9 The transfusion of blood products in the perioperative setting is often necessary to provide adequate tissue oxygenation and to maintain hemostasis.1–3 The benefits of these transfusions, however, need to be balanced against the risks of hemolytic and febrile transfusion reactions, volume overload, transfusion-related acute lung injury (TRALI), immunosuppression, intravascular thrombosis, and transmission of infectious agents. The appropriate use of transfused blood products relies upon recognition of these risks and benefits and an understanding of the collection, preparation, and storage of blood components, and their biochemistry. Units of blood collected for use in the United States are exclusively obtained from volunteer donors.4 The donated units are ABO and Rh typed and screened for infectious agents including bacteria, syphilis, hepatitis B and C, human immunodeficiency virus (HIV) 1 and 2, human lymphocytotropic virus (HTLV) 1 and 2, West Nile virus, and Trypanosoma cruzi. In addition, the level of alanine aminotransferase is measured as a surrogate marker for hepatitis. In the future, screening for other blood-borne pathogens or exclusion of donors may be required, because of immigration from areas where malaria and other blood-borne infections are prevalent. For example, donors who have lived in the United Kingdom for more than 3 months between 1980 and 1996 are excluded from blood donation due to the theoretical risk of transmission of bovine spongiform encephalopathy. Red blood cells are stored at 1° to 6°C in the acid-citrate-dextrose (ACD) or citrate-phosphate-dextrose (CPD) solution to which adenine, glucose, and mannitol have been added. Under these conditions, the red blood cells (RBCs) are suitable for transfusion for up to 6 weeks.5 Older units of RBCs may have a shorter survival in recipients, and may be less efficient in oxygen exchange; however, there are no clear-cut recommendations regarding the use of older versus newer RBC units.6 In some instances, units of RBCs can be frozen in a glycerol solution and stored for longer periods of time. Since 1996, filtration of blood at the site of collection has been mandated as a means of reducing white blood cell contamination of blood products. The contamination of blood products by white blood cells (principally lymphocytes) and their products increases the risk of febrile nonhemolytic transfusion reactions by the passive transfer of cytokines released during the processing and storage of blood. Contaminating leukocytes can sensitize the recipient to human leukocyte antigens (HLAs) and generate antibodies that will shorten the survival of transfused platelets, leading to shortened survival of the transfused cells. In addition, lymphocytes containing cytomegalovirus (CMV) can infect a seronegative recipient and produce clinically significant pneumonia, colitis, hepatitis, or retinitis, particularly if the recipient is immunosuppressed.7,8 In severely immunocompromised patients, such as those receiving chemotherapy, radiation therapy, bone marrow and solid organ transplant, or potent immunosuppressive agents, viable lymphocytes from the donated blood product (usually RBCs or platelets) may engraft and cause fatal graft-versus-host disease (GVHD). The symptoms of GVHD include skin eruptions, diarrhea, fever, thrombocytopenia, and hepatic dysfunction. This complication can be prevented by irradiation of the blood products prior to transfusion to render any contaminating lymphocytes incapable of proliferation.9 As noted above, the need for filtration leukoreduction for all units of donated blood has made the collection of platelet concentrate from individual blood units difficult. Many transfusion departments exclusively collect platelets by apheresis from volunteer donors. A donor can provide about 3 × 1011 platelets per treatment, which is the equivalent of four to six units of platelets prepared from single units of blood. The higher costs of this method of collection is offset by the potential benefits of reduced exposure of the recipient to multiple donors, reduced risk of HLA sensitization, improved platelet survival, and the potential for HLA matching of donors with patients who are refractory to transfusion.10 If the recipient has not received a blood transfusion or has not been pregnant within the past 3 months, often electronic crossmatch can be performed because the risk of clinically significant antibodies would be low. In other instances, a screen for alloantibodies may be necessary to prevent an immediate or delayed hemolytic transfusion reaction. It is important to note, however, that most instances of clinically significant transfusion-associated hemolytic episodes are not due to minor blood group antigen incompatibility. Instead, errors in patient identification and specimen labeling of the type and screen at the bedside are responsible for these potentially lethal events.11 The transfusion of one unit of packed RBCs, which contains a