Introduction
The traditional principle in neuro-oncology is to study the tumor first, with little consideration regarding the host; that is, the brain. Nevertheless, to define the optimal therapeutic management for each patient bearing a diffuse glioma (DG), the concept of oncofunctional balance must be taken into account. Although understanding of the natural history of the disease is crucial, this is not enough. The adaptive reaction of the central nervous system (CNS) induced by the glioma growth and spread should also be investigated. Dynamic interactions between the glioma and the CNS may allow neuroplasticity phenomena, resulting in the compensation of glial tumor progression and in the preservation of quality of life until the limits of plastic potential are reached, thus leading to seizures and/or neurologic deficits.
This chapter analyzes mechanisms underpinning brain plasticity, based on original insights from cerebral mapping and functional outcomes in patients who have had awake surgery for DG. The aim is to switch from a localizationist model to a hodopical framework of neural processing. Such a connectomal account of brain organization results in tailoring an adapted therapeutic strategy according to the dynamic relationships between DG course and adaptational cerebral functional remapping at the individual level.
Neural plasticity
Historical Note
For more than 1 century, 2 different concepts of CNS functioning were suggested. First, the theory of equipotentiality hypothesized that the whole brain, or at least 1 complete hemisphere, was involved in the practice of a functional task. By contrast, in the theory of localizationism (based on phrenology), each part of the brain was supposed to correspond with a specific function. Progressively, an intermediate view emerged, namely a brain organized (1) in highly specialized functional areas, called eloquent regions (eg, the rolandic, Broca, and Wernicke areas), for which any lesion generates major permanent neurologic deficits; and (2) in noneloquent regions, with no functional consequences when damaged. Therefore, the dogma of a static CNS organization, with the inability to compensate for any damage involving the eloquent regions, was settled for a long time. Nevertheless, thanks to regular observations of functional improvement after injuries of structures considered as critical, this principle of a rigid CNS was called into question. If there is a lesion of neural tissue, the brain can reallocate the remaining physiologic resources to maintain a satisfactory level of function in a cognitively and socially demanding environment. Thus, many investigations were performed, initially in vitro and in animals, then more recently in humans, in order to study the mechanisms underlying these compensatory phenomena: the concept of neuroplasticity was developed. Advances in functional mapping and neuroimaging techniques have dramatically changed the classic modular model for a new dynamic and distributed perspective of CNS organization, able to reorganize itself both during everyday life (learning) and after a pathologic event such as a DG. However, although there are a few literature reports on cases of functional recovery or adaptation in various neurologic contexts, the most persuasive body of evidence for the brain’s lesion-induced plasticity comes from neurosurgery in general and from the resection of DG in particular.
Definition and Mechanisms
Neuroplasticity is a continuous processing allowing short-term, medium-term, and long-term remodeling of the neuronosynaptic organization, with the aim of optimizing the functioning of neural networks during phylogenesis, ontogeny, and physiologic learning, and following brain injury. At a microscopic scale, pathophysiologic mechanisms underlying plasticity are mainly represented by synaptic efficacy modulations, unmasking of latent connections, phenotypic modifications, synchrony changes, and neurogenesis. At a macroscopic scale, diaschisis, functional redundancies, cross-modal plasticity with sensory substitution, and morphologic changes are involved. The behavioral consequences of these phenomena have been investigated, in particular the ability to recover after cerebral damage (postlesional plasticity), and the underlying patterns of functional remapping have been analyzed. Neural plasticity can be conceived only in a dynamic account of CNS organization; the brain is an ensemble of complex networks that form, reshape, and flush information dynamically. Thus, reorganization could occur, based on the existence of multiple and overlapping redundancies organized hierarchically. These findings have shown that neuronal aggregates, beside or outlying a lesion, can increasingly adopt the function of the damaged area and switch their own activation pattern to substitute the lesioned structure while facilitating functional recovery.
