Fig. 30.1
An example of a cerebellar metastasis treated with 18 Gy in a single fraction, and the 18 Gy isodose line in yellow conforms around the target. Despite a small solitary lesion, prior to SRS this patient’s whole head would have been radiated and on the right is a typical whole brain radiation portal
For years, the Recursive Partitioning Analysis (RPA) scoring system was the standard by which patients were prognosticated to guide treatment options and patients were grouped simply into three RPA classes based on performance status, stability of extracranial disease, and age with resultant median survival times of 7 months, 3–6 months, and, ~2 months (Gaspar et al. 1997). However, in contemporary oncology practice, we do not infrequently observe that patients with brain metastases may have an extended survival of several years post-diagnosis, and newer and more accurate prognostic indices have been developed, including the Graded Prognostic Assessment (GPA) and diagnosis-specific GPA (DS-GPA) scoring systems, and these now even incorporate tumour molecular profiles (Sperduto et al. 2008, 2010). As a result, we are now better equipped to select patients for focal stereotactic radiosurgery (SRS) (Fig. 30.1) and/or surgery, where the aim is to maximize local control rather than to simply temporize brain control with WBRT. Stereotactic radiosurgery is defined as the use of 1–5 fractions of high dose radiation delivered to a well-defined three dimensional target using a radiation delivery system that permits sub-millimeter delivery accuracy. An example of a SRS dose distribution is shown in Fig. 30.1.
Over the last 5 years, there has been a major shift in the management of brain metastases as we now have the randomized data and meta-analyses to support SRS alone or in conjunction with WBRT for patients presenting with up to four metastases (Aoyama et al. 2006; Kocher et al. 2010; Chang et al. 2009; Tsao et al. 2012a, 2012b). The success of SRS has broadened its application to even the postoperative patient where historical dogma favoured postoperative WBRT without consideration of focal therapy (Roberge et al. 2012). This review will summarize the latest evidence formulating today’s standards of care in the clinical management of brain metastasis, and detail recent technological advances specific to SRS.
Clinical Outcomes and Standards of Care Based on Randomized Controlled Trials and Meta-Analysis
Steroid therapy is the first line of management for symptomatic brain metastasis, and dexamethasone is the drug of choice given its relatively low mineralcorticoid effects. Steroids often improve symptoms related to cerebral edema. Steroid dose should be tapered as tolerated post CNS directed therapy.
Surgical resection, WBRT, SRS, or combinations of these therapeutic options are the standard CNS directed therapies for brain metastasis (Sahgal et al. 2012). Although some controversy exists as to the optimal therapeutic choice or choices, the treatment strategy has generally been dictated by the number of brain lesions at presentation and patient- and cancer-related prognostic factors. Currently available randomized trial data do not deal with poor-prognosis patients, patients with low KPS, or patients with a large number of brain mets, but they do deal with a select patient cohort with high performance status, relatively controlled low burden of extracranial disease, and a limited number of brain lesions. The following discussion is focused on evaluating treatment options with a focus on only these randomized trial data.
The Role of Surgery in the Management of Brain Metastasis
A practice-altering phase III trial randomized 54 patients with a Karnofsky Performance Status (KPS) over 70 and a solitary brain lesion (no other site of metastatic disease) to surgical resection vs. brain biopsy, in either case followed by WBRT (Patchell et al. 1990). All outcomes favoured the surgical group, including recurrence at original site of disease (20 % vs. 50 %), time to recurrence, median survival (10 vs. 4.2 months), time to death from neurological cause, and preservation of performance status (8.7 vs. 1.8 months). These findings were essentially confirmed by a second randomized trial (Vecht et al. 1993; Noordijk et al. 1994). In the third trial evaluating the same question, a survival benefit was not observed largely due to the selection criteria that allowed patients with poorer performance status to be included (Mintz and Cairncross 1998). Accordingly, surgical resection in the setting of a solitary site of brain disease is considered standard of care. What is not known is if SRS can replace surgery in these patients without compromising a potential gain in overall survival, and this will be discussed further in this chapter. However, for large metastases (typically >3 cm) and those resulting in significant edema and neurologic deficit, surgery is the best option to not only remove the tumour burden but relieve symptoms (Table 30.1).
