Bone Graft Alternatives in Spinal Surgery
Alberto Di Martino
Jeff S. Silber
Alexander R. Vaccaro
The accomplishment of a successful spinal fusion depends on the stability of the fusion bed, the viability of the local blood supply, and the responsiveness of the cellular environment to osteoinductive and osteoconductive elements. The prospect of a successful fusion depends on four distinct processes: osteogenesis, osteoinduction, osteoconduction, and stability, or lack of motion. Osteogenesis describes the process of new bone formation occurring at a cellular level and implies the recruitment of osteoprogenitor stem cells. Osteoinduction is the cytokine-mediated recruitment and differentiation of various cell types essential for new bone formation; an osteoinductive material must contain one or more growth factors that can induce de novo ectopic bone formation through differentiation of progenitor cells into osteoblasts. Osteoconduction is defined as the apposition of growing bone to the three-dimensional surface of the graft structural scaffold with the graft providing the framework for the ingrowth of the tissue.
Autogenous bone graft, due to its intrinsic osteogenic, osteoinductive, and osteoconductive properties, is an optimal graft source for a spinal fusion. In addition, modern surgical techniques provide early stability through the use of spinal instrumentation; nonetheless, long-term stability is dependent on the development of a solid biologic fusion.
PRINCIPLES OF BONE GRAFTING IN SPINAL FUSION
The local host environment is of critical importance in obtaining a solid spinal fusion. The presence of an adequate blood supply is essential. Disruption of the blood supply can be minimized during surgery through careful surgical manipulation of the surrounding bony and soft tissue elements. Experimental models of posterolateral intertransverse fusions demonstrate that blood supply to the graft arises from the decorticated transverse processes; inadequate decortication leads to failure of the graft to incorporate and achieve fusion (1). Preserving an intact blood supply maintains oxygen tension as well as the nutrient levels; these are necessary to preserve cellular synthetic activity, promote neovascularization, and allow migration of cells into the developing fusion mass.
Systemic illnesses such as diabetes or chronic renal failure can also have an adverse impact on fusion rates; this mechanism is supposed to be related mostly to the impairment in vascular ingrowth. Additionally, cigarette smoking, most likely as a result of the effect of nicotine on vascular supply (2), and malnutrition are associated with a general impairment of wound healing and may also have an adverse effect on fusion healing.
Local biomechanical factors are dependent on the type and position of the bone graft applied to the spine. Anterior interbody structural grafts are exposed to local compressive forces. Precise contouring and fit may affect ultimate fusion success or failure. On the contrary, a posterior applied bone graft is placed along the tension side of the spinal column.
Because there are no local compressive stimuli, bone graft incorporation is not as directly affected by local biomechanical factors. The lack of stability of this grafting technique and the presence of excessive strain may lead to inadequate neovascularization into the graft and ultimately failure to attain a successful arthrodesis.
Because there are no local compressive stimuli, bone graft incorporation is not as directly affected by local biomechanical factors. The lack of stability of this grafting technique and the presence of excessive strain may lead to inadequate neovascularization into the graft and ultimately failure to attain a successful arthrodesis.
![]() FIG. 29.1. Osteoconduction: scheme of impeded (A) and complete (B) bone ingrowth through a porous structure. Reprinted, with permission, from Interpore Cross International, Irvine, CA. |
Osteogenic cells responsible for new bone formation are an absolute requirement for a successful fusion. Autograft bone directly provides viable progenitor stem cells and mature osteoblasts. The bone extracellular matrix provided by the autograft as well as the local wound hematoma at the fusion site are sources of osteoinductive factors such as bone morphogenetic proteins (BMPs). These play a critical role in the formation and maturation of new bone and stimulate the recruitment of additional osteogenic cells. Many growth factors (GFs) are involved in the process of osteogenesis; these include plateletderived growth factor (PDGF), transforming growth factor beta (TGF-β), and vascular endothelial growth factor (VEGF), but BMPs are the only known factors able to induce the entire process of new bone formation (3).