In this context, the concept of the brain connectome has recently emerged. This concept captures the characteristics of spatially distributed dynamic neural processes at multiple spatial and temporal scales. The new science of brain connectomics is contributing to both theoretic and computational models of the brain as a complex system, and experimentally to new indices and metrics (eg, nodes, hubs, efficiency, modularity) in order to characterize and scale the functional organization of the healthy and diseased CNS. However, in pathology, neural plasticity is possible only if the subcortical connectivity is preserved, to allow spatial communication and temporal synchronization among large interconnected networks, according to the principle of hodotopy. Although distinct patterns of subcortical plasticity were identified, namely unmasking of perilesional latent networks, recruitment of accessory pathways, introduction of additional relays within neuron-synaptic circuits, and involvement of parallel long-distance association pathways, the real capacity to build a new structural connectivity (so-called rewiring) leading to functional recovery have not yet been shown in humans.
The time course of diseases and central nervous system reshaping
In contrast with acute lesions such as stroke or traumatic cerebral injury, DG is a progressively growing tumor that invades the CNS over weeks/months (high-grade gliomas) or even over years (low-grade gliomas). For example, this slow time course explains why patients with low-grade gliomas usually have no or only mild functional deficits, despite the frequent involvement of eloquent structures, because these lesions induce progressive functional brain reshaping. In high-grade gliomas, because of their more rapid growth, neurologic deficits are more frequent at the time of diagnosis. However, because of facilitated access to neuroimaging, many patients with anaplastic glioma or glioblastoma now show only slight or moderate neurologic deficits at the time of diagnosis, supporting some degree of neural reorganization. Therefore, neuroplasticity cannot be fully understood without considering the temporal pattern of the cerebral injury. In stroke, even if many patients improve within the months following damage, only around 25% of patients totally recover, whereas more than 90% of patients with diffuse low-grade glioma (DLGG) (same location as stroke) have a normal neurologic examination.
Using a neurocomputational model based on the training of a series of parallel distributed processing neural network models, a recent study simulated acute versus slow-growing injuries. A very different pattern emerged in the simulation of DG compared with the simulation of stroke, with slow decay of the links within the same subnetwork leading to minimal performance decline, in agreement with the patient literature. Moreover, at the end of the decay regimen, the entire affected hidden layer could be removed on the simulation with no effect on performance, which closely matches the lack of major impairment from DG resection. This finding is likely caused by abrupt stroke causing rapid neuronal death, whereas DG initially spares neuronal tissue and thus gives time for cerebral remapping. Thus, the functional status at the time of diagnosis might be a good reflection of the natural history of the disease.
Preoperative functional reallocation in patients with diffuse glioma
Concerning the neural foundations of functional compensation in DG before any treatment, the patterns of reorganization may differ between patients. Preoperative functional neuroimaging has shown that 4 kinds of preoperative functional redistribution are possible in patients without any deficit : (1) function still persists within DG, thus there is a very limited chance to perform a fair resection; (2) eloquent areas are redistributed around the tumor, thus there is a reasonable chance to perform at least a near-total resection despite a likely immediate transient deficit and subsequent recovery; (3) a preoperative compensation by remote areas within the lesional hemisphere has occurred; (4) areas are recruited in the contralateral hemisphere and, in the 2 last patterns, the chances to achieve a total resection are very high, with only a slight and transient worsening. These different patterns can be associated. Therefore, in DG involving eloquent areas, plasticity mechanisms seem to be based on a hierarchically organized model; that is, first there is intrinsic reorganization within injured areas (index of favorable outcome); second, when this reshaping is not sufficient, other regions implicated in the functional network are recruited, in the ipsilateral hemisphere (close or even remote to the tumoral site) and then in the contralateral hemisphere if necessary.
To sum up, as recently supported by magnetoencephalography study, a focal DG disturbs the functional and effective connectivity within the whole brain, and not only in restricted areas around the tumor. These network dysfunctions are related to cognitive processing in patients with DG. When objective neuropsychological and health-related quality-of-life assessments have been performed, visuospatial, memory, attention, planning, learning, emotional, motivational, and behavioral deficits have regularly been observed in patients with glioma. These results show that brain plastic potential has limitations, which should be studied at the individual level. Because surgical treatment itself may induce changes in large-scale functional connectivity, such knowledge of individual patterns of remapping should be taken into account in order to tailor personalized therapeutic management in patients with DG.