Table 30.1
Summary of the phase III randomized controlled studies evaluation SRS and WBRT
RCT | % Single brain metastases | Performance status | Tumour size | Primary endpoint | Local control | Distant control | OS |
---|---|---|---|---|---|---|---|
Aoyama: SRS (n = 67) vs. WBRT + SRS (n = 65) | 49 % vs. 48 % | 52 % KPS 90–100 vs. 66 % KPS 90–100 | Median: 1.3 cm vs. 1.4 cm | Brain tumour recurrence | 72.5 % vs. 88.7 % at 1 yr (p = 0.002) | 36.3 % vs. 58.5 % at 1 yr (p = 0.003) | 28.4 % vs. 38.5 % at 1 yr (p = 0.42) |
Chang: SRS (n = 30) vs. WBRT + SRS (n = 28) | 60 % vs. 54 % | 100 % KPS ≥70 (each arm) | Median TV: 1.4 cc vs. 2.3 cc | Neurocognition: HVLT scores at 4 months | 67 % vs. 100 % at 1 yr (p = 0.012) | 45 % vs. 73 % at 1 yr (p = 0.02) | 63 % vs. 21 % at 1 yr (p = 0.003) |
Kocher: SRS (n = 100) vs. WBRT + SRS (n = 99) | 68 % vs. 66 % | 100 % WHO status 0–2 (in each arm) | Median: 2.0 cm vs. 1.8 cm | Duration of functional independence | 69 % vs. 81 % at 2 yr (p = 0.008) | 52 % vs. 67 % at 2 yr (p = 0.023) | Median: 10.9mo vs. 10.7mo (NSIG) |
Kondziolka: WBRT + SRS (n = 13) vs. WBRT (n = 14) | 0 % | 100 % KPS ≥70 (each arm) | All tumors <2.5 cm | Local Control | 92 % vs. 0 % at 1 yr (p = 0.0016) | NR | Median: 11mo vs. 7.5mo (NSIG) |
Andrews: WBRT + SRS (n = 164) vs. WBRT (n = 167) | 56 % vs. 56 % | 57 % KPS 90–100 vs. 63 % KPS 90–100 | 50.5 % ≤2 cm vs. 59 % ≤2 cm | Overall Survival | 82 % vs. 71 % at 1 yr (p = 0.01) | NR | Median Single mets:6.5mo vs. 4.9mo (p = 0.04) Multiple mets: NSIG |
WBRT in the Postoperative Patient
Patchell et al. (1998) also reported a phase III study evaluating the role of postoperative WBRT by randomizing 95 patients with a solitary brain lesion treated with surgical resection to WBRT vs. observation alone. Postoperative WBRT was found to significantly reduce both the rate of local recurrence (10 % vs. 46 %) and distant brain failures (14 % vs. 37 %), and deaths due to neurological causes (14 % vs. 44 %). However, overall survival and duration of functional independence were similar between the two cohorts. This study concluded that postoperative WBRT was the standard of care.
A more recent randomized trial published by Kocher et al. (2010) evaluated the role of WBRT vs. observation following either surgery or SRS. In the surgical cohort (160 patients), outcomes were similar to those observed in the Patchel et al. (1990) trial. Postoperative WBRT decreased intracranial disease progression at 24 months (31 % vs. 54 %, respectively) and the risk of neurological death (25 % vs. 43 %), while not impacting overall survival or preservation of performance status.
Stereotactic Radiosurgery as an Alternative to Surgery
As of yet, no completed randomized trials have directly compared surgical resection to SRS. The study by Muacevic et al. (2008) came closest, and randomized patients with a single resectable brain metastasis measuring 3 cm or smaller to microsurgery plus WBRT vs. SRS alone. This trial was closed early due to poor accrual, but the results of 64 randomized patients were reported in 2008 with overall survival, neurological death rates, and local control appearing similar in the two groups. The patients randomized to SRS alone were observed to have more distant brain recurrences, although this difference was no longer observed when accounting for salvage SRS. As compared to surgical resection and WBRT, SRS alone was also associated with a shorter hospital stay, less frequent and shorter use of steroids, decreased CNS toxicity, and improved quality of life scores at 6 weeks post therapy (this effect was no longer observed at 6 months). Coupled with the favourable local control results observed in the randomized studies evaluating SRS alone (summarized in the following sections), most believe that SRS is a reasonable alternative to surgery except for large lesions.