Osteoconductive substitutes may be used as scaffolds to deliver bone growth factors. These materials facilitate bone formation but do not contain natural growth factors that can attract osteoblast precursor cells and initiate osteoblast differentiation. They include collagen, ceramics, polymers, coral, and allograft bone, and they function similarly to the native collagen and hydroxyapatite of autograft bone, providing a lattice within the developing fusion mass for new blood vessels and migrating stem cells (4). Osteoconductivity of a graft material is a function of its geometric and biomechanical properties and of the similarity of the graft to the native bone. In particular, pore size and three-dimensional interconnectivity of the host and donor tissue must be closely matched; this allows the migration of osteoblastic precursor cells and the ingrowth of new bone (Fig. 29.1) (4). The graft also has to be biocompatible to minimize interference with bone induction from an autoimmune inflammatory reaction (5).
AUTOGRAFT AND ALLOGRAFT BONE
The term autograft implies that a piece of bone is harvested from one donor anatomical site and then transplanted to a receptor site in the same individual. Autograft sources include the fibula, ribs, posterior spinal elements, and tibia, although the gold standard for the majority of spinal procedures is autograft taken from the iliac crest. The latter contains osteoblasts and mesenchymal osteogenic precursor cells with osteogenic potential; moreover, it has osteoconductive and osteoinductive properties. A bone allograft is defined as a piece of bone transferred between individuals of the same species.
As demonstrated by Goldberg and Stevenson (6), the incorporation of a bone graft occurs in five distinct stages. Initially, autograft and allograft evoke an acute inflammatory response followed, in 2 weeks, by granulation tissue deposition and activation of osteoblastic activity due to osteoinduction. Vascularization and continued osteoinduction
then occurs; it is during these stages of incorporation that the host’s immune system becomes sensitized to donor antigens if allograft is used. After this stage, the graft’s three-dimensional lattice acts through osteoconduction to promote infiltration of osteoprogenitor cells within its boundaries. This phase lasts from several months for trabecular grafts to years for cortical grafts. Over time, the voids between the graft and host bone are bridged by new bone. Concurrently, remodeling of the graft occurs through local mechanical stimuli (6). Lastly, segmental stiffness increases as new bone is deposited and remodeled onto the osteoconductive matrix of the graft.
then occurs; it is during these stages of incorporation that the host’s immune system becomes sensitized to donor antigens if allograft is used. After this stage, the graft’s three-dimensional lattice acts through osteoconduction to promote infiltration of osteoprogenitor cells within its boundaries. This phase lasts from several months for trabecular grafts to years for cortical grafts. Over time, the voids between the graft and host bone are bridged by new bone. Concurrently, remodeling of the graft occurs through local mechanical stimuli (6). Lastly, segmental stiffness increases as new bone is deposited and remodeled onto the osteoconductive matrix of the graft.
In general, as compared to autograft, allograft bone incorporates more slowly and less completely, which may be a manifestation of the host’s immune response to Class I and II major histocompatibility complex antigens (HLA) contained in the allograft. Experimental allograft bone grafting studies have demonstrated that HLA-matched allografts incorporate in a fashion closely resembling incorporation of autograft bone (7).
The use of allograft bone in spine surgery has increased greatly as a result of improved procurement, preparation, and storage methods. Allograft bone is available in different forms to match the surgeon’s needs in spinal applications (Fig. 29.2). It is both osteoconductive and weakly osteoinductive, the degree of osteoinductivity being directly related to the method of preparation and sterilization of the allograft bone. The processing consists of removal of cells and extraneous debris from the allograft bone, thus minimizing the risk of infection as well as the immunogenicity (8). The downside of this process is a dampening of the osteogenic potential of the allograft tissue.
Allograft preparations are available clinically as either fresh frozen or freeze dried. Both processes start with graft cooling to -70°C. Freeze-dried specimens undergo an additional lyophilization step that destroys any remaining viral DNA. Unfortunately, this process often denatures contained BMPs. Manufacturers of particular demineralized bone matrix (DBM) products that use freeze-dry processing have noted preservation of osteoinductive capabilities resulting from the presence of active BMPs. The most commonly expressed concern among patients who are intended to receive an allograft is the potential that the bone graft will transmit infection to them. Studies suggest the risk of human immunodeficiency virus (HIV) infection to be about 1 per 1.6 million procedures of all allograft bone grafting. The risk of hepatitis B or C transmission is less with freeze-dried than with fresh-frozen allografts (9).
