3 Biology of the Lumbar Intervertebral Disc Ashok Biyani, Philip D. Nowicki, and Howard S. An A daily accretion in the knowledge of intervertebral disc (IVD) biology and biochemistry is advancing rapidly due, in part, to the advent of newer biochemical techniques and more precise disc models. New discoveries regarding the form and function of the IVD extracellular matrix as well as the complex interaction and balance of growth factors, proteinases, and proteinase inhibitors have opened the door for new and exciting options for clinical therapeutics of IVD degeneration. Though currently in their infancy, such therapies may one day become routine in preventing disc degradation and providing pain relief to patients with degenerative disc disease. In this chapter, we will discuss the important biological processes that characterize the intervertebral disc in both health and disease. To understand the biology of the healthy IVD, a review of the normal development and embryology of the disc is in order. The notochord is derived from embryologic mesoderm that becomes the predecessor for multiple tissues that ultimately form the spine and its various elements. The first tissue is the neural tube, which creates the formation of the floor plate.1 The second tissue is that of the ventral somites, which ultimately develop into the sclerotomes and subsequent skeletal elements of the ribs and vertebral column.1,2 Portions of this ventral somite tissue remain in an axial position and ultimately form the anulus fibrosus and the vertebral bodies through chondrification.2,3 The notochord itself degrades and disappears where the vertebral bodies condense, but is maintained in the areas between the bodies, giving rise to the nucleus pulposus (NP).1,4,5 Type II collagen has been recognized as important in this differentiation.1 A cascade of events occurs as the fetus grows, establishing the distinct spinal levels and anteroposterior polarity of the spinal column. Various transcription factors are imperative to this development and include Sox9, Pax1, Pax9, Shh, and Bapx1.2 The primitive notochord cells remain within the pulposus until approximately 12 years of age, when they develop into mature tissue.6 These cells are replaced by infiltrating chondrocyte-like cells that form a fibrocartilaginous matrix rich with chemical markers responsible for cell migration, extracellular matrix digestion, and cell proliferation.5 The gradual change of cell populations from notochordal cells to chondrocytic cells occurs at different ages in different species.7 In the human IVD, notochordal cells to predominate in the very young nucleus and are present in a limited number until the age of 12 years. Although the precise mechanism of transition from notochordal cells to chondrocytic cells in the nucleus is not known, there is evidence in rabbits and rats that chondrocytes from cartilaginous endplates migrate toward the NP region as notochordal cells undergo apoptosis.8,9 The mechanism of apoptosis of the notochordal cells is by way of either an autocrine or a paracrine Fas-mediated mitochondrial caspase-9 pathway.9 The migration of chondrocytes from cartilage end-plates into the NP is accompanied by the expression of membrane-type I matrix metalloproteinase (a marker of cell migration and extracellular matrix digestion), Ki-67 protein (a cell proliferation marker), and type II collagen (a marker for cartilaginous matrix). There are a multitude of biochemicals in the anulus fibrosus (AF) and NP. These include specific collagens, proteoglycans, growth factors, and cytokines. Each tissue type is unique in its composition, which gives each tissue distinct properties employed in their distinct structure and function. Figure 3.1 illustrates the anatomy of the anulus fibrosus and the NP in association with the spinal segment and surrounding structures. The anulus fibrosus consists primarily of highly ordered arrangements of type I collagen. This lamellar structure provides the tensile strength of the IVD.2,4–6,10–12 Approximately 70% of the dry weight of the anulus is composed of collagen molecules.4 The anulus is divided into inner and outer portions. The outer portion is composed of cells that are ellipsoidal in shape and fibroblast-like in nature. The cells are aligned in a radial fashion to