hematocrit of ~ 0.70 and a volume of ~ 225 mL, should increase the hemoglobin by 1 g/dL and hematocrit by 0.03. Although the increment of hemoglobin following the transfusion of units of RBCs is well known, the criteria for their use in the perioperative setting have changed in the past decade as the indications for transfusions have become more rigorous. The traditional recommendation of maintaining a hemoglobin concentration of 10 g/dL and hematocrit of 0.30 for patients undergoing surgery has been critically challenged.12–16 A series of studies evaluating the risks and benefits of restriction of RBC transfusions in cardiac and orthopedic surgery have demonstrated that patients undergoing surgery with hemoglobin concentrations of greater than 10 g/dL do not require transfusions, whereas those with hemoglobin concentrations of less than 7 g/dL will benefit from this intervention. Bracey et al17 performed a randomized controlled trial of 428 patients undergoing coronary artery bypass surgery in which patients were transfused at hemoglobin concentrations of either less than 9 g/dL or 7 g/dL. Although the rate of transfusion was higher in those patients allocated to the higher hemoglobin goal, outcomes including fatigue scores, morbidity, and mortality, were similar in both groups. This study also indicated that the use of blood transfusions for patients with hemoglobin concentrations that were greater than 8 g/dL did not reduce perioperative mortality. These studies have also suggested that the risk of infection and prolonged hospitalization was associated with more liberal use of RBC transfusion Hébert et al13 studied 838 critically ill euvolemic, nonsurgical patients, and randomly assigned 418 to a restrictive transfusion strategy that maintained the hemoglobin between 7 and 9 g/dL compared with patients maintained at hemoglobin concentrations at 10 to 12 g/dL. In the subgroup analysis of the study, patients who were less ill (Acute Physiology and Chronic Health Education [APACHE] II score ≤ 20) and younger had better outcomes with a more restrictive transfusion protocol.18 Conversely, patients with a history of angina or congestive heart failure did worse with the more restrictive criteria for transfusion. The conclusions that were derived from this study were that rather than relying upon a fixed target hemoglobin, the patient’s overall cardiovascular function needs to be assessed, and RBC replacement adjusted accordingly. Similar conclusions have been reached by meta-analyses of clinical trials of patients undergoing elective surgical procedures. The advantage of more liberal or restrictive transfusion policies in neurosurgical patients is less clear. Flückiger et al19 observed that acute resuscitation of patients with traumatic brain injury to a hematocrit of 0.28 was associated with improved outcomes. There are contrasting results regarding the optimum transfusion goal in patients with subarachnoid hemorrhage (SAH) and patients with intracerebral hemorrhage (ICH). Although arterial blood flow may be favored by a lower hemoglobin concentration and blood viscosity, this might be at the expense of tissue oxygen delivery. Naidech et al20 studied the effects of hemoglobin concentration in a retrospective study of 103 patients with SAH. Univariate analysis showed that a high hemoglobin concentration on day 0 and day 1 following the SAH predicted a better clinical outcome. Serial determinations of hemoglobin concentration also showed that SAH survivors had consistently higher values during their hospital course. A similar conclusion was reached by Sheth et al,21 who retrospectively reviewed 546 patients with nontraumatic ICH. These investigators noted an improved 30-day survival in patients receiving RBC transfusions compared with nonanemic, nontransfused patients.21 Unfortunately, in neither of these studies was there a consistent hemoglobin concentration that triggered the decision for transfusion. Thus, precise recommendations of optimum hemoglobin concentration in ICH and SAH remain elusive. Although most reviews of blood use in neurosurgery indicate that many more units are screened and prepared than are used, preoperative and intraoperative measures are available to further reduce the need for allogeneic blood transfusion.22–26 Preoperative treatment of the elective patient with erythropoiesis-stimulating agents (ESAs, darbepoetin and erythropoietin) have been proposed as a means of raising the hemoglobin concentration and enabling the patient to provide autologous blood for use during surgery.27,28 Although this approach has been widely used in orthopedic surgery (including spine surgery) and had been shown to reduce use of allogeneic transfusions, its application to neurosurgery is less clear. In addition, the use of ESAs has been associated with an increased risk of thrombosis; thus, this approach may be undesirable in neurosurgical patients who are already at increased risk of thrombotic events. Thrombocytopenia in