Biology of Spinal Fusion
This chapter reviews the anatomy, histology, and physiology of bone healing and the biology of spinal fusion. Bone is a dynamic biological tissue composed of metabolically active cells that are integrated into a rigid environment. The healing potential of bone is influenced by biomechanical, biochemical, cellular, hormonal, and pathological mechanisms. Normally, bone is in a constant state of flux because its deposition, resorption, and remodeling are continuous. The goals of spinal internal fixation procedures are to achieve anatomical alignment, to protect the neural elements, and to stabilize the spine mechanically while preserving the motion of normal spinal segments. These goals may be attained only by achieving arthrodesis. Ultimately, the success of a fusion depends on satisfactory bone healing. Instrumentation provides immediate but often temporary spinal fixation. Metal instrumentation is susceptible to fatigue, loosening, breakage, and failure even after a fusion has formed. Only a solid osseous union ensures long-term spinal stability. The surgical success for fusion depends on preparation of the fusion site, the ability of the graft material to induce bone healing, and the effect of systemic and local factors.
Bone Anatomy and Histology
There are three primary types of bone in humans: woven, cortical, and cancellous.1,2 Typically, woven bone has randomly arranged collagen bundles and irregularly shaped vascular spaces lined with osteoblasts. The disordered arrangement of woven bone makes it relatively weak. Woven bone is found during rapid bone formation: at birth, during fracture healing (callus formation), and in some disease states (hyperparathyroidism and Paget disease).2 Woven bone normally is remodeled and replaced with cortical or cancellous bone.
Cortical bone, also called compact or lamellar bone, forms the internal and external tables of flat bones and the external surfaces of long bones ( Fig. 34.1 ).1,2 Microscopically, cortical bone consists of densely packed regions called osteons bound on the endosteal and periosteal surface by inner and outer circumferential lamellae. Osteons, the fundamental structural units of bone, are lamellar cylinders of bone that surround longitudinally oriented vascular channels (haversian canals). Horizontally oriented canals (Volkmann′s canals) connect adjacent osteons.
Cancellous bone is interposed between cortical bone surfaces and is referred to as spongy bone, trabecular bone, or marrow. Cancellous bone has honeycombed hematopoietic interstices and bony trabeculae. The trabeculae are predominantly oriented perpendicular to external forces to provide structural support.3,4
Cellular components of bone include osteoblasts, osteoclasts, osteocytes, osteogenic precursor cells, and hematopoietic elements of the bone marrow.1,2 Mature bone cells differentiate from mesenchymal precursors.2,5,6 Osteoblasts, osteoclasts, and osteocytes are present in all types of bone.
Osteoblasts are metabolically active bone-forming cells. They are plump, cuboidal cells that secrete osteoid. As bone-forming activity nears completion, some osteoblasts are trapped within the osteon and become osteocytes incorporated into the bone structure. Other osteoblasts remain on the periosteal or endosteal surfaces as lining cells.
Osteocytes are mature osteoblasts entrapped within the bone matrix. Their cell bodies are found in lacunae. Multiple cytoplasmic processes extend to blood vessels and other osteocytes via canaliculi, which are 1 to 2 lumens in diameter ( Fig. 34.2 ).
Osteoclasts are multinucleated, bone-resorbing cells controlled by hormonal and cellular mechanisms. They resorb bone by releasing acid and proteolytic enzymes and reside in shallow pits called Howship′s lacunae. Working in groups called cutting cones, osteoclasts can dissolve the inorganic and organic matrices of bone and calcified cartilage.
Modified from Dickman CA, Maric Z: The biology of bone healing and techniques of spinal fusion. BNI Quarterly 1994; 10(1):2-12. (Modified with permission from Barrow Neurogolical Institute.)
Bone Biochemistry
Bone is composed of organic and inorganic components. Water constitutes ~20% of the weight of bone tissue.2 The weight of dry bone consists of inorganic calcium phosphate molecules (65 to 70%) and an organic matrix of fibrous protein and collagen (30 to 35%).1,2,6,7
Osteoid is the unmineralized organic matrix produced by osteoblasts and subsequently undergoes mineralization. In mature human lamellar bone, a 1-lumen layer of osteoid is produced each day. Osteoid is composed of 90% collagen and 10% ground substance, which consists primarily of noncollagenous proteins, glycosaminoglycans, and glycoproteins.2,7 The strength and rigidity of bone reflect the presence of inorganic mineral salts embedded in the organic matrix.3,4
The inorganic mineral content of bone constitutes 50% of bone volume and 70% of bone weight.2 The primary bone minerals are calcium phosphate and calcium carbonate, with small quantities of magnesium, fluoride, and sodium. The mineral crystals form hydroxyapatite, Ca10 (PO4)6(OH)2.