Intraoperative plasticity in diffuse glioma surgery
Intrasurgical Electrostimulation Mapping
During surgery for DG, especially in eloquent areas, it has become common clinical practice to awaken patients in order to assess the functional role of restricted cerebral regions. Surgeons can maximize the extent of resection, and thereby improve the overall survival, without generating functional impairments, thanks to the individual mapping and preservation of critical structures. Therefore, resection is not performed according to purely anatomic and oncological limits, but up to functional boundaries. Patients perform several sensorimotor, language, cognitive, and emotional tasks while the surgeon temporarily interacts with discrete areas within the gray and white matter around the tumor, using direct electrostimulation mapping (DEM). If the patients stops to perform the task or produces an incorrect response, the surgeon avoids removing the stimulated site. DEM transiently interacts locally with a small cortical or axonal site but also nonlocally, because focal perturbation disrupts the whole subnetwork sustaining a given function. Thus, DEM represents a unique opportunity to identify with great accuracy and reproducibility, in vivo in humans, the structures that are crucial for brain functions both at cortical and subcortical (white matter and deep gray nuclei) levels ( Figs. 18.1 and 18.2 ).


Task Selection for Intrasurgical Cognitive Mapping
Optimal selection of the tasks used during intraoperative mapping is essential to preserve a normal life. For example, language mapping can be used to identify possible crucial epicenters in the right, nondominant, hemisphere in left-handers or ambidextrous patients (and even in some right-handers) if language disturbances are detected on the preoperative cognitive assessment, even in cases of left-lateralization on functional MRI. The aim is to map the neural circuits underpinning the different but interactive subfunctions, which should be preserved intraoperatively, by serving as boundaries of resection. DEM allows the mapping of many functions, such as movement (including control of bimanual coordination) ; somatosensory function ; visual function ; auditory-vestibular function ; spatial awareness ; language, including spontaneous speech and counting, object naming, verbal comprehension, writing, reading, syntax, bilingualism, switching from one language to another (see Ref. for a recent model of anatomofunctional connectivity of language based on DEM); higher-order functions such as calculation, memory, attention, cognitive control, cross-modal judgement, nonverbal comprehension ; mentalizing; and consciousness.
Acute Functional Remapping During Diffuse Glioma Surgery
First, intraoperative stimulation mapping before resection can confirm the functional reshaping induced by DG, as supposed using preoperative functional neuroimaging. Nonetheless, recent studies comparing preoperative functional MRI with intraoperative DEM have shown a low reliability of neuroimaging, in particular for high-grade gliomas, with a sensitivity of only 37.1% and a specificity of only 83.4% for language mapping, showing that this technique is not reliable enough for it to be used in clinical practice.
Regarding intrasurgical plasticity, a remarkable observation concerns the existence of acute functional remapping triggered by the resection itself and taking place within 30 to 60 minutes of beginning the surgery. This type of acute reorganization has been well documented in the sensorimotor system. In several patients harboring a frontal lesion, although stimulation of the precentral gyrus induced motor responses only at the level of a limited number of cortical sites before resection, an acute unmasking of redundant motor sites located within the same precentral gyrus, and eliciting the same movements as the previous adjacent sites when stimulated, was observed immediately following lesion removal. Acute unmasking of redundant somatosensory sites was also regularly observed within the retrocentral gyrus in patients operated on for a parietal glioma. Furthermore, it was equally possible to detect a redistribution within a larger network involving the whole rolandic region; that is, with unmasking of functional homologues located in the precentral gyrus for the first cortical representation and in the retrocentral gyrus for its redundancy (or vice versa). The most likely hypothesis suggests that a local increase of cortical excitability allows an acute unmasking of latent functional redundancies (ie, multiple cortical representations of the same function), via a decrease of intracortical inhibition. In agreement with this idea, animal models have shown that focal brain damages induce large zones of enhanced cortical excitability in both the lesioned and the intact hemispheres. Likewise, human studies have provided evidence that the level of intracortical inhibition is reduced in the damaged hemisphere in patients with stroke. Therefore, it is tempting to speculate that the latent redundant networks revealed by the resection process participate in functional recovery. This idea fits well with the importance of adjacent reorganizations for behavioral recuperation.