the neurosurgical patient poses a unique problem because hemorrhage, which would be considered trivial in abdominal, cardiovascular, or gynecologic surgery, may be catastrophic following procedures in the axial or peripheral nervous system. Unfortunately, there is no evidence-based information to determine a “safe” level of platelet count prior to neurosurgery.28,29 Several consensus statements have chosen a value of 100,000/μL as a minimum platelet count; however, the patient’s risk of hemorrhage is affected not only by the number of platelets but also by their function.30 A patient with a platelet count of 200,000/µL who has been treated with aspirin or clopidogrel is at a much greater risk of bleeding than a patient with immune thrombocytopenia and a platelet count of 80,000/µL.31,32 Consequently, decisions regarding platelet transfusion in the neurosurgical patient need to be based on the complete medical history. Patients with a platelet count less than 100,000/μL who require neurosurgical intervention will usually receive platelet transfusions. One unit of apheresis platelets will increase the platelet count in a patient with normal clearance of platelets by 40,000 to 60,000/μL. The efficacy of this replacement can be reduced by the presence of platelet alloantibodies (which might develop in multiply transfused patients such as those requiring transfusions during the course of chemotherapy or bone marrow failure) or by intravascular consumption due to disseminated intravascular coagulation or thromboses. Drug-induced thrombocytopenia might also lead to reduced survival of transfused platelets. Consequently, the adequacy of the response to platelet administration should be documented within 1 to 2 hours following transfusion to ensure adequate hemostasis during and following a surgical procedure. Even with normal platelet survival, the need for additional platelet transfusions should be anticipated. The platelet count should be maintained at greater than 100,000/µL for at least 48 to 72 hours following a neurosurgical procedure, and greater than 50,000/µL for the next 7 days. Radiographic follow-up for evidence of hemorrhage into the operative site is prudent even with maintenance of an adequate platelet count. Since the advent of the HIV epidemic, patients and physicians have sought to limit exposure to allogeneic units of blood. Two commonly employed approaches are directed donations and autologous donations. With autologous blood donation, the patient donates one to two units of whole blood prior to elective surgical procedure. As noted above, ESAs may be employed; however, the safety of their use has been questioned.27,33 The advantage of this approach is the ability to replace RBCs and plasma volume with a product that does not entail the risks of transmission of disease, transfusion reaction, or alloimmunization.34 The autologous donation programs place a burden on the transfusion service, as they require separate record keeping, a guarantee that the autologous units are administered to the appropriate patient, and the development of a policy for disposition of units not used during surgery. This last point is surprisingly complex; often patients are discharged from the hospital following surgery without using the autologous units, only to wind up needing them several days later. The blood bank needs to be certain that the units are not disposed of (either by wasting them or administering them to another patient) before determining that the donor is no longer in need of them. An additional complication ensues when the patient might be otherwise ineligible to donate blood due to HIV or hepatitis B or C infection. These units need to be strictly quarantined from the remainder of the blood supply, and management of these blood products places an additional administrative and medicolegal burden on the transfusion service. Directed donation of blood products are often requested by patients with the belief that products from friends or family members are inherently safer than those obtained from volunteer donors. Epidemiological and serological studies of the risks of transfusion-associated disease have shown no difference in the rates of HIV infection and hepatitis B and C infection among directed donors compared with the general donor population.35 In addition, ABO or Rh incompatibility may make the directed donations of RBCs useless for the intended patient. Direct donations of single-donor apheresis platelets can be useful in supplementing the supply of blood products, particularly in patients who, due to alloimmunization and prolonged myelosuppression, may have a prolonged need for platelet transfusions. In general, however, directed donations should be discouraged if their use is based on the perception of greater safety of the blood products from the recipient’s friends and family members. Jehovah’s Witnesses refuse the administration of blood products on religious grounds. Generally, patients whose religious