Hydroxyapatite precipitates in an orderly arrangement around the collagen fibers of the osteoid. These collagen fibers are type I collagen, found in skin, tendon, and fascia.1,2 Type I collagen molecules have a helical structure with extensive cross-linking and are composed of two α1 chains and one a2 chain. Osteoid initially becomes calcified within a few days; however, calcification is completed only after several months.
Bone Growth Factors
The mineral and nonmineral components in both cancellous and cortical bone undergo constant remodeling. Coupling of bone absorption and formation is mediated through bone-cell-derived growth factors, local factors, and biomechanical stresses.7–10
Several proteins cause healing bone to vascularize, solidify, incorporate, and function mechanically. Bone healing also can be influenced directly by neurotransmitters, hormones, vitamins, and minerals ( Tables 34.1 and 34.2 ).
Proteins that enhance bone healing include bone morphogenic proteins (BMPs), insulin-like growth factors, transforming growth factors (TGF-β), platelet-derived growth factor, and fibroblast growth factor, among others. A few of these proteins have been synthesized using recombinant DNA technology and have the potential to revolutionize our approach to spinal surgery.
The most well known of these proteins are BMPs, which are osteoinductive glycoproteins extracted from bone matrix. BMPs induce mesenchymal cells to differentiate into bone cells.11–13 Several BMP subtypes have been defined: osteoinductive factor, osteogenic protein, osteogenin, and osteopontin. BMP has a molecular weight of 17,500 daltons.11–13 It has a concentration of less than 1 mg/kg of bone and is most abundant in cortical bone.11–13 BMP may be extracted from gelatinized bone matrix prepared from demineralized bone, or it may be prepared from recombinant DNA techniques. Clinically, it has tremendous potential for stimulating bone healing and for promoting spinal fusion (see Bone Morphogenic Protein section).
Protein | Abbreviation |
Bone morphogenic protein | BMP |
Epidermal growth factor | EGF |
Cartilage-derived growth factor | CDGF |
Insulin-like growth factor | IGF |
Platelet-derived growth factor | PDGF |
Fibroblast growth factor | FGF |
Transforming growth factor | TGF |
Bone-derived growth factor | BDGF |
Osteoinductive factor | OIF |
Anabolic steroids |
Calcitonin |
Cancellous bone exposure of fusion bed |
Electrical stimulation |
Estrogen |
Growth hormone |
Insulin |
Large bone surface area of fusion bed |
Local growth factors |
Mechanical loading |
Mechanical stability |
Parathyroid hormone |
Somatomedins |
Testosterone |
Thyroxine |
Vitamins A and D |
Several other proteins influence bone healing in different ways. TGF-β regulates angiogenesis, bone formation, and extracellular matrix syntheses and primarily controls cell-mediated activities. Osteonectin, fibronectin, osteoponectin, and osteocalcin function as osteoconductive elements to promote cell attachment, to encourage cell migration, and to activate cells.2,7,14
Bone Growth and Development
Bones are formed by intramembranous or endochondral ossification.1,2 Intramembranous ossification occurs de novo from mesenchymal cells. Mesenchymal cells form a sheet of tissue with an abundant vascular supply and subsequently differentiate into osteoblasts. These cells then deposit collagen and osteoid, which ossifies. The collagen is arranged randomly and initially forms woven or primitive bone. The woven bone is remodeled into flat plates with an outer and inner cortical shell and intervening cancellous bone. Membranous bone forms directly without an intermediate process. Membranous bone is found in the skull, face, mandible, and clavicle.
Endochondral ossification occurs through an intermediary step in which cartilage is transformed to bone. Primitive hyaline cartilage forms from mesenchymal tissue. The cartilage is invaded by blood vessels and bone-forming cells. The cartilage becomes calcified and is reabsorbed by osteoclasts. Simultaneously, osteoblasts deposit new bone. The intermediary cartilaginous phase distinguishes endochondral from intramembranous bone formation. The skull base and the bones of the axial skeleton are formed by this process.