WBRT with or Without SRS
In the early years of SRS it was recognized that local control may not be optimal with WBRT alone. Therefore, it was important to clarify the role of SRS as a boost to WBRT in patients preseting with up to three metastases. Andrews et al. (2004) published the landmark trial based on 331 patients randomized to WBRT plus or minus SRS as a boost. Stereotactic radiosurgery was shown to improve local control, KPS, and for patients with a single brain metastasis, a statistically significant improvement in OS (6.5 months with SRS + WBRT vs. 4.9 months WBRT alone). In addition, a trend to support a median survival advantage for RPA class one patients (11.6 vs. 9.6 months), lung histology (5.9 vs. 3.9 months), and tumour size >2 cm (6.5 vs. 5.3 months) was observed in sub-group analysis.
An earlier but small study of 27 patients with 2–4 brain metastasis was published (Kondziolka et al. 1999). Patients were randomized to WBRT alone vs. WBRT and SRS. Local failure was 100 % at 1 year in patients treated with WBRT alone, and only 8 % progressed in those boosted with SRS. A statistically non-significant OS benefit was observed in the SRS group (MS 7.5 vs. 11 months). This study is controversial in that 100 % local failure in the WBRT alone cohort may reflect selection bias or bias associated with a small sample size.
A meta-analysis of these two studies concluded there was no difference in OS, with a hazard ratio (HR) of 1.63 (95 % CI 0.72–3.69, p = 0.24), while LC significantly favoured WBRT plus SRS with a HR of 2.88 (95 % CI 1.63–5.08, p = 0.003) (Tsao et al. 2012a).
SRS with or Without WBRT
The next question was the role of SRS alone while deferring WBRT to salvage therapy upon brain progression. This strategy was driven largely over concerns related to WBRT-associated neurotoxicity. Three randomized studies have been reported evaluating SRS alone to WBRT plus SRS as a boost in patients with up to 3–4 metastases (Aoyama et al. 2006; Kocher et al. 2010; Chang et al. 2009).
Aoyama et al. (2006) published the first study based on 132 patients with 1–4 lesions randomized to SRS alone vs. WBRT plus SRS boost. Whole brain radiotherapy was observed to reduce the risk of developing new brain metastasis (63.7 % vs. 41.5 %) and improve the 1-year local control rate (72.5 % vs. 88.7 %); however, no difference in OS (8.0 vs. 7.5 months). Neurocognitive function changes were tested using the mini-mental status examination (MMSE) with no significant differences observed bewteen the two arms. However, neuropsychologists do not recognize the MMSE as an adequate test for assessing those neurocognitive functions most likely to be damaged by radiation.
In order to study neurocognitive changes associated with WBRT, a trial evaluating 58 patients with 1–3 metastases randomizing to SRS alone vs. WBRT plus SRS with the primary endpoint of neurocognition changes at 4 months (Chang et al. 2009). They used a validated neurocognitive outcome tool, namely the Hopkins Verbal Learning Test (HVLT), as opposed to the insensitive MMSE previously described in the Aoyama et al. (2006) RCT. Chang et al. (2009) reported worse neurocognitive function with respect to memory at 4 months following treatment in the WBRT plus SRS cohort. This impairment in memory was observed despite the better local and distant brain tumour control seen in that cohort. Although there were several limitations in this study that could have also contributed to the poorer neurocognitive outcomes in the WBRT group, such as greater extra-cranial and intra-cranial disease burden and more deaths at the 4 month interval, this study was the first to provide level 1 evidence that WBRT independently and adversely impacts neurocognition. A larger study by Brown et al. (2009) is currently accruing with neurocognition as the primary endpoint which will hopefully provide the much needed confirmatory evidence to support or refute the Chang study.