Healing of allograft bone to native bone is partially dependent upon its anatomical placement within the spine. Compared to autograft used in anterior column applications,
allograft bone used in posterior or posterolateral position is associated with reduced fusion rates. In this position, allograft is associated with more extensive resorption, slower incorporation rates, and increased infection risk compared with autologous bone graft (10,11,12).
allograft bone used in posterior or posterolateral position is associated with reduced fusion rates. In this position, allograft is associated with more extensive resorption, slower incorporation rates, and increased infection risk compared with autologous bone graft (10,11,12).
A recent literature review on spinal fusion trends for the treatment of lumbar degenerative disk disease over the last 20 years revealed that iliac crest autograft was the most common graft source utilized, with reported fusion rates of 87%. Allograft fusion alone was successful in 86% of cases, being more frequently used as an interbody graft compared to autograft. Combined autogenous/allogenous grafts resulted in overall fusion rates of 86% (13). Therefore, depending on the clinical situation, an acceptable fusion rate may be achieved using an allograft source alone such as, in a nonsmoker, a single-level anterior cervical discectomy and fusion followed by anterior cervical plating. In this clinical scenario, the morbidity of autograft harvest can often be avoided without compromising outcomes (7,14,15,16,17).
The principal disadvantage of using autograft bone remains the morbidity associated with graft harvest. In fact, in anterior cervical discectomy and fusion procedures, complications related to the graft harvesting site occur more commonly than complications related to the anterior cervical fusion. Incidence of donor-site complication ranges from 4% to 49%, the major complications associated with graft harvest procedures being chronic pain and infection, wound drainage, hematoma formation, pelvic ring destabilization, formation of enterocutaneous fistula, neurosensory loss, gait disturbance, and bowel herniation (8).
The morbidities associated with bone graft harvest have led to the development of numerous biologic and synthetic graft alternatives, including allograft machined implants, demineralized bone matrix, recombinant bone growth factors, and ceramics. These alternatives can be used alone, in combination with one another, with autologous bone marrow, or together with autologous bone graft as bone graft extenders.
BONE MARROW ASPIRATE AND GROWTH FACTORS
Bone marrow aspirate represents an important source of a population of pluripotential progenitor cells, referred to as mesenchymal stem cells, capable of differentiation into cells able to produce bone, cartilage, tendon, and muscle. The percentage of stem cells among nucleated cells varies according to age, decreasing in the bone marrow of elderly patients (18). Stem cell concentration is 1 in 50,000 nucleated cells in young patients and becomes 1 in 2 million in the elderly. Centrifugation of the marrow aspirate allows increasing the relative concentration of stem cells (19).
The procedure of bone marrow aspiration (BMA) from the iliac crest is associated with minimal morbidity; once harvested, the bone marrow can be used as an adjunct to grafting procedures for spinal fusion (Fig. 29.3). Adding bone extracts containing BMPs to the BMA enhances its potential usefulness. Currently, BMA is used clinically as an adjunct to various autograft alternatives, thus providing the osteogenic potential to the osteoinductive and osteoconductive properties of the grafting material.