Throughout life there is a continuous, highly regulated process of bone reabsorption by osteoclasts and bone deposition by osteoblasts. All bone formed by remodeling is called secondary bone. In contrast, bone formed by endochondral ossification or by direct subperiosteal deposition is called primary bone.1,2
Biomechanics of Bone Grafts
Bone is a living tissue with hard and rigid characteristics that reflect the orderly deposition of minerals.2,4,14,15 Bone resists force better in compression than in tension.4 The composite arrangement of bone forms a material that is very strong for its weight and is much stronger than either the organic or inorganic components would be alone.2,3 The orientation of the collagen fibers in the matrix, which affects the material properties, is analogous to the way that the grains of lumber determine the strongest orientation of wood.2 The strength-to-weight ratio of bone is very high compared with other structural materials, such as aluminum and cast iron, and it is half as strong as steel.2,3
Cortical bone grafts are used primarily in areas that are subjected to compressive or shear stress.3 Cortical bone is compact, provides immediate structural support, and resists tensile and compressive forces.3 Cortical bone grafts have to be fixated properly to produce a satisfactory mechanical result. In comparison, cancellous bone is much weaker than cortical bone under compressive or tensile loads.3,4 Because cancellous bone grafts are relatively weak, they should be used with rigid fixation in areas subjected to large mechanical stresses. Cancellous bone has several advantages: it is more cellular and porous, becomes rapidly vascularized, and promotes fusion better than cortical bone. Therefore, the ideal reconstruction graft contains a mixture of cortical and cancellous bone (i.e., corticocancellous iliac crest struts), which combines optimum mechanical strength and bone-healing properties.
Allograft bone can be weakened during sterilization, procurement, processing, or preparation.7,15–17 Although freezing does not alter bone strength, freeze-drying significantly reduces the three-point bending, torsional stiffness, and compressive strength of allografts.17,18 Irradiation of bone grafts can reduce their bending, compression, and torsional strength and reduce their osteoinductive properties.7,17 High-dose irradiation and freeze-drying should be avoided whenever possible to preserve the biomechanical integrity of the graft. Freeze-dried allografts need to be reconstituted in saline for 30 minutes before shaping.
Mechanical and Structural Bone Adaptation
Bone remodeling and healing are dynamic phenomena that are strongly influenced by the local biomechanical environment. Functional adaptation of bone tissue is a widely recognized property. The geometry, thickness, trabecular orientation, and density of bone can change depending on the mechanical demands placed on it. Bone placed under compressive or tensile stress is remodeled. Bone is formed where stresses require its presence, and bone is absorbed where stresses do not require it. Bone can become hypertrophic or atrophic.
In 1892, J. Wolff19 developed his ideas regarding the structural adaptation of bone. It is worthwhile to understand the English translation of Wolff′s ideas: “Every change in the form and function of bones, or of their function alone, is followed by certain definite changes in their internal architecture and equally definite secondary alteration in their external conformation, in accordance with mathematical laws.”19 The modern version of Wolff′s law is the following: “Loaded areas of bone have increased deposition of bone in an effort to increase the structural strength.”2
Stress shielding is another important concept of functional adaptation that applies to spinal fixation.10,20 Rigid fixation can shield the bones from physiological stresses and can cause osteopenia or osteoporosis. However, in clinical and experimental studies, rigid instrumentation significantly improves the fusion rates in spinal surgery.20 The beneficial effects of rigid spinal instrumentation on fusion clearly outweigh the potential disadvantages of instrument-related osteoporosis. The “ideally rigid” spinal instrument system has not yet been developed. Theoretically, this device would provide temporary rigid fixation until a satisfactory fusion developed and would then eventually dissolve or loosen to allow the healed bone to be subjected to normal mechanical stresses.
Physiology of Bone Repair
A bone graft can possess several properties that can enhance healing: osteogenic, osteoconductive, and osteoinductive properties ( Table 34.3 ).6–8,15,21 Osteogenesis, the ability of the graft to make bone, requires live bone cells within the graft. Autogenous cortical bone and bone marrow are osteogenic.6 Osteoconduction is the ability of the graft to act as a scaffolding to facilitate bone healing over the entire volume of the graft. Osteoconductive materials include collagen, bone, demineralized bone matrix, hydroxyapatite, and calcium phosphate.6 Osteoconductive activity is secondary to structural properties that influence cell attachment, cell migration, cell differentiation, and vascular ingrowth.6 Osteoinduction refers to the ability to induce stem cells to differentiate into mature bone cells. BMP is the principal osteoinductive agent.6
Stages of Fracture Healing
Bone healing responses are strikingly similar in fractured bone and in bone grafts. Fracture healing restores the tissue to its original physical and mechanical properties and is influenced by local and systemic factors ( Tables 34.1, 34.2, and 34.4 ). The healing occurs in three distinct histological stages: inflammation, repair, and remodeling.1,2,6,8,10,19,21
A hematoma initially forms and an inflammatory response occurs at the fracture site during the first hours and weeks. Inflammatory cells (macrophages, monocytes, lymphocytes, and polymorphonuclear cells) and fibroblasts infiltrate the bone under the direction of chemical messengers. The initial inflammatory stage of bone healing is characterized by formation of granulation tissue, ingrowth of vascular tissue, and migration of mesenchymal cells. If anti-inflammatory drugs or cytotoxic drugs are given during the first week of healing, they may modify the inflammatory response and inhibit bone healing.