principles lead them to avoid blood products are sophisticated regarding the consequences of this refusal; release forms describing the risks of not accepting blood products are usually available in most health care facilities and may help to absolve the physician of a poor outcome due to severe anemia or bleeding.36 The magnitude of the risk of refusal of blood has been reported by Carson et al37 in patients undergoing abdominal, orthopedic, or neurosurgical procedures. The 30-day mortality following a variety of surgical procedures was 0% when patient’s postoperative hemoglobin was 7.1 to 8.0 g/dL. This mortality rate rose to 30% with the postoperative hemoglobin concentrations of 3.1 to 5 g/dL and to 64% with hemoglobin concentrations of 3 g/dL or less.37 The experience of neurosurgery without the use of blood products was reported by Suess et al,38 who described cranial and spinal neurosurgical procedures in 103 Jehovah’s Witness patients compared with 515 control patients. Surgical hemostasis was maintained with electrocautery and topical hemostatic agents, and volume expansion was maintained only with crystalloids and colloids. A cell saver was used in 34% of the patients undergoing spine surgery. Interestingly, the blood loss from the 103 Jehovah’s Witness patients was 34.5% less than from the control group, although their length of hospitalization was 15% longer than that of the controls.38 The management of patients who refused to accept blood transfusions includes the use of ESAs to raise the hemoglobin prior to surgery, the use of RBC sparing, the use of procedures such as cell savers during the course of the surgery, and the potential use of blood substitutes. The use of erythropoietin prior to surgery may require several weeks to obtain an optimal response. Consequently, elective procedures should be planned well in advance. The postoperative administration of erythropoietin will also have a delayed onset of effect. As noted earlier, the use of these medications may be associated with the risk of thrombosis, and consequently these risks should be considered in formulating a treatment plan. The use of cell savers to scavenge blood from the operative field has been widely used in abdominal, thoracic, and orthopedic surgery.24,39 It should be noted, however, that Jehovah’s Witness patients usually accept the use of this device only if the blood circuit is completely closed, and there is no offline processing of the blood products. Blood substitutes have been in development for over 80 years, but no truly satisfactory product has become commercially available. Initial attempts with the use of fluorinated hydrocarbons were unsuccessful due to the low oxygen-carrying capacity of these molecules and the risk of thrombosis, including stroke and myocardial infarction.40 The use of stroma free hemoglobin has been investigated in patients requiring blood resuscitation following trauma.41,42 The use of these products has also been complicated by the risk of thrombosis, vasoconstriction, and hypertension, probably mediated by the ability of the hemoglobin to antagonize the vasodilatory effect of nitric oxide.43 In addition, unmodified stroma free hemoglobin is rapidly cleaved into dimers and cleared by glomerular infiltration; this property may contribute to the nephrotoxicity of this form of hemoglobin. Because the free hemoglobin is not bound to 2,3-diphosphoglycerate as in an intact RBC, it has a higher affinity for oxygen and is less efficient in its release of oxygen to the tissues. Modifications of the hemoglobin molecule, including polymerization and internal cross-linking, may reduce the nephrotoxicity and improve the tissue oxygen delivery.43 Case reports of the compassionate use of a polymerized bovine hemoglobin oxygen carrier (HbOC-1, Hemopure, Biopure Corporation, Cambridge MA) in patients with sickle cell anemia, hemorrhage, acute leukemia, and hemolytic anemia have been reported, with six of eight patients surviving.44 Complications included hypertension, thrombosis, and methemoglobinemia. Thus, although these products may have some benefit in an emergency, they remain investigational. Fresh frozen plasma is isolated from donated blood and stored at −18° to −30°C; it has a shelf life of at least 1 year. The principal use of this product is to replace coagulation factors in situations where coagulation factor concentrates are not available, to treat patients with multiple coagulation factor deficiencies (i.e., liver disease), to acutely reverse warfarin anticoagulation, to treat thrombotic thrombocytopenic purpura, to replace rare plasma protein deficiencies (C-1 esterase inhibitor, congenital thrombotic thrombocytopenic purpura [TTP]), and to treat patients requiring massive transfusion with RBCs and crystalloid.45 The usefulness of FFP in replacing coagulation factors is limited by the low concentration of the coagulation factors relative to their need for hemostasis.46 For example, 1 mL of FFP contains