behind after surgical resection and conventional adjuvant therapies. Such systemic therapy must also be highly specific for infiltrating tumor cells. Immunotherapy represents an attractive modality, with the potential to harness the potency, specificity, and memory of the immune system against infiltrating glioma cells.
Strategies in antiglioma immunotherapy broadly include cytokine therapy, passive immunotherapy, and active immunotherapy. Cytokine therapy is based on the concept that administration of immunomodulatory cytokines will activate the immune system. Passive immunotherapy includes serotherapy, in which monoclonal antibodies are given to aid immune recognition of tumor and deliver toxins to tumor cells, and adoptive therapy, which involves tumor-specific immune cells that are expanded ex vivo and reintroduced to the patient. Active immunotherapy involves generating or augmenting the patient’s own immune response to tumor antigens, typically by administering tumor antigens or professional APCs.
Cytokine Therapy
Cytokines have a potent capacity for immunomodulation, and immunotherapy with cytokines has been applied in oncology against a variety of tumors with variable success. In vitro experiments have established the efficacy of cytokines in eliciting antitumor activity of immune effector cells. Different strategies for delivery of cytokines to the CNS have been explored, including injection/infusion of recombinant cytokines, vectors containing cytokine encoding genes, cells that secrete cytokines, or cytokines linked to toxins.
The first clinical trial using cytokine immunotherapy employed intratumoral IFN-α in addition to surgery and radiotherapy, and compared the trial group with a group who received surgical resection, radiation, and chemotherapy. Although the investigators reported encouraging results, conclusions drawn regarding efficacy of IFN-α were limited due to flaws in study design. Farkkila and colleagues published the results of their trial using IFN-γ neoadjuvant and adjuvant to radiotherapy. Although the therapeutic regimen was well tolerated, there was no statistically significant difference in overall survival between the trial and control groups. Studies that followed using systemic or intrathecal administration of IFN-α, IFN-γ, or IL-2 continued to find no significant improvement in survival while patients in the treatment arm encountered considerable toxicities. Current approaches are looking to targeted delivery of cytokines to reduce systemic toxicity and increase the effective cytokine concentrations within the tumor.
Viral vectors have been used for local delivery of cytokines, with evidence of glioma-specific responses. Strategies using intratumoral implantation of various cell types genetically modified to produce cytokines have produced more encouraging results. Injection of IL-2–secreting allogeneic fibroblasts into GL261 tumors in mice significantly delayed tumor development when injected before the tumor cells, and prolonged survival in mice with established tumors. Neural stem/progenitor cells have many qualities that make them attractive candidates for carriers for delivery of cytokines. These cells self replicate, have prolonged survival, and migrate further than do viral vectors. Injection of neural stem/progenitor cells transfected to produce IL-2, IL-4, IL-12, and IL-23 into animals with established gliomas improved survival.
Alternative vehicles for intratumoral injection include liposomes and biopolymer microspheres. Injection of liposomes containing a plasmid with the IFN-β gene into GL261 gliomas in mice induced a robust activation of natural killer cells, IFN-β expression by tumor cells, significant infiltration of cytotoxic T cells, a 16-fold reduction in mean tumor volume, and a complete response in 40% of animals. These effects were much more robust than those seen with exogenous administration of IFN-β. These infiltrative T cells as well as systemic T cells were isolated from treated mice, and were shown to have specific antitumor responses. Biopolymer microspheres containing IL-2 are also effective in generating a specific response when injected into tumors of mice and rats.
Cytokines have also been used to deliver toxins such as Pseudomonas exotoxin. Malignant glioma cells and medulloblastoma cells express high levels of the IL-4 receptor (IL-4R) on their surface not found on normal brain cells. This overexpression has been used for selective delivery of toxins. Cytotoxic effects of Pseudomonas exotoxin conjugated to IL-4 against glioma and medulloblastoma cells have been demonstrated in culture. In a clinical trial of intratumoral injection of IL-4–pseudomonas exotoxin for patients with high-grade glioma, 6 of 9 patients enrolled showed evidence of tumor necrosis, and there was no significant toxicity found in any patient. Current clinical trials are continuing to evaluate this modality’s efficacy and maximum tolerated dose.