the collagen fibers within individual lamellae that take and distribute loads from the NP during various types of exercise.4,5,10 The outer portion anchors the anulus and disc itself directly to the vertebral body at the endplate.4 The inner layer of the anulus is less structured and more fibrocartilaginous in nature, with an increasingly higher concentration of type II collagen and other collagen types (i.e., types V and XI). These additional collagens allow for cross-bridging of other collagen molecules and form the fibrillar collagen network to add to the strength of the anulus.4,5,10 Type VI collagen plays a unique role in the anulus, contributing along with the fibrillar collagen network to establish the major strength to tensile loads by way of fibrillar sliding.4,10,13 The inner layer of the anulus is less cellular and is composed of a pericellular matrix that extends into the NP. This inner anulus tissue helps to modify the local environment at the annular-nucleus interface.5 Included in this pericellular matrix are the proteoglycans decorin and biglycan, which are thought to help regulate fibroblast and matrix synthesis.12 Fig. 3.1 Schematic view of a spinal segment and the intervertebral disc. The figure shows the organization of the disc with the nucleus pulposus (NP) surrounded by the lamellae of the anulus fibrosus (AF) and separated from the vertebral bodies (VB) by the cartilaginous endplate (CEP). The figure also shows the relationship between the intervertebral disc and the spinal cord (SC), the nerve root (NR), and the apophyseal joints (AJ). (From Urban JP, Roberts S. Degeneration of the intervertebral disc. Arthritis Res Ther 2003;5:120–130. Reprinted with permission.) The NP is derived from primitive interconnected notochord cells that gradually disappear and leave behind a collection of sparse chondrocytes, which comprise ~1% of the total disc material.4,14 Despite this diminutive population, chondrocytes are the predominant cell type in the nucleus, decreasing in number in the central disc portions.15 Water constitutes 70 to 80% of the nucleus. Up to 50% of the dry weight of the NP is made up of various proteoglycans and collagens. Aggrecan is the predominant proteoglycan type, and type II collagen is the predominant collagen type found in the nucleus.4 The proteoglycans themselves are part of the highly organized extracellular matrix found in the nucleus. The unique and inherent chemical properties of the proteoglycans to retain water give the nucleus its main function to resist compression loads.11,12,16 This hydration ability grants the nucleus unique biologic responses to mechanical stimuli (Fig. 3.2). At low frequencies and moderate compressive forces, the nucleus is in a relative state of anabolism. With higher loading frequencies and magnitudes, higher rates of apoptosis and increased protease expression are found.17,18 This response is thought to occur from biomechanically induced secondary messengers activating intracellular calcium gradients and cytoskeletal remodeling that effects downstream gene expression and posttranslational biosynthesis.5 Fig. 3.2 The structure of human aggrecan. Aggrecan is a central core protein with keratan sulfate (KS) and chondroitin sulfate (CS) side chains. The core protein possesses three globular domains (G1, G2, and G3), with the G2 and G3 domains being separated by a KS-rich domain and two CS-rich domains (CS1 and CS2). (From Roughley PJ, Melching LI, Heathfield TF, Pearce RH, Mort JS. The structure and degradation of aggrecan in human intervertebral disc. Eur Spine J 2006;15(suppl 3): S326–332. Reprinted with permission.) The extracellular matrix of the intervertebral disc has been the focus of more recent research. Through its distinct biochemical properties, this matrix is important in the absorption of shear and rotational stresses disseminated on the annular lamellae. The presence of an extensive communication array in the anulus allows for biologic and mechanical adjustments to occur between lamellae.13 Other molecules of importance in the extracellular matrix include aggrecan, a family of proteoglycans known as leucine-rich repeat proteins, type VI collagen, and cartilage oligomeric matrix protein, among others (Fig. 3.3). Aggrecan is the most important and abundant proteoglycan