Keywords
brain metastases, small cell lung cancer, placental growth factor, blood–brain barrier, tight junctions, brain endothelial cells
Outline
Introduction 213
Placental Growth Factor 215
Roles of PLGF in SCLC Metastasis to the Brain 216
Levels of PLGF Expression in SCLC Patients 217
Effects of PLGF on SCLC Migration across the Brain Endothelium 219
PLGF-Induced Changes in Tight Junctions between Brain Endothelial Cells 220
Discussion 221
Acknowledgments 224
References
Introduction
Small cell lung cancer (SCLC) is the most aggressive histologic subtype of lung cancer, with a strong predilection for metastasizing early. About 10% of patients with SCLC develop brain metastasis at the time of diagnosis, and the majority of these patients will be symptomatic. The progressive growth of metastasis in the brain is often associated with the terminal stage of the disease, and the prognosis of most patients is consistently poor, with median survival duration of 4–6 months. Additionally, brain metastasis (BM) occurs during the course of the disease in over 50% of SCLC patients surviving 2 years or more ( ). Obviously, it is necessary to prevent BM in SCLC management. Although BM can be surgically removed without producing severe neurologic complications, the prognosis of the disease is still very poor. Therefore, a better understanding of the mechanisms of SCLC metastasis to the brain is important to improve current therapies and design new treatment modalities.
The brain is a common site of metastasis in SCLC. It is important for successful BM formation that SCLC cells migrate across the blood–brain barrier (BBB), which is constituted with the endothelium and the surrounding cells. The unique anatomical structure of BBB isolates central cells from peripheral tissue and maintains highly stable conditions ( ). Owing to the existence of the BBB, the mechanism of SCLC metastasis to the brain is believed to be different from that to other tissues. SCLC cells adhesion to and migration through this barrier require complex molecular interactions, and the detailed understanding of these events may lead the way to target inhibition of BM formation. However, in-depth knowledge about these mechanisms is largely lacking. In many patients, the BBB has failed to block the invasion of metastatic SCLC cells into brain parenchyma, suggesting the presence of some mechanisms that facilitate invasion of cancer cells into the brain parenchyma through the BBB. Moreover, the BBB is breached in metastases as evidenced by magnetic resonance imaging, which shows that more than 70% of SCLC brain metastases have leakage of contrast agent from blood vessels ( ). It is likely that the increased BBB permeability plays a crucial role in the process. However, the detailed mechanism remains a mystery.
A major anatomical feature regulating BBB permeability is the presence of closely associated areas called tight junctions (TJs) between opposing endothelial cells. The TJs are the main components that confer the low paracellular permeability and high electrical resistance. TJs function both as a “zipper” and a “fence” that limit paracellular permeability and are composed of transmembrane proteins, such as claudins and occludin, joining the cytoskeleton via cytoplasmic proteins, such as zonula occludin proteins (ZO-1 and ZO-2). Thus, the regulation of TJ protein expression and/or subcellular distribution plays a key role in the alteration of the BBB permeability.
Our previous studies had clearly indicated that SCLCs could trigger the disassembly of TJs between brain microvascular endothelial cells resulting in the enhancement of the BBB permeability ( ). So, the “opening” of TJs seemed to be a key event in SCLC cell transendothelial migration. Then, how do SCLC cells “open” the TJs between brain endothelium? Recent investigations were beginning to provide insight into how several cytokines and their signaling pathways participate in breast cancer and melanoma cell metastatic colonization in the brain. A variety of the factors were postulated to be involved in altering BBB permeability when these tumor cells metastasized to brain. , performed comparative genome-wide expression analysis on breast cancer cell brain metastatic variants and identified the cyclooxygenase COX2, as a critical mediator of extravasation through the blood–brain barrier; determined that stromal cell-derived factor 1α (SDF-1α) induced blood vessel instability, through increased vascular permeability, resulting in the penetration of breast tumor cells through the human brain microvascular endothelial cells. Overexpression of transforming growth factor-β2 (TGF-β2) in murine B16 melanoma cells induced parenchymal micrometastases but had no effect on the leptomeningeal lesions ( ). It was reported that vascular endothelial growth factor (VEGF) is an important regulator for breast cancer cell migration across the brain endothelial cells ( ). In a brain metastatic variant of MDA-MB-231 cells, there was a significant increase in VEGF production, and inhibition of VEGFR activity significantly reduced brain tumor burden after intracarotid injection in mice ( ). However, some studies demonstrated that VEGF expression was not sufficient for BM production. When lung adenocarcinoma cells (PC14PE6) transfected with antisense VEGF165 were injected into the carotid artery of nude mice, the formation of brain metastases was substantially decreased compared with untransfected cells. However, in lung squamous carcinoma cells (H226) transfected with VEGF121 or VEGF165, no increase in metastasis was observed ( ). Therefore, another vascular permeability factor beside VEGF might facilitate tumor cell migration across the BBB and metastasize to the brain. The alteration of BBB permeability between brain endothelium was an important biological event during SCLC cell transendothelial migration. However, the detailed molecular mechanism which contributes to the pathological process needs to be elucidated.