As noted above, the use of autologous marrow is limited by the relatively low osteoprogenitor cell frequency and number in the starting marrow population. However, a number of groups have shown that human adipose tissue is also a source of osteoprogenitor cells (20,21,22,23,24). Unlike marrow, adipose tissue can be obtained in relatively large quantities with minimal morbidity. In a recent study, Cowan et al. demonstrated the ability of cells derived from murine adipose tissue seeded onto an apatite-coated scaffold to
promote healing in a critical-size (4-mm) calvarial defect in mice (25). The authors also noted that the cells derived from adipose tissue reached confluence approximately 7 times faster than marrow-derived cells (2-3 days compared to 2-3 weeks). Further, De Ugarte et al. (26) compared adipose-derived and marrow-derived stem cells and showed that adipose cells grew approximately 20-fold more effectively than those from marrow of the same donor. Thus, adipose tissue may represent a superior source of osteoprogenitor cells for orthopedic applications.
promote healing in a critical-size (4-mm) calvarial defect in mice (25). The authors also noted that the cells derived from adipose tissue reached confluence approximately 7 times faster than marrow-derived cells (2-3 days compared to 2-3 weeks). Further, De Ugarte et al. (26) compared adipose-derived and marrow-derived stem cells and showed that adipose cells grew approximately 20-fold more effectively than those from marrow of the same donor. Thus, adipose tissue may represent a superior source of osteoprogenitor cells for orthopedic applications.
![]() FIG. 29.3. Intraoperative bone marrow aspiration from the iliac crest. Reprinted, with permission, from Orthovita, Malvern, PA. |
Another fusion enhancement strategy is the addition of growth factors as a bone graft promoter. Following injury, white blood cells and platelets collect at the site of insult and form a clot while attracting reparative growth factors. The osteopromotive nature of this clot has been studied extensively by Muschler et al. (27). Using a canine posterior spinal fusion model, they compared fusion rates of cancellous bone matrix (mineralized or demineralized) combined with bone marrow-derived cells containing osteoblastic progenitor cells with and without a bone marrow clot. They found that adding the bone marrow clot to an enriched cell-matrix composite graft resulted in a significant increase in bone graft healing. At present, several commercial products are available that assist in separating out and concentrating specific blood components such as platelets, growth factors, and the leukocyte-rich buffy coat at the time of surgery. These blood products are prepared in the form of a gel and combined with bone grafting materials to be used as a bone graft extender during a fusion procedure.
The local application of platelet-rich plasma (PRP) to promote or enhance the maturation of bone fusion is based on the release of the growth factors present in platelets, such as PDGF, TGF-β (28), and insulin growth factor-I (IGF-I), which have been shown to be involved in the process of osteogenesis. One commercially available service, Autologous Growth Factor (AGF, Biomet, Warsaw, IN, USA), collects a unit of blood through a cell saver and separates it into three parts: red cells, plasma, and a buffy coat. The buffy coat is then removed and ultraconcentrated. This concentrate is then added to thrombin to form a soft or hard gel, which then is added to an osteoconductive carrier or delivered directly to the fusion site (29). Schlegel et al. (30), in a skull defect model in adult pigs, found that animals treated with a combination of PRP and bovine collagen demonstrated new bone growth similar to that found with the use of autologous bone alone. In contrast, Jensen et al. (31) found no benefit in using PRP combined with allograft in a canine proximal humerus defect model. Walsh et al. (32)
recently reported that the combination of autologous bone marrow, buffy coat derived growth factors, and a porous ceramic (Pro-Osteon 500R) used as a graft substitute in an instrumented posterolateral fusion model resulted in a fusion rate similar to autograft bone alone (Fig. 29.4). At present, blood-derived growth factors have found useful applications in maxillofacial and spinal fusion procedures. Further studies are needed to analyze the potential benefits of these techniques in bone healing in the appendicular skeleton.
recently reported that the combination of autologous bone marrow, buffy coat derived growth factors, and a porous ceramic (Pro-Osteon 500R) used as a graft substitute in an instrumented posterolateral fusion model resulted in a fusion rate similar to autograft bone alone (Fig. 29.4). At present, blood-derived growth factors have found useful applications in maxillofacial and spinal fusion procedures. Further studies are needed to analyze the potential benefits of these techniques in bone healing in the appendicular skeleton.