IL-13R is similarly overexpressed in malignant glioma, but not in normal brain cells. IL-13 conjugated to Pseudomonas exotoxin also is well tolerated when given intratumorally for patients with recurrent, malignant glioma. Recent efforts have focused on optimizing the specificity and strength of the interaction between IL-13 and IL-13R of glioma cells, as well as novel modes of delivery of the interleukin-toxin. Pseudomonas exotoxin conjugated to TGF-α has also resulted in improvement in survival of mice bearing tumor xenografts, with greater improvements seen in mice that express the epidermal growth factor receptor (EGFR). In a phase 1 trial, 2 patients received intratumoral infusions of TGF-α conjugated to the Pseudomonas exotoxin, and showed radiographic response with relative safety.
Overall, immunotherapy with cytokines has demonstrated relative safety, with variable efficacy. Thus, given the relative nonspecificity of cytokine therapy, it may ultimately prove most useful as an adjunct to other types of therapies, and potential use of cytokines as adjunct to chemotherapy, termed chemoimmunotherapy, is an active area of development.
Passive Immunotherapy
Serotherapy
Passive immunotherapy includes serotherapy and adoptive immunotherapy. Serotherapy uses monoclonal antibodies to effect an antitumor response or to achieve very specific delivery of toxins, chemotherapy, or radiotherapy to the tumor cells. An important determinant of its success is the identification of “tumor antigens,” specific antigens expressed on tumor cell surfaces but not on normal brain parenchyma. In human gliomas, antigens of interest have been those with quantitative differences between glioma and normal cells. Targeted glioma antigens have included tenascin, EGFR and its mutated form EGFRvIII, chondroitin sulfate, vascular endothelial growth factor (VEGF) receptor, neural cell adhesion molecule (NCAM), and hepatocyte growth factor/scatter factor.
Tenascin is an extracellular matrix protein that is strongly expressed in gliomas but not in normal brain. Tenascin is readily identified immunohistochemically by monoclonal antibody (mAb) 81C6. Systemic administration of 131 I-conjugated 81C6 mAb to mice with human glioblastoma xenografts prolonged survival, with evidence of localization of the radioisotope to the tumor. Clinical trials of 131 I-conjugated 81C6 mAb given intrathecally have shown relative safety of the technique at low radiation doses, with dose-limiting neurotoxicity and hematologic toxicity at higher dose. Several phase 1 and 2 trials studying 131 I-conjugated 81C6 mAb injected into the surgical resection cavity in humans with glioblastoma have shown improved survival in patients with malignant gliomas relative to those receiving traditional therapies.
Similar to tenascin, EGFR is specifically overexpressed by glioma cells, and signaling through EGFR is believed to play a key role in survival, proliferation, and progression of gliomas. In a clinical trial by Kalofonos and colleagues, patients with high-grade gliomas with evidence of localization of markers linked to anti-EGFR mAb to tumor radiographically were treated with 131 I-conjugated mAb to EGFR injected intravenously or infused into the internal carotid artery. Six of 10 treated patients showed clinical response lasting 6 months to 3 years, with no major toxicity. In another trial, increasing doses of murine anti-EGFR mAb, EMD55900 (mAb 425) was given in a single intravenous injection to 30 patients with malignant gliomas. In 73% of patients, there was evidence of significant binding of EMD55900 to the tumor. Similar binding was observed by Crombet and colleagues. In a phase 1/2 study in which patients were given repeated infusions of EMD55900, toxicity was minimal, but no significant therapeutic benefit was found, as 46% of patients had progressed at 3 months. Yet another trial using EMD55900 was stopped due to high levels of toxicity of inflammatory reactions. Another phase 1/2 trial used a humanized anti-EGFR antibody, h-R3, designed to inhibit the kinase activity of the receptor for patients with malignant glioma, and demonstrated no high-grade toxicity and overall 37.9% response rate with stable disease in 41.4% patients at median follow-up of 29 months. Several other phase 1, 2, and 3 trials have been conducted using EMD55900 conjugated to 125 I. These trials have demonstrated that the conjugated mAb effectively localizes to the GBM and is well tolerated. A phase 2 trial in which patients received radiolabeled mAb following standard surgical resection and radiation therapy demonstrated a median survival of 15.6 months. A similar study reported median survival of 13.5 months. Phase 3 trials are currently ongoing.