White matter tracts as the limit of neural plasticity: the concept of hodotopy
Subcortical Connectivity and the Minimal Common Brain
Although the plastic potential is high at the cortical level, subcortical plasticity is low, implying that axonal connectivity should be surgically preserved to allow postoperative compensation. By combining cortical function and axonal connectivity, an updated model of CNS processing was proposed, moving from the localizationist model to a hodotopical framework. In pathology, a topological mechanism (from the Greek topos, meaning place) refers to a dysfunction of the cortex (deficit, hyperfunction, or a combination of both), whereas a hodological mechanism (hodos, meaning road or path) refers to dysfunction related to connecting pathways (disconnection, hyperconnection, or a combination of both). Thus, clinicians should take into account the complex functioning of a large-scale distributed corticosubcortical network to understand its physiology as well as the functional consequences of a lesion in this circuit, with possible different deficits depending on the location and the extent of the damage (eg, purely cortical, or purely subcortical, or both).
Recently, probabilistic atlases of postsurgical residue and of functional plasticity were computed in patients who underwent resection for a DG based on intraoperative DEM. Combining the intrasurgical functional data with postoperative anatomic imaging provided both a greater understanding of the functional limits of surgical removal, and new insights into neuroplasticity. These atlases provided a general framework to establish anatomofunctional correlations by computing for each brain voxel its probability to be left, because of its functional role, on the postoperative MRI. Their overlap with the cortical MNI (Montreal Neurological Institute) template and a diffusion tensor imaging tractography atlas offered a unique tool to analyze the potentialities and the limitations of interindividual variability and plasticity, both for cortical areas and axonal pathways. These atlases highlighted the crucial role of the axonal pathways in postlesional reorganization. A low probability of residual tumors on the cortical surface was observed, whereas most of the regions with high probability of residual tumor were located in the white matter. Thus, the functions subserved by long-range projection and association fibers seem to be less subject to interindividual variability and reorganization than cortical sites (except in primary unimodal areas in a small set of neural hubs). Because these pathways define the surgical deep limits, and because DGs infiltrate these tracts, subcortical connectivity constitutes the main obstacle to radical resection.
For some of these structures, their low potential of remodeling could be explained because they act as input or output areas: input sites convey, or are the first relay of, information entering the brain, whereas output sites are the last relay or the fiber tracts sending information outside the brain. These areas include the primary motor and somatosensory areas, the corticospinal and thalamocortical tracts, and the optic radiations (ie, the projection fibers). These areas are mainly unimodal and organized serially. The absence of a parallel alternative pathway explains the impossibility of restoring their function after any damage. For all other areas, their nonresectability should be analyzed within a network perspective. High-order cognitive processes are mediated by short-range and long-range networks, with cortical epicenters connected by U-shaped fibers, associative and commissural pathways, and a particular network topology (like the small word one) is required to allow proper synchronization between several distant areas. As mentioned, a local lesion can disturb a whole network, which in turn could ultimately hamper the function sustained by this network. For example, beyond the posterior part of the left superior temporal gyrus, subcortical structures like the inferior fronto-occipital fascicle (IFOF) and arcuate fascicle (AF) are nonresectable because their lesions would cause such major changes in the network that the dynamic plastic potential would be overwhelmed. Note that areas such as the left posterosuperior temporal gyrus are considered as hubs in revisited models of cognition. These functional epicenters allow a plurimodal integration of multiple data coming from the unimodal areas. This integration may lead to conceptualization, performed at the level of a wide network that includes the hubs. These hubs are interconnected by subcortical pathways, themselves crucial for brain function; for example, the AF, which enables a direct communication between the posterior temporal and frontal plurimodal regions. The reproducibility of these results, despite the interindividual anatomofunctional variability and plastic mechanisms, may suggest the existence of a minimal common brain, necessary for the basic cognitive functions, even if it is not likely to be sufficient for more complex functions such as multiprocessing. This hypothesis is in good agreement with recent biomathematical models analyzing the effect of a simulated focal lesion on the whole-brain network topology. For these areas, even biological plasticity would fail in the long term to repair the connectivity required to rebuild an effective network topology, hence a functional circuit.
In summary, these atlases shed new light on the topological organization of CNS, may be useful in predicting the likelihood of recovery (as a function of lesion topology), and thus give an objective preoperative estimation of the expected extent of resection for DG resected under intraoperative DEM. This rationale assists neurosurgeons in decisions regarding surgical resection and may contribute to the elaboration of a therapeutic consensus for DG.