Placental Growth Factor
Placental Growth Factor in Normal and Tumor Cells
Placental growth factor (PLGF) is the second member of VEGF family. The name refers to the placenta since it was cloned from a human placental cDNA library ( ). The human plgf gene mapped to chromosome 14q24, and was formed by seven exons spanning 13.7 kb, excluding the upstream and downstream regulatory sequences. PLGF is highly expressed in placenta throughout all stages of gestation. It has been proposed to control trophoblast growth and differentiation. In addition, PLGF is expressed at a low level in several other organs including the heart, lung, thyroid, skeletal muscle, and adipose tissue under normal physiologic conditions. The major function of PLGF is as a pro-angiogenic factor for pathologic angiogenesis in adults and is not produced by the majority of normal human tissues. Additionally, PLGF also stimulates leukocyte infiltration, stromal cell migration, and revascularization of ischemic tissues, indicating that PLGF has a biological activity in vivo ( ).
The role of PLGF in tumor progression has been explored extensively in a large body of clinical and experimental research. A prognostic implication of PLGF expression independent of conventional pathologic prognosticators has been demonstrated in a variety of cancers. PLGF expression in tumor tissues has been found to be correlated with tumor stage and patient survival in gastric cancer and colorectal cancer, with tumor stage in lung cancer, with recurrence and metastasis in breast cancer, and even with postoperative early recurrence in hepatocellular carcinoma ( ). More importantly, PLGF levels in plasma and tumors are also correlated with tumor stage, vascularity, recurrence, metastasis, and survival in various tumors. Plasma PLGF levels were upregulated and correlate with tumor grade and survival in patients with renal-cell carcinoma ( ), and the recurrence and survival in patients with colorectal cancer ( ). However, controversial studies addressing the role of PLGF in various models yielded conflicting results. PLGF overexpression enhanced tumor growth in some models but, in others, PLGF paradoxically had an inhibitory effect ( ). Up to now, the significance of this molecule is still a matter of debate. It remains to be determined whether PLGF acts as a positive regulator of cancer growth and metastasis.
In tumor tissues, PLGF is not only produced by malignant cells, but also by endothelial cells, smooth-muscle cells, pericytes, tumor-associated macrophages, and inflammatory cells. Some experimental studies showed that PLGF mediated a number of cellular activities to promote metastasis, including attraction of endothelial cells for establishment of a blood supply and enhanced cellular movement ( ). Notably, recent studies showed that PLGF blockade had no significant effect on primary tumor growth but significantly inhibited melanoma cell extravasation into the lung ( ). Altogether, these observations suggested that PLGF had more versatile roles in cancer pathology besides tumor angiogenesis.
PLGF and Vascular Permeability
PLGF is a homodimeric glycoprotein, structurally and functionally related to VEGF. Exogenous PLGF has been reported to have either an indirect or direct effect on in vitro or in vivo vascular permeability but most studies agree that PLGF enhances VEGF-induced vascular permeability ( ). Some evidence has shown that the absence of PLGF reduced vascular permeability and inflammation in pathological models of neoangiogenesis ( ). Transgenic mice overexpressing PLGF in the skin displayed a marked increase in dermal vascular permeability ( ), while PLGF deficiency resulted in a diminished and abbreviated inflammatory response, together with a reduction of plasma extravasation and edema formation ( ). Recombinant PLGF induced an increase of filtration in isolated cerebral vein of Galen in mice ( ). These findings revealed that PLGF might be a potent vascular permeability factor, but the detailed mechanism was unclear.