EGFRvIII is a constitutively active mutant form of EGFR, and as a tumor antigen that likely has a large role in tumorigenicity, is an attractive target for serotherapy. Y10, a mAb developed against a murine homologue of EGFRvIII, can generate antibody-dependent cell-mediated cytotoxicity in vitro. In vivo, a single dose of Y10, injected intratumorally into an intracranial murine B16 melanoma transfected to produce EGFRvIII, prolonged survival by 286%. This mechanism of this effect was Fc receptor-dependent. Systemic injections of the anti-EGFRvIII mAb 806 into mice with U87 glioma xenografts significantly reduced tumor volume and increased survival.
Antibodies can provide specific targeted delivery of chemotherapeutics or toxins as well. Mamot and colleagues used fragments of mAbs binding both EGFR and EGFRvIII conjugated to immunoliposomes containing the cytotoxic drugs doxorubicin, vinorelbine, and methotrexate, and observed delivery of these drugs to glioblastoma cells in vitro. These investigators observed intracellular accumulation in glioma cells of the drug at high rates, beginning at 15 minutes. The toxicity to the tumor cells was also higher when immunoliposomal formulation was given, compared with the same drugs given without the liposomes. The same group followed this study with experiments in xenograft models, showing superior efficacy in slowing tumor growth of EGFR-targeted immunoliposomes containing cetuximab. Antibodies have also been conjugated to several different toxins, with varying results. The specificity of delivery of therapeutic agents by mAb to tumor-specific antigens holds great potential for limiting therapeutic toxicity in immunotherapy against gliomas.
Adoptive immunotherapy
Adoptive immunotherapy augments the antitumor response with the reintroduction of immune effector cells that have been isolated from patients and expanded ex vivo. Expansion of cells ex vivo permits amplification under controlled conditions isolated from the immunosuppressive tumor microenvironment. Most adoptive immunotherapeutic strategies have used harvested lymphocytes stimulated with IL-2, without the presence of specific antigens. This process creates lymphokine-activated killer cells (LAKs). Others have used tumor-infiltrating lymphocytes, neural stem cells (discussed earlier), tumor-draining lymph node T cells, and non-MHC-restricted, cytotoxic T-cell leukemic cell lines.
Jacobs and colleagues reported the first clinical trial studying immunotherapy with LAKs. LAKs and IL-2 were infused directly into the tumor bed of patients with malignant glioma, with minimal toxicity, and mean progression-free survival in this small cohort was 25 weeks.
Other trials using this technique found a small benefit in patient survival, but demonstrated dose-limiting neural toxicity related to cerebral edema, due to IL-2. A recent study by Dillman and colleagues reported a median survival of 17.5 months in glioblastoma patients who had LAKs placed in the resection cavity compared with 13.6 months in controls. In mouse models, LAKs coated with a bispecific anti-CD3 and antiglioma antibodies increased the LAK activity of peripheral blood lymphocytes against the xenograft gliomas. The application of these coated LAKs in clinical trials showed promising results. Infusion of coated LAKs into the tumor bed in human malignant gliomas resulted in either partial or complete regression tumor radiographically in 8 of 10 patients. None of the 10 patients suffered tumor recurrence during the 10- to 18-month follow-up, and 9 of the 10 control patients given untreated LAK cells developed a recurrent tumor within 1 year.
Tumor-infiltrating lymphocytes (TILs) found within glioma tissue contain a higher proportion of cytotoxic (CD8+) T cells compared with the composition of the peripheral blood lymphocyte population. Presuming their recognition and activity to one or more tumor antigens, combined with the fact that they are readily expanded in culture, has made TILs potential candidates for adoptive immunotherapy. In vitro, when compared with LAKs, TILs are much more cytotoxic to glioma cells.
Saris and colleagues studied the antitumor activity of these TILs in a murine glioma model (GL261). TILs were incubated with enzymatically digested GL261 cells and IL-2, and then infused intraperitoneally into mice harboring gliomas in the liver or brain. The infusion reduced the number of liver metastases relative to that in animals receiving saline or IL-2 alone, but did not lengthen the survival of animals with GL261 tumors in the brain, leading the investigators to conclude that the inefficacy of TIL therapy in the brain reflects the unique challenges of the immunosuppressive tumor microenvironment, and that more efficient delivery systems need to be evaluated. Subsequent studies, however, have reported success with TIL in treatment of intracranial gliomas in vitro. Several clinical pilot studies have described the feasibility of reinfusion of IL-2 and autologous TILs expanded in vitro to patients systemically and locally, with little toxicity. Evidence for efficacy of such technique is currently lacking. However, TILs display many dysfunctional functions that contribute to the failure to generate an effective antitumor response in vivo: altered cellular signaling, decreased proliferation, defective cytokine secretion, decreased cytotoxic capacity, and a predisposition toward apoptosis. Despite their defects, the superior specificity of TILs over LAKs and early clinical success with TIL strategies warrant further investigation.