found in the extracellular matrix of the nucleus. It is bound centrally to chains of chondroitin and keratin sulfate, which bond to water and maintain hydration. A collection of aggrecan molecules attach to hyaluronan at their N-terminus ends.10 At the opposite C-terminus, aggrecan can bind to other molecules of the extracellular matrix as well as to collagen and the fibrillar collagen network (Fig. 3.4).10 This creates an extensive net of crosslinked molecules that support nucleus structure and function. Trivalent pyridinoline crosslinks have been found to be important in maintaining nucleus tissue cohesiveness. With age, these crosslinks decrease and become replaced with pentosidine, a sign of advanced glycosylation.19 This substitution alters the biochemistry of the extracellular matrix and leads to degeneration. Fig. 3.3 Schematic illustration of assemblies of matrix proteins into structures in the intervertebral disc. COMP, cartilage oligomeric matrix protein; CS, chondroitin sulfate; H4, hyaluronan; HS-PG, heparin sulfate proteoglycan; KS, keratan sulfate; MAT, matrilin; PRELP, praline arginine-rich end leucine-rich repeat protein. (From Feng H, Danfelter M, Stromqvist B, Heinegard D. Extracellular matrix in disc degeneration. J Bone Joint Surg Am 2006;88A(suppl 2):25–29. Reprinted with permission.) Fig. 3.4 Structure of collagen and the proteoglycan aggrecan. Sites of attack by collagenases and other proteinases (arrowheads). CS, chondroitin sulfate; G1-G3, globular domains; KS, keratan sulfate. (From Recklies AD, Poole AR, Banerjee S, et al. Pathophysiologic aspects of inflammation in diarthrodial joints. In: Buckwalter JA, Einhorn TA, Simon SR, eds. Orthopaedic Basic Science: Biology and Biomechanics of the Musculoskeletal System. 2nd ed. Rosemont, IL: American Academy of Orthopaedic Surgeons; 2000:489–530. Reprinted with permission.) The leucine-rich repeat proteins (LRRPs) are a family of proteoglycans granting additional structural and functional properties to the extracellular matrix of the IVD. The most studied molecules in this group include fibromodulin, decorin, lumican, and versican. These proteins contain a central portion of 10 or 11 specific repeats that maintain leucine residues at conserved locations.10 This biochemical structure enables direct interaction with collagen and aids in collagen crosslinking. Decorin and lumican have been found to play an important organizational role in assembly, transport, and incorporation of collagen fibrils into the extracellular matrix.10,12 Versican is thought to attach adjacent annular lamellae to one another by way of elastic fibers, enhancing cellular adhesion as well as proliferation.12,20 These LRRPs can bind to growth factors, with a predilection for binding to transforming growth factor-β, and thus may play a role in the development of the nucleus.10 The LRRPs are expressed at various times within the life cycle of the IVD, reflecting the phenotypic differences of cell populations in different regions and at different times in the life of the disc.12 In addition to proteoglycans, the extracellular matrix is also composed of a variety of other molecules. Cartilage oligomeric matrix protein (COMP) is a pentameric extracellular matrix protein that is abundantly expressed in many tissues in the body, with the highest levels manifested in weight-bearing tissues.21 It is a molecule important for the development of cartilage and cartilage growth, as mutations in the COMP gene lead to skeletal dysplasia, pseudoachondroplasia, and multiple epiphyseal dysplasia.22,23 COMP is heavily bound to collagen and can concomitantly bind to other matrix molecules, especially in the anulus. COMP therefore may play a role in maintaining the structural stiffness of the normal IVD.21 This multimolecular binding capacity of COMP allows for the rapid and efficient regulation of collagen fibril assembly into well-defined collagen fibers and maintains the stability of the fibrillar collagen network.10 Type VI collagen is secreted into a filamentous and beaded network that binds to LRRPs, which then bind to aggrecan and other collagen types, resulting in organization of the territorial matrix.24 Type VI collagen is thought to be involved in remodeling of the pericellular microenvi-ronment.25 NG2 is a transmembrane