Anemia |
Antineoplastic drugs |
Bone devascularization |
Bone healing |
Bone necrosis |
Bone wax |
Castration |
Corticosteroids |
Deficiency of vitamin D, calcium, or iron |
Diabetes |
Excessive mechanical motion |
Fibrous dysplasia |
Infection |
Intoxication by vitamin A or D |
Irradiation |
Local marrow pathology (thalassemia, sickle cell disease) |
Malnutrition |
Nonsteroidal anti-inflammatory drugs |
Obesity |
Osteoporosis |
Paget disease |
Primary or metastatic tumors |
Rheumatoid arthritis |
Scar tissue |
Syndrome of inappropriate secretion of antidiuretic hormone (SIADH) |
Smoking |
A callus subsequently forms to bridge the bone defect and extends across the periosteal and endosteal surfaces, restoring the continuity of the bone. The proliferation of callus tissue is a critical step in spontaneous fracture repair. During the first 4 to 6 weeks of bone healing, callus tissue is very weak and requires adequate protection to avoid refracture and reinjury. The callus subsequently ossifies to form a bridge of woven bone between bone fragments. During this phase, adequate bone healing requires immobilization. Persistent fibrous tissue or fibrous transformation of callus tissue (fibrous union) may occur if the fixation is inadequate or the gaps between the fracture fragments are large.
Fracture healing is completed by remodeling of the bone to the original shape, structure, and mechanical strength. Remodeling occurs slowly and is facilitated by mechanical stress placed on the bone. Adequate strength of a spinal fusion is achieved only after 3 to 6 months of osseous healing. Maximum strength may develop months to years later, after the bone is remodeled and subjected to physiological stresses.
Primary (contact) fracture healing occurs by direct remodeling of areas of bone that are in apposition. Primary healing allows direct revascularization of the fracture fragments and reconstitution of the cortical bone structure; however, it also requires an exact reduction of the fractures, restoration of alignment, stable fixation, and a sufficient blood supply. Primary bone healing occurs at compressed surfaces and only with rigid internal fixation.10
Secondary (spontaneous) fracture healing occurs with formation of periosteal and endosteal callus. The callus forms an envelope that encases and bridges the fracture fragments to immobilize the injured site. The callus becomes ossified and is subsequently replaced by new bone.
The healing of a spinal fusion is more complicated than the healing of a long-bone fracture.10 The grafts are usually nonvascularized and are replaced with a continuous bone mass.10 Only superficial cells in the bone graft survive by diffusion of nutrients; the remaining graft cells die.22 Bone grafts are incorporated by an integrated process in which old necrotic bone is reabsorbed and simultaneously replaced with new viable bone. This process of incorporation is called creeping substitution.7,10
The processes of inflammation and revascularization are critical to bone graft healing.22,23 Incorporation and remodeling require that mesenchymal cells from the host bed have vascular access to the graft and differentiate into osteoclasts and osteoblasts.
Factors that can inhibit bone healing include malnutrition, obesity, cigarette smoking, diabetes, rheumatoid arthritis, medications, and variety of other factors ( Table 34.4 ). The most critical period in determining the ultimate success or failure of a fusion attempt is during the first 3 to 7 days of healing. Exposure to steroids, cytotoxic drugs (e.g., methotrexate, doxorubicin), nicotine, nonsteroidal anti-inflammatory agents, and radiation is particularly harmful during the first postoperative week.