Plautz and colleagues employed a novel technique using the site of tumor-rich vaccine injection. In a phase 1 clinical trial, 12 patients with astrocytoma, anaplastic glioma, or GBM were initially were given these T lymphocytes from injection site–draining lymph nodes after activation and expansion ex vivo. Partial regression was observed in 4 patients, and no long-term toxicity was seen during the 2-year follow-up period.
The use of MHC nonrestricted, cytotoxic T-cell lines has also been explored. TALL-104, one such clone, was established from a patient with acute T-lymphoblastic leukemia, and is able to distinguish between tumor and normal cells with MHC nonrestricted tumoricidal activity. Transfer of TALL-104 cells into tumor sites of U87 xenografts in mice significantly reduced tumor growth and prolonged survival, by the dual mechanisms of direct tumoricidal action and recruitment of endogenous antitumor activity.
Geoerger and colleagues subsequently showed evidence of significant cytotoxic activity of TALL-104 cells against several human glioblastoma and medulloblastoma cell lines in rat models, and stressed the importance of local, as opposed to systemic, administration of TALL-104 cells. Preclinical studies have characterized the cytotoxic activity, trafficking patterns, viability of TALL-104 cells under different conditions, and activity against brain tumor versus normal brain cells have concluded that TALL-104 cells are appropriate for human clinical trials. TALL-104 implantation therapy shows killing of glioma cells, but not of normal brain cells, through a mechanism mediated by specific cytokine release, and their activity is not altered by presence of radiotherapy or corticosteroids. Recent studies have further elucidated the wide array of mechanisms of cytotoxic activity against tumor cells by TALL-104 cells.
Unfortunately, expression of tumor antigens, such as EGFRvIII, is not uniform among cells of a given tumor. Thus, adoptive immunotherapy targeting a single tumor-specific antigen can be compounded by variants in the target, allowing evasion from immune-mediated killing. This lack of efficacy has been seen with adoptive T cell therapy directed at melanoma-specific antigens, with the result of preferential survival of tumors with antigenic variants. Therefore, it is likely that the most successful adoptive cell transfer therapies will target multiple tumor antigens. Adoptive immunotherapy, like cytokine therapy, although not fully effective by itself, may become an important adjuvant to standard treatments and other immunotherapies for primary brain tumors.
Active Immunotherapy
Active immunotherapy involves priming or augmenting patients’ immunity in vivo by vaccination against tumor antigen. Tumor vaccines for malignant glioma have been the focus of great interest in recent years. Successful development of glioma vaccines, however, requires proper presentation of tumor antigens and induction of effective, durable antigen-specific T cell immune response. Early efforts in active immunotherapy used vaccines containing autologous tumor cells as a source of tumor antigens, given with various cytokines for immune stimulation. Despite evidence of safety and feasibility of such techniques, challenges remain due to innate poor antigen-presenting capacity of tumor cells, with low levels of expressed costimulatory molecules.
To augment the process of antigen presentation, professional APCs have also been used in glioma vaccines. Recent interest has turned to dendritic cells (DCs), which have an abundant expression of costimulatory molecules and have great capacity for activating T lymphocytes. DCs are exposed to tumor antigens, then used to initiate an antitumor response in the patient’s endogenous T cells. More specifically, autologous DCs are obtained from peripheral blood mononuclear cells or bone marrow, primed to maturation, exposed to whole tumor cell lysate or tumor antigen ex vivo, and reintroduced to the patient. In vitro experiments have established the ability of DCs exposed to tumor antigens in inducing T cell proliferation and generating cytotoxic responses.