proteoglycan that has been found to be closely associated with type VI collagen and the pericellular matrix.26–28 Because of this association, NG2 is thought to be involved in cell-matrix interactions and transmembrane signaling pathways.27 Specifically, NG2 binds to growth factors such as platelet-derived growth factor and basic fibroblast growth factor.29,30 This binding potential likely makes NG2 important in cellular proliferation and migration.27 The function of cartilage intermediate layer protein in the nucleus has yet to be elucidated, but it has been shown to be involved with degeneration of lumbar discs.31 The health of the IVD is dependent upon a balance between anabolic and catabolic factors as well as cellular demand and transportation limits.6,11,32,33 There are numerous factors important in this balance, including blood supply, cellular metabolism, cytokines, growth factors, proteinases, proteinase inhibitors, and mechanical stimuli. The IVD is almost completely avascular. Blood vessels are limited to the periphery of the AF. There is minimal penetration into the inner disc layers, leaving the central portion of the NP without direct nutritional supply (Fig. 3.5).4,15,33 The metabolism of the disc is therefore highly dependent upon diffusion and glycolysis.33,34 The vertebral endplates, which are composed of hyaline cartilage, are porous in structure and directly abut the blood vessels of adjacent vertebral bodies to allow for nutrient diffusion to occur.15 Oxygen tension, pH, and glucose concentrations are the main determinants of cellular biology in the IVD. Their levels are closely maintained within specific narrow limits to allow optimal metabolism and matrix synthesis.33 The osmotic pressure gradient is also important for disc metabolism. This gradient is affected by mechanical forces within the disc that help to regulate disc height and hydration, as well as intracellular signaling responsible for cell death or survival.5 If the cellular environment of the disc strays too far from optimal concentration gradients, apoptosis and degradation ensue. Fig. 3.5 Schematic showing the concentrations of oxygen, glucose, and pH levels across the disc from endplate to endplate. (From Grunhagen T, Wilde G, Mokhbi Soukane D, Shirazi-adl SA, Urban JP. Nutrient supply and intervertebral disc metabolism. J Bone Joint Surg Am 2006;88A(suppl 2):30–35. Reprinted with permission.) IVD anabolism is regulated by insulin growth factor (IGF), transforming growth factor-β (TGF-β), and the bone morphogenetic protein (BMP) family. These molecules are involved in extracellular matrix production and regulation.11,35 IGF has been shown to stimulate prostaglandin synthesis in the NP of bovine cells,36 but gradually with time this response by IVD cells diminishes.37 TGF-β has demonstrated similar effects on prostaglandin synthesis but also has shown the ability to stimulate disc regeneration.38 Contrary to these findings, some authors have found that high TGF-β concentrations may actually be toxic to disc cells and in fact inhibit prostaglandin synthesis.39 BMP-2 appears to increase cell proliferation, prostaglandin synthesis, expression of type II collagen messenger ribonucleic acid (mRNA), aggrecan, and osteocalcin production in the AF.40,41 BMP-2 is inhibited in the NP by nicotine.41 BMP-2 also seems to be important in maintaining disc height, as direct injection into discs has been shown to reverse early height loss.42 When utilized in conjunction with TGF-β and IGF-1, BMP-2 is especially beneficial in stimulating prostaglandin synthesis.43 Osteogenic protein 1 (OP-1), also known as BMP-7, stimulates and retains proteoglycan and collagen metabolism, doing so in fetal, adult, and old bovine annular and nuclear cells.44–46 OP-1 has a role in repair of the degenerated nuclear extracellular matrix and the anulus.47,48 OP-1 may play a role in maintaining, restoring, and regenerating intervertebral disc height as evidenced in multiple disc injection studies.49–51 Annular and nuclear cells respond to OP-1 by dividing as well as enhancing their synthesis of aggrecan and collagen.35 Growth and differentiation factor-5 (GDF-5) is a member of the BMP family. Its absence has been implicated as a causative factor in multiple skeletal dysplasias.52,53 It is also necessary