Nevertheless, Chang’s conclusion is supported by a recent randomized trial of prophylactic cranial WBRT (PC-WBRT) in patients with non-small cell lung cancer (NSCLC) (Sun et al. 2011). Based on HVLT assessments, PC-WBRT was shown to adversely impact memory both at 6 months and 1 year despite a reduction in the risk of development of brain metastases in the PC-WBRT group (Sun et al. 2011). What both these RCTs suggest, is that recurrence is not the only cause of neurocognitive decline, but WBRT independently impairs memory function.
A recent meta-analysis of the Aoyama and Chang study by Tsao et al. (2012a), found no difference in OS between the two arms with a HR of 0.98 (95 % CI 0.71–1.35, p = 0.88). Therefore, it is unlikely that SRS alone adversely impacts survival. The outcomes for local control and distant brain control from these two studies were pooled with the SRS alone vs. SRS plus WBRT arms within the Kocher et al. (2010) RCT (this was not possible for survival analysis due to the way in which the data were reported). It was observed that the addition of WBRT improved local control and distant brain control vs. SRS alone. The HR for local control was 2.61 (95 % CI 1.68–4.06, p < 0.0001) and for distant brain control 2.15 (95 % CI 1.55–2.99, p < 0.00001) (Tsao et al. 2012b). If the aim is to preserve neurocognition, then SRS alone is the preferred therapeutic option. If the patient is more focused on brain control and less concerned with neurocognition, then WBRT plus SRS is the preferred strategy. In either case, close imaging-based follow-up is required, to provide salvage therapy as needed.
The Evolving Role of SRS to the Resection Cavity as an Alternative to WBRT in the Postoperative Patient
Due to concerns of WBRT-related neurotoxicity, some investigators applied SRS to the postoperative surgical bed or surgical cavity, as opposed to treating with WBRT (Roberge and Souhami 2010; Roberge et al. 2012). A review by Roberge et al. (2012) analyzed 492 postoperative patients treated with SRS to the resection cavity in non-randomized trials. Crude LC was 79 %, and the risk of radiation necrosis was estimated at ~5 %. Randomized trials are underway to compare WBRT to postoperative cavity radiation, and clearly this is the current question that needs an answer (Roberge et al. 2012).
There are challenges with treating cavities with SRS due to the relatively large size and irregularity of the cavity shape, such that the SRS dose is typically compromised due to the principle of reducing the dose prescribed with increasing volume to repect safety. This has led some centers to treat with focal hypofractionated radiosurgery (3–5 fractions) to minimize risks of normal tissue toxicity. Some preliminary data support a hypofractionated approach both for postoperative patients (Katz et al. 2012) and also for intact metastases (Kim et al. 2011). The ability to deliver hypofractionated SRS is largely due to advances in SRS technology and this will be discussed in the subsequent sections.
SRS Technology
Gamma Knife Technology
Intracranial SRS was pioneered in the 1950s by Sweedish neurosurgeon Lars Leksell, initially as a therapeutic approach for functional brain disorders, and subsequently for tumours. Certainly the largest application of SRS is now in the treatment of brain metastases. Ultimately, Leksell developed a self-contained SRS unit that is now known as the Gamma Knife. Gamma Knife (Eletka AB). Stereotactic radiosurgery is based on localizing and fixing the target in three-dimensions, such that target motion during treatment is negligible. The treatment process begins with the patient’s skull fixed and indexed in three-dimensional space using a stereotactic metal head frame screwed to the outer calvarium.