DEMINERALIZED BONE MATRIX
Demineralized bone matrix (DBM) is generally a weakly osteoinductive allograft bone graft extender. The osteoinductive potential of DBM is partly dependent on how it is processed, with some proving experimentally to be more osteoinductive than others (33). DBM is produced via removal of the mineral content of bone through mild acid extraction; this process leaves an organic product made predominantly by BMPs, collagen, and various noncollagenous proteins (18). The trabecular structure of the original tissue is retained and can serve as a biologic scaffold; however, the original structural strength contributed by the bone mineral is no longer present. The naturally occurring BMPs present in DBM stimulate mesenchymal stem cells to differentiate into chondrocytes and osteoblasts, thus exerting their osteoinductive effect (29). Extensive processing makes DBM the least immunogenic of all currently available allograft bone products. DBM has reported excellent results in stable and well-supported skeletal defects in animal studies, as well as in the treatment of giant cell tumors of bone in humans (34,35). In addition, Glowacki and Mulliken (36) found that DBM and bone marrow composite grafts were comparable to autogenous iliac crest bone graft in some arthrodesis procedures.
A variety of DBM products are commercially available. One of the most intensively studied and used product is Grafton Demineralized Bone Matrix (Osteotech, Eatontown, NJ, USA). This allograft material is considered banked human tissue by US regulatory authorities: it consists of DBM in a glycerol carrier (18). Grafton DBM is available in five different forms (Fig. 29.5): Gel, which consists of particulate DBM; Grafton DBM Putty, which contains DBM in fiber form (fibers have been shown to be more osteoconductive than particles (37); Grafton DBM Flex, which is made of nonwoven sheets of DBM fibers; Grafton DBM Matrix, a preformed fiber material designed for specific spinal applications; and Grafton Plus DBM, a paste product designed to be moldable and irrigable. Grafton has proven to be osteoinductive in animal models, and preclinical studies have
yielded favorable results when Grafton was used either alone or as an autograft extender. A recently reported clinical trial of 120 patients who underwent a posterolateral instrumented lumbar spine fusion using autograft alone on one side and Grafton DBM Gel as an autograft extender on the other side found similar fusion results for both sides (38,39).
yielded favorable results when Grafton was used either alone or as an autograft extender. A recently reported clinical trial of 120 patients who underwent a posterolateral instrumented lumbar spine fusion using autograft alone on one side and Grafton DBM Gel as an autograft extender on the other side found similar fusion results for both sides (38,39).
Other commercially available DBM preparations use a variety of carriers such as calcium sulfate (Allomatrix, Wright Medical Technology, Memphis, TN, USA); hyaluronic acid (DBX, Synthes, Paoli, PA, USA); porcine collagen (Osteofil, Regeneration Technologies Inc., Alachua, FL, USA) (40); cortical bone chips (Opteform, Regeneration Technologies, Inc., Alachua, FL, USA); and poloxamer excipient (Dynagraft, GenSci Regeneration Sciences, Inc., Irvine, CA, USA).
CERAMICS
Ceramics are synthetic bone graft alternatives. The biggest advantages of their use are their limitless availability and the absence of disease transmission. Ceramics are not derived from bone and are purely osteoconductive without possessing osteogenic or osteoinductive properties. The two-dimensional surface of ceramics provides the scaffold for adherence and migration of bone-forming cells, but it is their three-dimensional microarchitecture (pore diameter and void interconnectedness) that is the major determinant of the rate of graft incorporation and remodeling. The optimal pore size for osteoconduction ranges from 150 µm to 500 µm (41). These highly porous, low-density implants offer a large surface area for nutrient access, neovascularization, and bony ingrowth (9).