Intercellular junctions, including adherens junctions (AJs) and TJs, between endothelial cells control vascular permeability and integrity. There has been no evidence up to now about the effect of PLGF on TJs between endothelial cells, but some evidence has been reported that PLGF mimics VEGF effects to regulate the external blood–retinal barrier function by a direct effect on retinal pigment epithelial cell TJs. Injection of PLGF into the rat eye vitreous induced an opening of the TJs between retinal pigment epithelial cells with subsequent sub-retinal fluid accumulation, retinal edema and cytoplasmic translocation of junction proteins ( ). These findings indicated that PLGF might be involved in the organization of TJs between the endothelium. Although PLGF has been shown to increase vascular permeability in peripheral tissue, whether PLGF affects the cerebral endothelium to enhance BBB permeability is unknown.
Roles of PLGF in SCLC Metastasis to the Brain
Brain metastasis is one of the major causes of morbidity and mortality in patients with SCLC. Therefore, a full understanding of these mechanisms is essential to controlling SCLC brain metastasis. Our previous studies had clearly indicated that the increase of brain endothelial permeability induced by SCLC cells was a key event in tumor cell transendothelial migration. Then, how do SCLC cells induce increased brain endothelial permeability? We pay close attention to the issue.
Levels of PLGF Expression in SCLC Patients
A variety of factors are involved in altering BBB permeability during tumor cell metastasis to brain, such as VEGF, SDF-1α, TGF-β2 and COX-2. So, which molecules contribute to SCLC cells migration across the BBB? To ascertain this question, we first examined the serum levels of several soluble factors in SCLC patients with or without BM. The collection of serum samples from 18 SCLC patients with BM, 27 SCLC patients without BM and 16 normal persons were used for ELISA analysis. The serum levels of PLGF and VEGF were significantly increased in SCLC patients with BM compared to SCLC patients without BM and normal specimens ( Figure 19.1 A), and the serum levels of SDF-1α, TGF-β2 and COX-2 were not elevated in SCLC patients with BM. PLGF is a member of the ‘‘VEGF family” of structurally related growth factors, which shares 42% amino acid sequence identity with VEGF and has a very similar biologic function. It had been reported that VEGF expression was necessary but not sufficient for the production of breast cancer cell BM. In our studies, the serum levels of PLGF and VEGF in SCLC patients with BM were significantly higher than SCLC patients without BM, which gives us a clue that PLGF might be a potential factor associated with BM, especially for SCLC. To determine potential values of serum PLGF for prediction of BM, we further performed the receiver operating curve (ROC) analysis of serum PLGF. For the screening method, a serum PLGF value of>136 ng/mL seemed to be the appropriate cutoff level for the prediction of BM with 77.78% sensitivity, 66.67% specificity, and 59.3% accuracy. The area under the ROC was 0.7562 ( p =0.0039, 95% confidence interval of 0.6013–0.9110), which indicated the potential predictive value of BM. Even though the sensitivity or specificity of PLGF cutoff value was not relatively high, the area under the ROC and the p value indicated the potential promising predictive value. In addition, we compared the ROC of PLGF to VEGF. The area under the ROC of VEGF was 0.6862 ( p =0.03607, 95% confidence interval of 0.5309–0.8416), which indicates the PLGF was more valuable than VEGF to predict BM (see Figure 19.1 B). Next, we sought to determine whether PLGF was increased in the specimens of SCLC BM. We also collected 21 SCLC BM specimens and 39 primary SCLC specimens, which were frequency matched by age and sex. None of the patients had received preoperative neo-adjuvant chemotherapy or radiation therapy. Immunohistochemical staining analysis showed that SCLC cells displayed strong positive staining for PLGF corresponding to the negative staining in no-tumor tissue of the lung. The immunostaining intensity of PLGF in tumor tissues was assessed as PLGF expression levels and was quantified by the integrated optical density (IOD) values. The results showed that the PLGF levels in SCLC BM specimens were significantly higher than primary SCLC specimens, which were consistent with the data of serum analysis ( Figure 19.2 ). These clinical data suggested that PLGF was a correlative factor with SCLC BM.