one unit of factor VIII (FVIII) activity. A patient with severe hemophilia A (FVIII level of < 1%) requires a level of about 78 to 80% to safely undergo a surgical procedure. The patient’s plasma volume can be calculated as (Weight [kg] × 70 mL/kg) × (1-Hematocrit). Thus, for a 70-kg patient with a hematocrit of 45, the plasma volume will be 2695 mL, and 2156 mL of plasma (or ~ 9 units) will be need to supply the requisite coagulation factor activity. In so doing, however, the plasma volume will be almost doubled, leading to dilution of the net coagulation factor activity, not to mention the risk of circulatory overload from the volume of the plasma. As a practical matter, the optimum increment of coagulation factor levels that can be achieved by the use of FFP is reached at a dose of ~ 15 mL/kg and results in an increase in coagulation factor levels of 10 to 12%.45 The usefulness of FFP as a source of coagulation factors is further limited by the short half-life of clinically important coagulation factors.45 A common use of FFP is correction of a prolonged prothrombin time due to treatment with warfarin. Warfarin depletes the activity of the vitamin K–dependent coagulation factors II, VII, IX, and X; however, the depletion of factor VII is its most hemostatically relevant effect. The half-life of factor VII is only 5 hours; thus, FFP replacement in this situation is only a temporizing measure until replacement of vitamin K more durably reverses warfarin anticoagulation. These inadequacies of FFP treatment of coagulopathy were emphasized by the study Abdel-Wahab et al,47 who analyzed data from 121 patients treated with FFP to correct to an international normalized ratio (INR) of 1.1 to 1.85. Adequate correction of the INR 8 hours after transfusion was achieved in 0.8% of patients, and there was no benefit in reduction in blood loss. These data have particular significance in the preoperative assessment of neurosurgical patients, as the importance of mild prolongation of the INR may not portend an increased risk of hemorrhage. Matevosyan et al46 assayed coagulation factor levels in 25 neurosurgical patients with INRs of 1.3 to 1.7. They identified hemostatically adequate levels of coagulation factors II, VII, and VIII. The discordance between the INR and the coagulation factor levels may be due to the use of sensitive reagents (principally recombinant tissue factor) in the prothrombin time assay. Based on these finding, the authors recommended against the preoperative use of FFP in patients with slight prolongation of the INR.46,47 Concentrates of factors II, VII, IX, and X are prepared by precipitation anion exchange chromatography, and rendered free of infectious agents by solvent detergent treatment or by heating. These complexes may contain variable amounts of the coagulation factor activities and are usually normalized for the activity of factor IX, because their principal use has been for the treatment of hemophilia B. These concentrates have been proposed as treatment for intracerebral hemorrhage secondary to over-anticoagulation with warfarin along with the use of vitamin K.48 Although several studies indicate that there may be a beneficial effect as measured by shortening of the prothrombin time and normalization of the INR, it should be noted that not all preparations are identical.48 Specifically, the majority of the anticoagulant effect of warfarin occurs by its ability to decrease the activity of factor VII. Preparations such as Bebulin (Baxter, Deerfield, IL) have a very low level of factor VII, whereas Octaplex (Octapharma, Hoboken NJ) contains almost as much factor VII as factor IX activity. Consequently, it is important to be aware of the composition of the prothrombin complex concentrates that are available if they are to be used for this off-label indication. As mentioned previously, the half-life of factor VII is very short; thus, repeated doses of prothrombin complex concentrates (PCCs) may be necessary until the effect of vitamin K becomes evident. Additional concerns regarding the use of PCCs are their potential thrombogenicity.48 Following their introduction over 40 years ago, multiple reports of local or disseminated thrombosis were associated with these products. It has been appreciated that older manufacturing methods generated activated coagulation factors that were responsible for these occurrences. Contemporary manufacturing techniques, including the use of low levels of heparin, antithrombin, protein C, and protein S in the final product, have reduced this complication of therapy.48 The thrombogenicity of PCCs, which is ascribed to the presence of activated factor VIIa, has led to their use in the treatment of patients with factor VIII inhibitors. This condition, in which a patient receiving factor VIII replacement for the treatment of hemophilia A develops an antibody that inhibits the activity of factor VIII, makes treatment of bleeding episodes with additional factor VIII impossible.49 