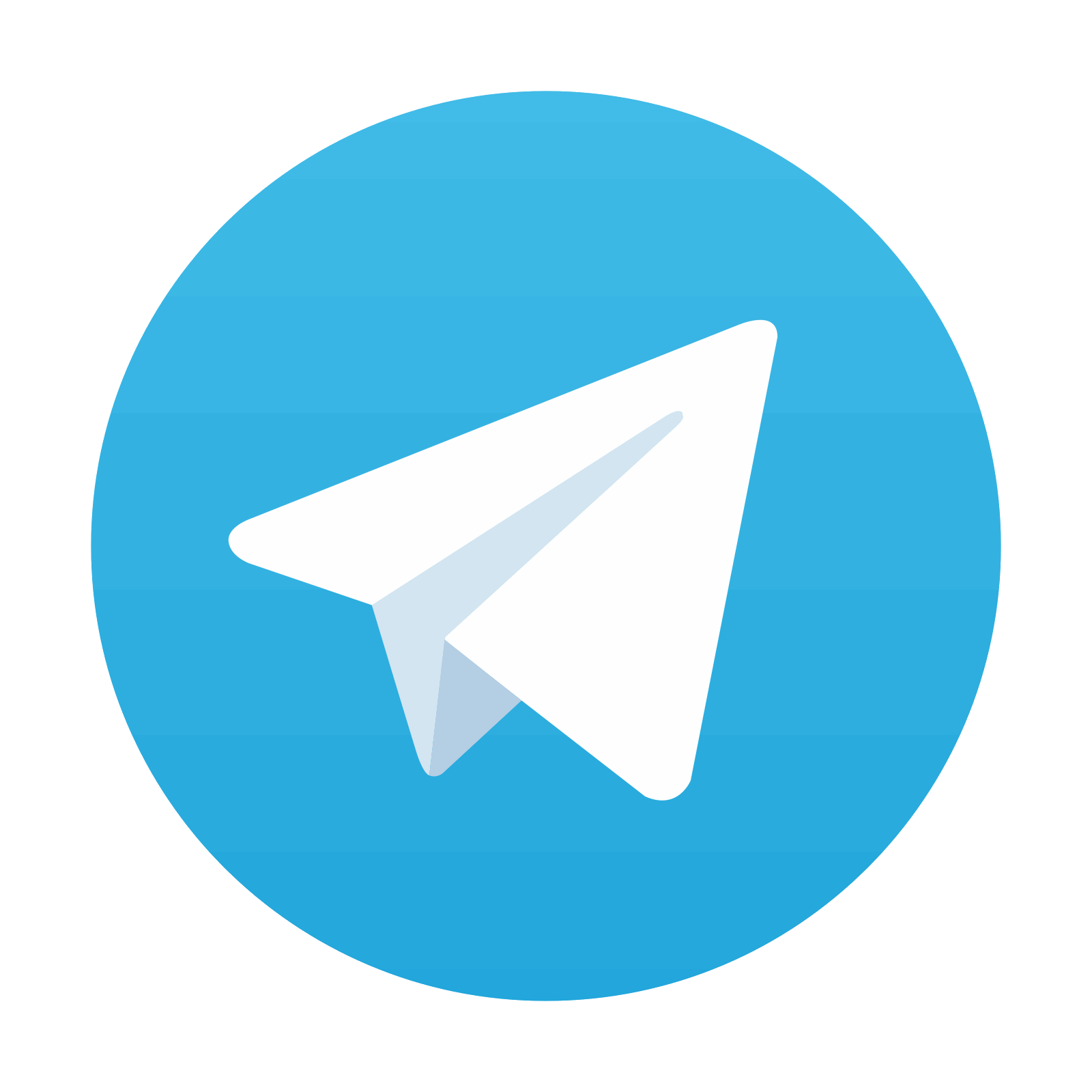
Stay updated, free articles. Join our Telegram channel

Full access? Get Clinical Tree
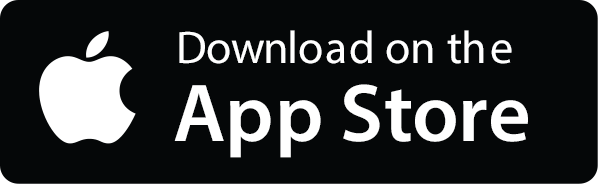
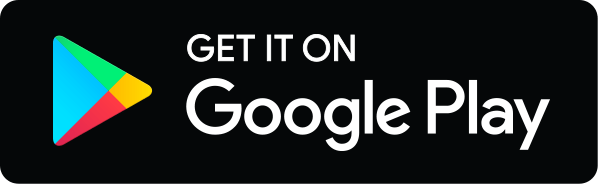