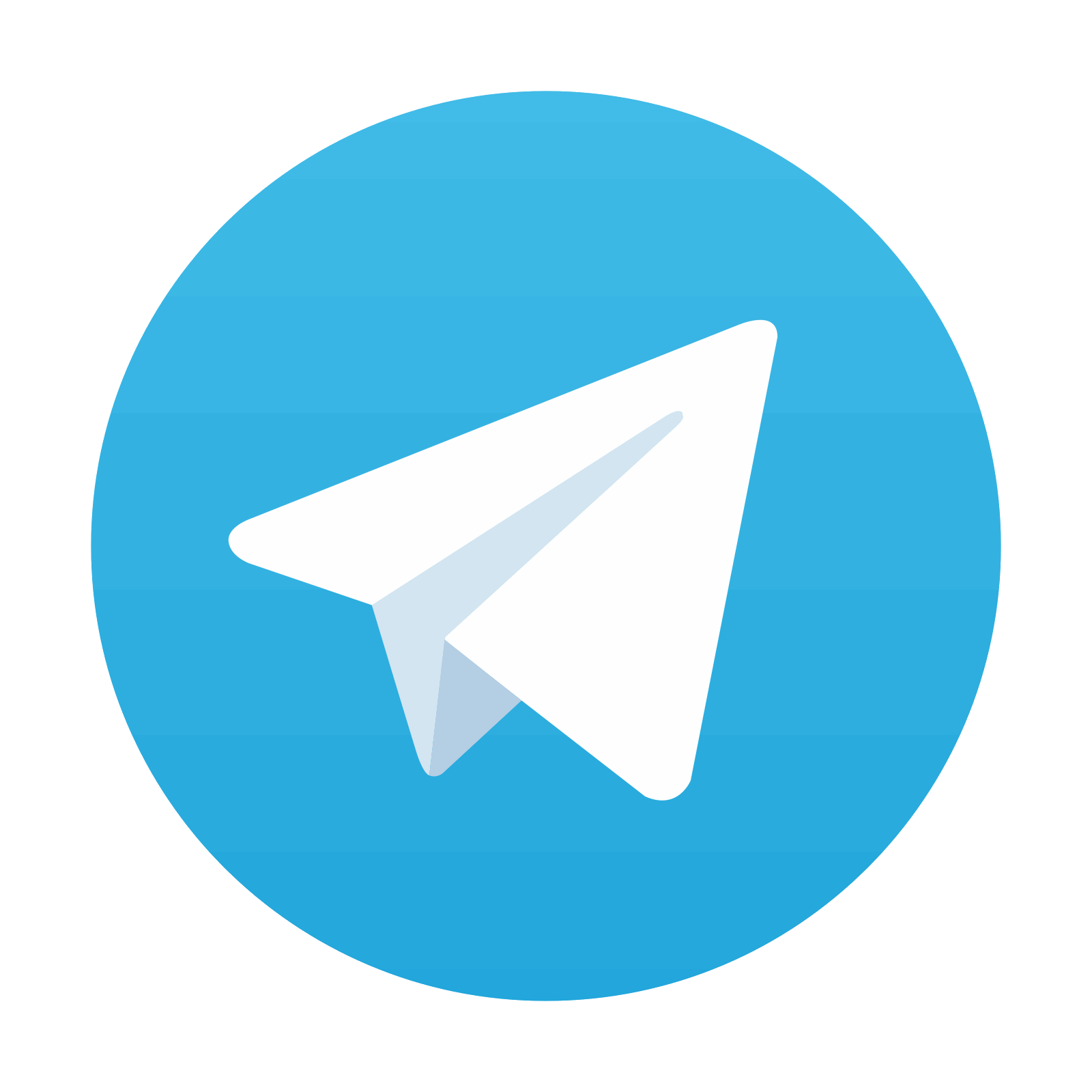
Stay updated, free articles. Join our Telegram channel

Full access? Get Clinical Tree
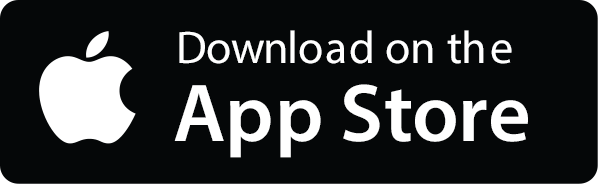
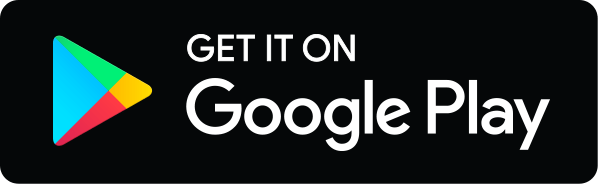