The activated coagulation factors in PCCs, particularly factor VII, are able to bypass the inhibitor and restore hemostasis. Recombinant activated factor VII (rFVIIa) was developed to address the problem of the factor VIII inhibitors and to avoid the thrombogenicity of PCCs and the potential viral contamination of these plasma-derived products.49 Doses of rFVIIa of 90 µg/kg have been used in patients with these inhibitors with good control of hemorrhagic events. Because this molecule leads to intense activation of the coagulation cascade, it has been proposed as a “broad-spectrum” hemostatic agent that might be used to treat intracerebral hemorrhage, hemorrhage due to warfarin treatment, or postoperative hemorrhage. Patients with either traumatic or spontaneous intracerebral bleeding have been treated with rFVIIa at doses between 40 and 160 µg/kg. At these doses a reduction in the volume of the intracerebral hematoma was observed, but in most studies there was no impact upon survival or neurologic function at 90 days.50,51 Patients receiving this product also had an increased incidence of arterial and venous thromboses.50 Recombinant factor VIIa has also been evaluated as a treatment of ICH in patients with supratherapeutic anticoagulation with warfarin. Although the INR values were rapidly corrected following treatment, there was no benefit with respect to survival in the patients receiving rFVIIa as opposed to FFP and vitamin K. Consequently, the use of rFVIIa in spontaneous or traumatic ICH is not recommended.50 The transfusion of blood products can be associated with adverse events including acute hemolytic transfusion reactions, febrile nonhemolytic transfusion reactions, TRALI, and delayed hemolytic transfusion reactions. Although the understanding of the pathophysiology of these events has reduced their incidence and provided guidance for further management, these potential complications remind physicians that blood transfusions are not a trivial component of medical and surgical care. Acute hemolytic transfusion reactions occur when RBCs are transfused into ABO-incompatible recipients. The immunoglobulin M (IgM) isohemagglutinins, which are present in persons with blood groups A, B, or O, will bind to mismatched RBCs, fix complement, and cause intravascular hemolysis.52 The hemoglobin liberated by this process will precipitate in the renal tubules and lead to acute tubular necrosis and renal failure. The intravascular release of RBC membrane stroma initiates disseminated intravascular coagulation. The patient usually presents with back pain, hemoglobinuria, fever, and hypotension. Although the severity of these reactions may be mitigated by treatment with intravenous fluids, corticosteroids, and alkaline diuresis, the outcome is often fatal. A catastrophic acute hemolytic transfusion reaction is almost always due to preventable errors at the bedside, leading to patient misidentification or sample mislabeling. Although clerical or technical errors in the blood bank laboratory can occur, they are seldom the cause.11 Consequently, institutions need to have processes in place to ensure proper identification of the type and screen specimens entering the blood bank laboratory and the units of blood product leaving the laboratory. These processes include obtaining duplicate type and screen specimens from patients who have not previously been seen at the institution, labeling and signing each type and screen specimen, reviewing the previous transfusion history to identify inconsistencies of ABO and Rh information, and conforming demographic data by two identifiers. Patients who present to the emergency department without identification pose a unique problem in this regard, and institutions should establish a formal plan for this situation to reduce the risk of patient, blood sample, and blood product misidentification.11 Febrile nonhemolytic transfusion reactions (FNHTRs) are the most commonly encountered adverse transfusion event, and they may occur following the administration of any blood product.52 These reactions are caused by the release of cytokines from lymphocytes in the donated blood product. Common symptoms include fever, tachycardia, bronchospasm, hypoxemia, and hypotension; rarely they may be fatal. The differential diagnosis of this presentation includes sepsis, pulmonary emboli, myocardial infarction, drug allergy, and TRALI, and these entities should be considered and investigated. These reactions, however, are not associated with hemolysis or liberation of free hemoglobin. The incidence of FNHTR has been reduced by universal leukodepletion by filtration of blood at the time of collection. Nonhemolytic febrile transfusion reactions can be treated with acetaminophen and antihistamines, or, in more severe situations, with corticosteroids. In addition, premedication with acetaminophen antihistamines may be useful in preventing these events. Transfusion-associated lung injury occurs 6 to 8 hours following