In clinical trials, DCs have been exposed to tumor antigens in a variety of ways, including whole tumor cells, isolated peptides, tumor lysates, or tumor RNA. An early phase 1 trial used peptide-pulsed DCs isolated from peripheral blood and showed the generation of robust T cell infiltration into the tumor. Early efforts by Kikuchi and colleagues employed DCs fused to glioma cells and injected intradermally for patients with malignant gliomas. No adverse reactions, but a partial response in only 2 of 8 patients, was observed. In a subsequent study by these investigators, IL-12 was added to the formulation and more robust response was seen: there was a 50% radiographic reduction of the tumor in 4 of 15 patients, with similar safety profiles. A complete regression of glioma was achieved in the murine GL261 model when a regimen of intrasplenic vaccination with DC/tumor fused cells, local cranial radiotherapy, and anti-CD134 mAb 7 was given. Other strategies of DC exposure to antigens have involved using tumor lysates. Reintroduction of DCs preloaded with total tumor RNA rather than tumor antigen also produced a strong cytotoxic T cell response against autologous glioma cells. These early clinical trials have yielded encouraging results regarding generating responses and improving survival in patients. Liau and colleagues proposed that the most promising patient subgroup for DC vaccine therapy may be patients with small, quiescent tumors with low expression of tumor TGF-β. The phase 2 randomized trial using tumor lysate pulsed DC vaccine is ongoing.
The use of unselected tumor extracts to prime DCs in such nonspecific approaches risks inducing autoimmunity against antigens of normal brain. In efforts to avoid this potential hazard, focus has turned to more specific approaches using tumor-specific antigens, such as EGFRvIII as targets for glioma vaccines. Preliminary studies using EGFRvIII peptide-pulsed DCs showed generation of cytotoxic activity against the U87 human glioma cell line. When the same vaccine was given to mice that subsequently received intracerebral injections of syngeneic melanoma expressing the murine homologue of EGFRvIII, animals that were immunized survived 6 times as long as those that were not immunized. Among immunized mice, 63% survived long term and 100% survived rechallenge with melanoma, indicating evidence of immunologic memory against the tumor.
A peptide-based vaccine targeting the tumor-specific mutated portion of EGFRvIII is also under investigation. A phase 1 trial with enrollment of 19 patients has been completed. The therapy was shown to be well tolerated, and treated patients had progression-free survival of 12 months and median overall survival of 18 months. Of note, recurrent tumors after the vaccine showed no expression of EGFRvIII. The phase 2/3 randomized trial of the EGFRvIII peptide vaccine with radiation and temozolomide is currently ongoing.
Another peptide-based vaccine currently under study is based on heat-shock protein gp96 and its associated peptides isolated from the patient’s autologous tumor acquired at time of surgery. Preliminary results of the ongoing phase 1/2 trial have demonstrated the vaccine to be well tolerated, with evidence of induction of tumor-specific responses.
Alternative active immunotherapeutic approaches have aimed at initiating an immune response to tumor in vivo through lymphocytes. In one such study, peripheral lymphocytes removed from patients with high-grade gliomas were cultured with irradiated tumor cells in the presence of bacillus Calmette-Guérin and IL-2, then systemically reinfused into patients, with minimal toxicity. Two of 9 patients experienced increased survival with evidence of tumor regression, 1 had tumor regression but not increased survival, and the remaining 6 had neither.
Infectious agents have also been used to induce an antigen-specific immune response to gliomas. These vaccines contain viral or bacterial vectors carrying tumor antigen genes, and the concept involves that an immune response to the highly immunogenic infectious agent should augment the response to the tumor antigen as well. Such an approach in animal models using Listeria monocytogenes have shown efficacy against extracranial but not intracranial tumor, suggesting potential for improved efficacy in gliomas with improved delivery systems to the CNS. In a phase 1 trial, recombinantly modified vaccinia virus Ankara carrying the cDNA for a melanoma antigen has proven safe, and capable of eliciting a strong response against the virus but not against the antigen.