for normal joint development as it is thought to play a crucial role in stimulating the formation and proliferation of chondrogenic cells.52,53 In IVDs void of GDF-5, total glycosaminoglycan concentration is decreased, but total collagen is not altered; there is also a downregulation of type II collagen and aggrecan genes.53 When rhGDF-5 was injected into degenerated IVDs, a dramatic restoration in disc height was observed.52 The NP maintained its hydrophilic properties on magnetic resonance imaging (MRI) and chondrocytic cell concentration increased in the nucleus and anulus.52 Injection of OP-1 (BMP-7) and GDF-5 into degenerated discs was found to have similar results, but the responses by the disc cells differed. OP-1 was found to have more of an effect on proteoglycan synthesis,54 whereas GDF-5 was found to stimulate proteoglycan and collagen synthesis in a similar manner.52 The biology of the healthy IVD is invariably dependent upon the balance between anabolic and catabolic factors (Fig. 3.6). Disc degeneration is complex and involves alterations in local biomechanical forces and loads, cell loss, disc nutrition and metabolism, and quantitative and qualitative changes in matrix production. Figure 3.7 illustrates the gross appearances and differences between healthy and degenerated discs. The inciting factor for the beginning of the disc degeneration cascade continues to elude researchers. Any one of these factors or multiple simultaneous factors may be responsible for initiating disc degeneration, and not necessarily one over another in sequence (Fig. 3.8). The health of the IVD is dependent on its ability to retain water. As water content decreases, not only does the local biologic environment change but the biomechanical environment changes as well. With diminished water content, the disc becomes more susceptible to damage produced by abnormal forces. Bending and compressive loads can lead to disc bulging, endplate deformation, and volumetric changes within the nucleus, whereas torque loads deform the anulus. These changes in biomechanical loading can lead to accelerated deformation and degeneration and will be discussed in more detail in a later chapter. Cell numbers within the IVD diminish with age and result in higher levels of apoptosis, especially in the anulus.55,56 These cells are not necessarily biologically inactive, but instead produce inappropriate matrix molecules such as matrix metalloproteinases (MMPs).56 Altered gene expression, altered posttranslational modifications, and altered transcription factors all contribute to this cellular senescence. These cell changes ultimately lead to a decreased ability for intact cells within the anulus and nucleus to recover from deforming forces.15 Intrinsic and extrinsic factors that decrease the vascular supply to the disc increase the risk for degeneration. Examples of such factors include sickle cell anemia, atherosclerosis, vibrational forces, trauma, cigarette smoking, scoliosis, and genetic predisposition.11,33,57 The presence of any one or more of these factors may lead to endplate calcification between the vertebral bodies and the IVDs. Endplate calcification affects cellular metabolism by diminishing the diffusion capacity of the disc and leads to inappropriate changes in vital metabolic gradients. If the microenvironment pH rises due to lactate formation from decreased oxygen tension, glycolysis diminishes and leads to the inhibition of cellular metabolism and the activation of apoptosis.33 Extracellular matrix synthesis and degradation are directly dependent upon disc metabolism. If the diffusion concentration of glucose falls below critical levels, apoptosis rapidly accelerates.33 If cell metabolism decreases, the cell phenotype changes and leads to decreased matrix synthesis and degeneration of the remaining matrix.33 Disc degeneration occurs from inside of the nucleus toward the outer nucleus as cells in the internal portion have the smallest concentrations of vital metabolic gradients (Fig. 3.5).58 Changes in cell phenotype activate new genes that activate the apoptotic cascade, the inflammatory response, and production of proteases. Specific enzymes and molecules involved in these degrading processes include the family of MMPs, aggrecanases, and proinflammatory cytokines.