Radiation has acute and chronic effects that inhibit bone healing. If bone is irradiated within the first 2 weeks of surgery, the effect is pronounced—radiation inhibits cell proliferation and induces an acute vasculitis.24 Delayed effects include osteonecrosis and scar tissue formation that create poor substrates for fusion.24
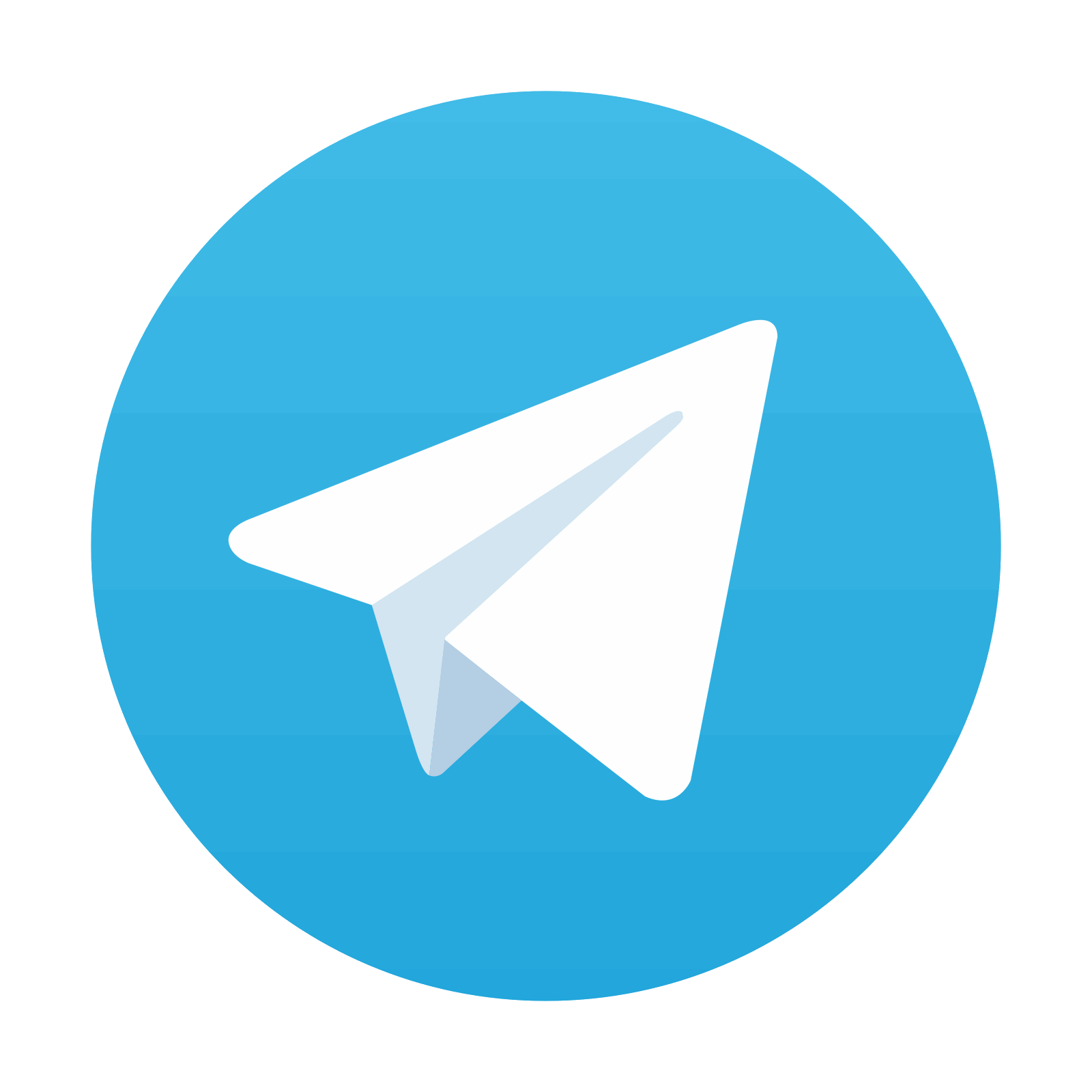
Stay updated, free articles. Join our Telegram channel

Full access? Get Clinical Tree
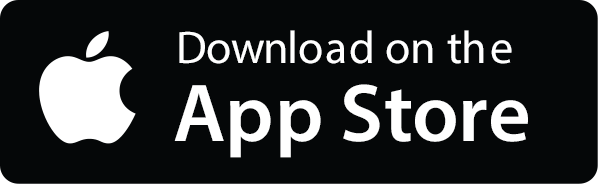
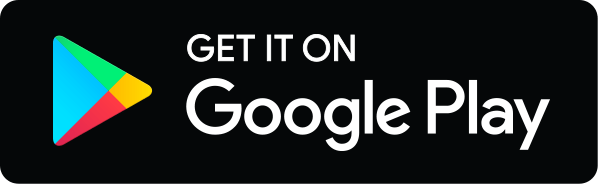