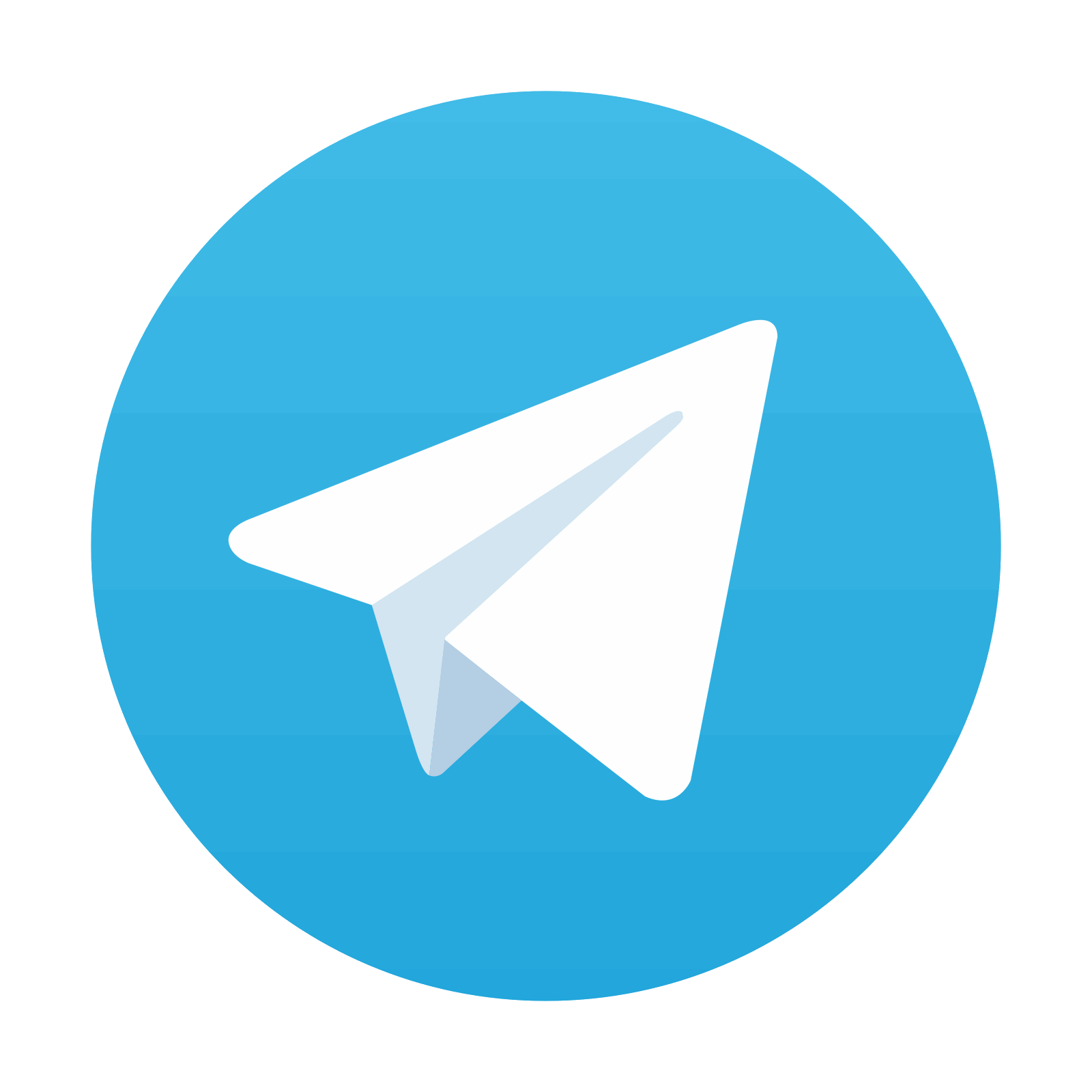
Stay updated, free articles. Join our Telegram channel

Full access? Get Clinical Tree
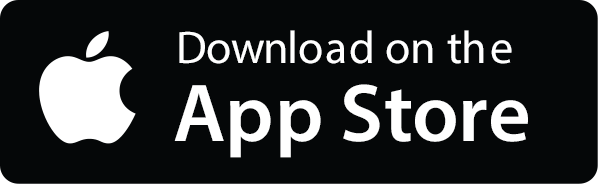
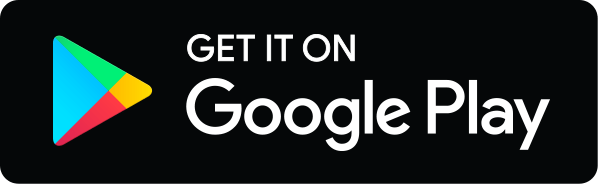