Anatomofunctional Subcortical Connectivity Subserving Neural Functions
Neurosurgeons should therefore improve their knowledge of white matter circuitry. As mentioned earlier, because DG migrates along the main projection, commissural, and long-distance association bundles, the optimal therapeutic approach cannot be defined without understanding the organization of individual neural networks. Cognitive neurosciences are closely related to neuro-oncology, because the use of DEM also provides new insights into the circuits mediating cognitive and behavioral functions.
For example, beyond the corticospinal (pyramidal) and thalamocortical (somatosensory) tracts, the functional connectivity underpinning movement planning and execution also includes the negative motor network, partly subserved by the frontostriatal tract. The visuospatial network is subserved not only by the optic radiations but also by the inferior longitudinal fascicle (ILF), which is critical for visual recognition, and part II of the superior longitudinal fascicle (SLF), which is crucial for spatial cognition, especially in the right hemisphere. Language is mediated by dorsal and ventral pathways. Schematically, the medial part of dorsal stream (AF) subserves phonological and repetition processing, and the lateral part of the SLF subserves speech articulation, and also represents the limiting factor of plastic potential of the left ventral premotor cortex. The ventral stream, mainly underlain by the IFOF (direct ventral route), subserves language semantics, whereas the ILF (indirect ventral route) is involved in lexical retrieval and reading. Concerning the network sustaining mentalizing (theory of mind), which is crucial for emotion and social cognition, intraoperative DEM combined with preoperative and postoperative behavioral examinations showed that this function is made possible by parallel functioning of 2 subsystems: accuracy of identification (mirror system; ie, the ability to appreciate other people’s emotions) and attribution of mental states (high-level inferential mentalizing) are subserved by the AF/SLF complex and the cingulum, respectively.
To sum up, the vision of the neural basis of cognition begins to shift. For a long time, cognitive functions were conceived in associationist terms of centers and pathways, the general assumption being that information is processed in localized cortical regions with the serial passage of information between areas through white matter tracts. In the hodotopical account, brain functions are conceived as resulting from parallel delocalized processing performed by distributed groups of connected neurons rather that individual centers. In contrast with serial models in which 1 process must be finished before the information accedes to another level of processing, these new models of independent networks state that different processing can be performed simultaneously with interactive feedbacks. In this connectomal view, neurologic function comes from the synchronization between different epicenters, working in phase during a given task, and explaining why the same hub may take part in several functions depending on the other cortical areas with which it is temporarily connected at any time. Brain processing therefore should not be conceived as the sum of several subfunctions. Rather, cerebral function results from the integration and potentiation of parallel (although partially overlapped) subnetworks.
To illustrate this multimodal model of brain networking, DEM showed the existence of an amodal executive system (including prefrontal cortex, anterior cingulum, and caudate nucleus) involved in the cognitive control of more dedicated subcircuits; for instance, the subnetwork underlying language switching in multilingual people (itself constituted by a wide corticosubcortical network comprising posterotemporal areas, supramarginal and angular gyri, inferior frontal gyrus, and SLF). Similar reasoning can be applied to the multimodal (verbal and nonverbal) working memory and attentional functions, which seem to be supported by distinct subparts of the SLF, according to the data provided by axonal DEM combined with perioperative neurocognitive assessments. Simultaneous recruitment of these subnetworks in addition to the distinct circuits specifically involved in language and visuospatial cognition are necessary if a person performs a sustained double task (eg, combination speech and line bisection test every 4 seconds throughout the resection) as regularly asked of awake patients during electrostimulation to increase the reliability of functional mapping, each axonal DEM being able to disrupt a specific subfunction with no consequences for the others. The next step is to explore interaction between corticosubcortical circuits involved in different types of consciousness, such as noetic consciousness (the awareness of knowing and understanding the world and the self, while being aware of such an awareness) and consciousness of the external environment.