the administration of blood products (usually FFP or platelets). The patient develops dyspnea and noncardiogenic pulmonary edema, which may be confused with transfusion-associated cardiac overload (TACO), infection, pulmonary embolization, or drug allergy.53 Histopathological examination of the lungs of patients with TRALI shows aggregates of neutrophils as well as proteinaceous fluid in the alveolar spaces. The neutrophils may be activated by passive transfer of anti–HLA I and II antibodies or antineutrophil antibodies from FFP or from the plasma contained in platelet or RBC transfusions.54 These antibodies bind to the normal marginated pool of neutrophils that resides in the pulmonary circulation and causes release of the cytokines and lysosomal enzymes.55 An alternative explanation of this event is that preexisting activation of the neutrophils contained in the pulmonary vessels and activation of the endothelial cells of the pulmonary vasculature by endotoxin or cytokines lead to leukostasis. These events prime the neutrophils for release of their contents upon exposure to the anti-HLA or antineutrophil antibodies. This latter, “two-hit,” model is consistent with the observation that most patients with TRALI are acutely ill prior to the transfusion, and the incidence is higher in patients who have had emergent cardiac surgery or who have received treatment with hematopoietic growth factors (which can also activate neutrophils). The origin of the anti-HLA antibodies and antineutrophil antibodies is important, as they are most likely to be detected in multiparous women. The incidence of anti-HLA antibodies in transfused versus nontransfused men was 1.0% versus 1.7% (no statistically significant difference), whereas the incidence of these antibodies was as high as 32.2% in women with four or more pregnancies.55 In addition, products derived from donors that have caused one episode of TRALI in one patient are more likely to cause the same problem in subsequent recipients. These observations have led to the elimination of the production of FFP from blood donations from multiparous women, and this strategy appears to reduce the incidence of TRALI. The American Red Cross’s hemovigilance program observed an increase of plasma collection from male donors from 55% in 2006 to 95% in 2008.56 Over the same interval, the incidence of TRALI associated with the transfusion of plasma as the only blood product decreased from 15.4 events per 106 plasma units distributed to 4 events per 106 plasma units distributed.56 The noncardiogenic pulmonary edema of TRALI is treated with mechanical ventilation employing low tidal volumes, diuretics, and intravenous corticosteroids, although the value of the latter has been questioned. The person whose blood donation was associated with TRALI should be screened for anti-HLA and antineutrophil antibodies, and the affected patient should not receive products from this particular donor. Patients who have been multiply transfused or have been pregnant may develop alloantibodies against the “minor” blood group antigen such a Kell, Kidd, Duffy, or Lutheran. These antibodies fall to undetectable levels over months or years, so that they may not be identified by the routine antibody screen prior to transfusion of subsequent units of blood. Should the newly transfused RBCs contain one (or more) of these antigens, they will generate an anamnestic response producing alloantibodies that will bind to the transfused RBCs. The patient will present with a subacute decrease of hemoglobin concentration, which may be mistaken for occult hemorrhage. Laboratory evaluation will show a slight rise of indirect bilirubin, a decrease of haptoglobin, and a positive direct antiglobulin test. Often the anemia is mild, and additional blood transfusions are not necessary; however, should patients need additional units of RBCs, they will need to be negative for the mismatched minor blood group antigen. The availability of blood components has enabled surgeons to support patients during operative procedures and has facilitated good clinical outcomes of life-threatening conditions. The appropriate use of blood products requires an understanding of their sources, their limitations, and their potential toxicities. This knowledge needs to be combined with the best available clinical evidence to optimize the benefit and reduce the risk of treatment with blood products. KEY POINTS • Blood product replacement needs to be guided by the clinical situation and not by adhering to arbitrary criteria of hemoglobin, platelet count, or coagulation parameters. • Mild abnormalities of the INR (< 1.7) are usually not associated with clinically significant depletion of coagulation factors. Factor replacement therapy is usually not necessary. • No significant advantages have been identified for directed donor blood products. • Rigorous attention to patient identification and specimen labeling is essential for the safe administration of blood products.