Biology of the Normal Lumbar Intervertebral Disc
Development
Structure and Function
The Extracellular Matrix
Cellular Biology
Growth Factors
Biology of the Diseased Lumbar Intervertebral Disc
Cellular Changes
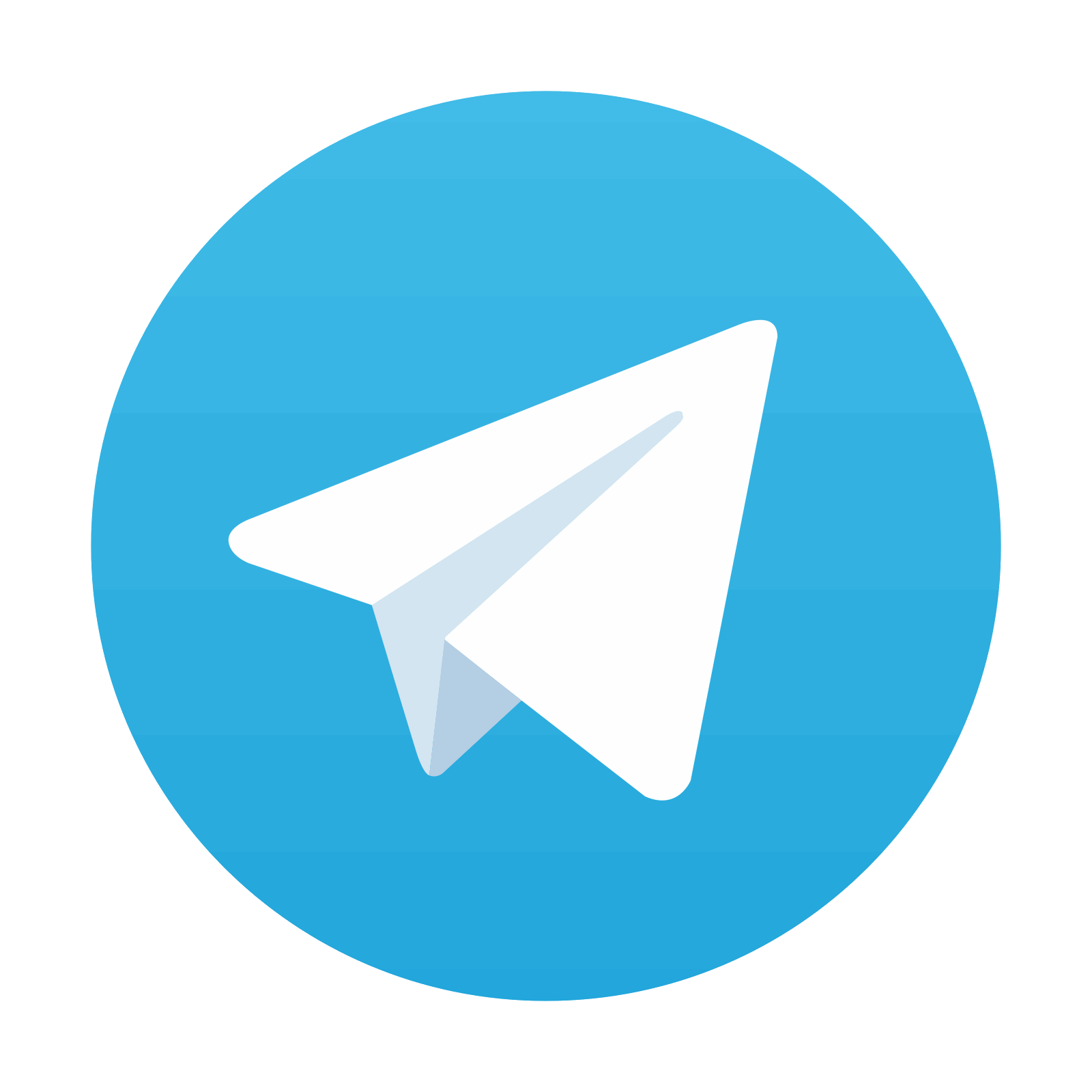
Stay updated, free articles. Join our Telegram channel

Full access? Get Clinical Tree
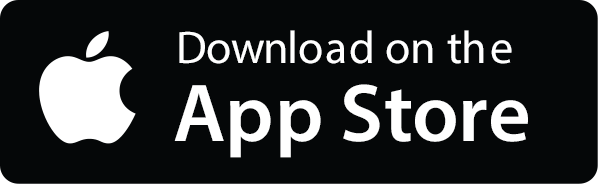
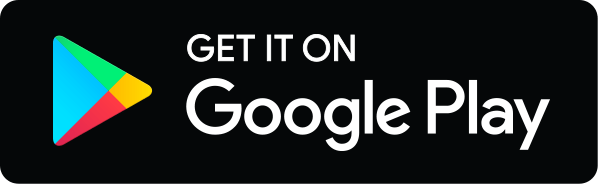