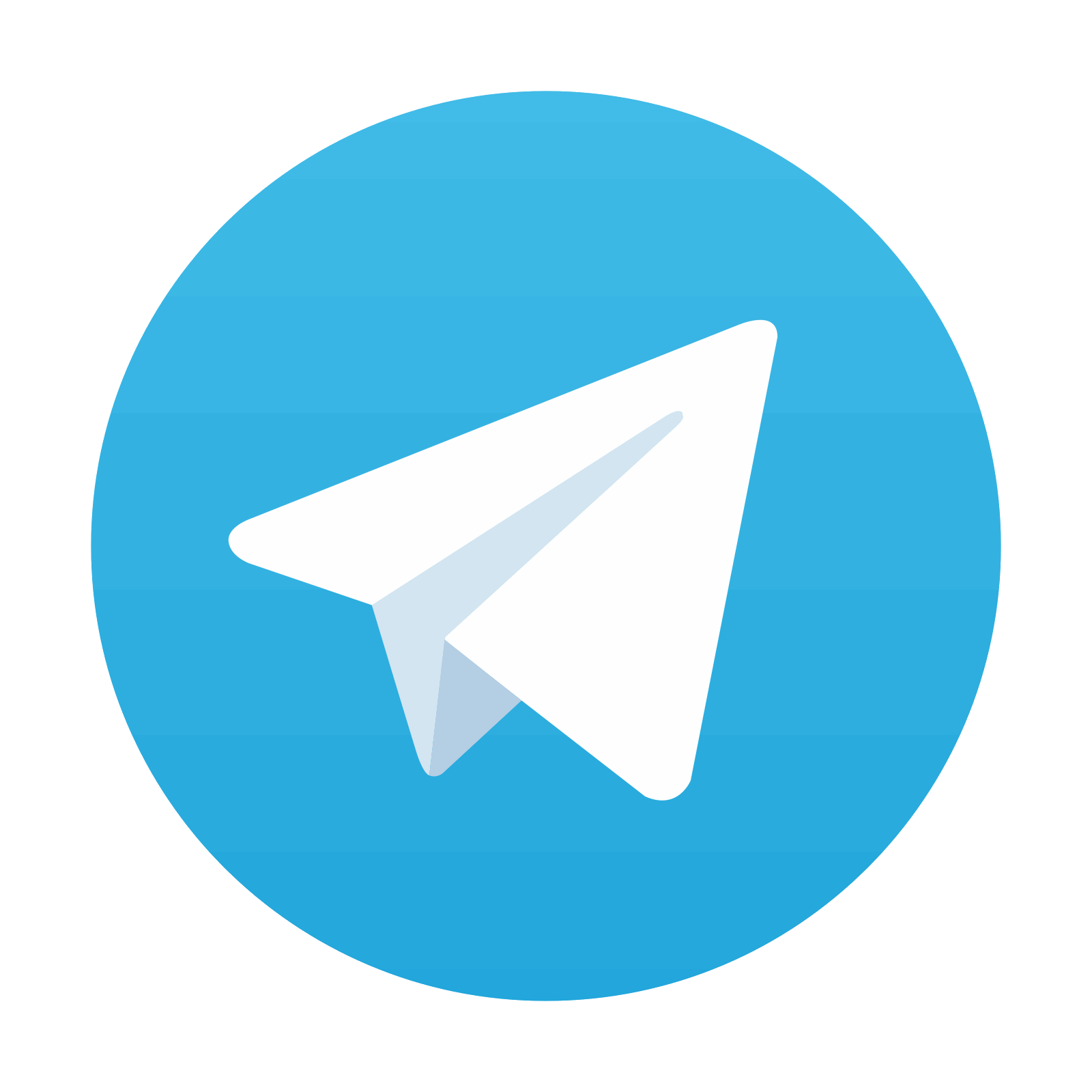
Stay updated, free articles. Join our Telegram channel

Full access? Get Clinical Tree
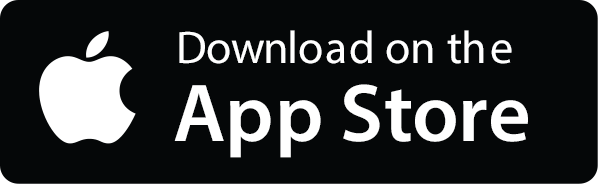
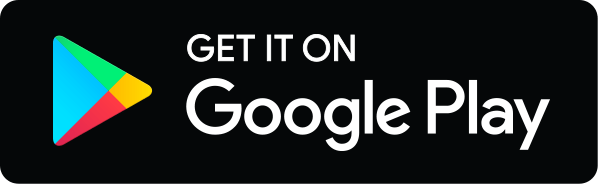