Blood Replacement
Collection, Processing and Storage of Donated Blood
Leukodepletion and Irradiation
Platelet Donation
Red Blood Cell Transfusions
Platelet Transfusion
Autologous and Directed Donation
Directed Donations
Blood Conservation Strategies in the Treatment of Jehovah’s Witnesses
Blood Substitutes
Plasma Components
Fresh Frozen Plasma
Prothrombin Complex Concentrates
Recombinant Factor VII
Adverse Reactions to Blood Products
Hemolytic Transfusion Reactions
Febrile Nonhemolytic Transfusion Reaction
Transfusion-Associated Lung Injury
Delayed Hemolytic Transfusion Reactions
Conclusion
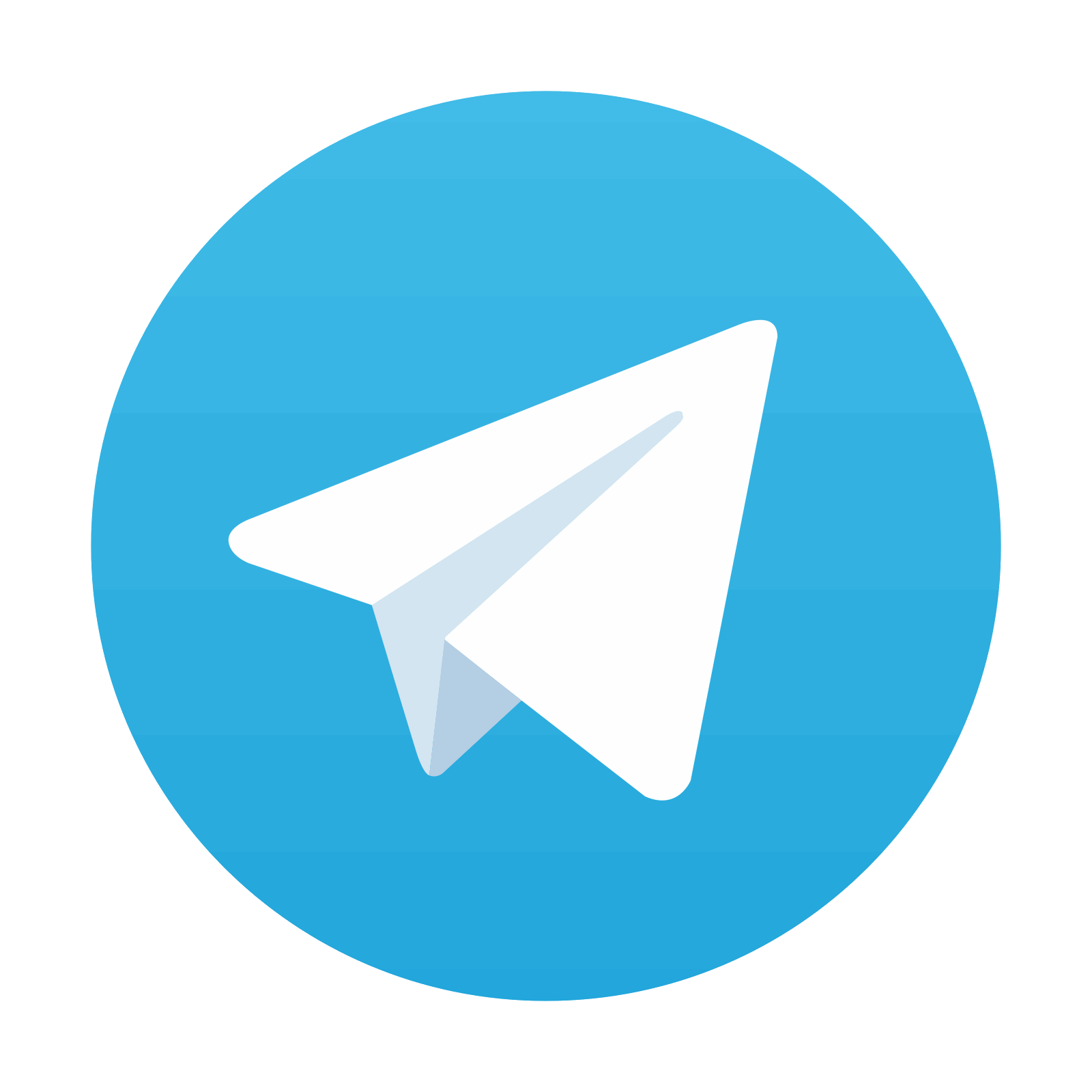