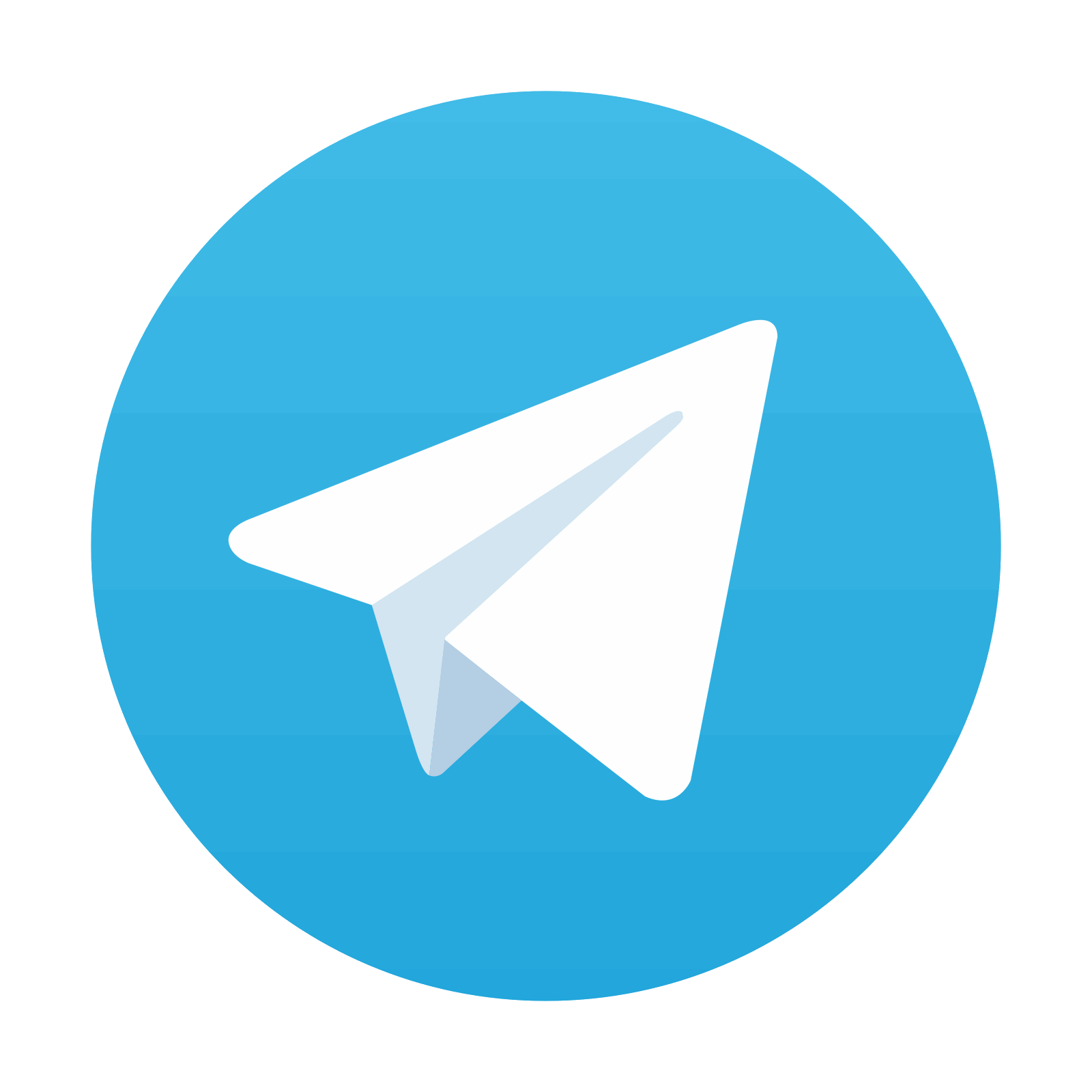
Stay updated, free articles. Join our Telegram channel

Full access? Get Clinical Tree
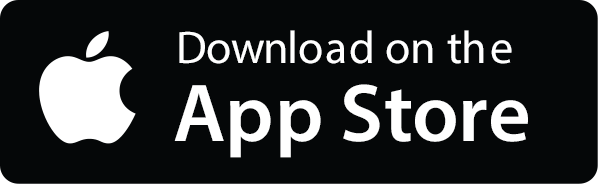
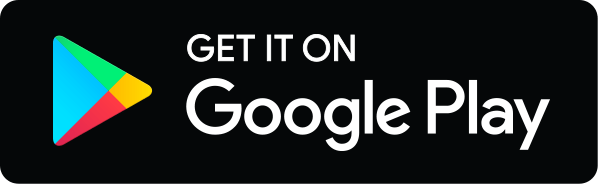