Fig. 7.1
Left panel: Average number of p-erk immunoreactive neurons in nucleus accumbens (NAc, Bregma: 1.92) in response to a challenge injection of amphetamine (0.5 mg/kg, i.p.), 21 days after a pretreatment with candesartan (Cv, 3 mg/kg, 5 days, p.o.) or vehicle (Veh) and a treatment with amphetamine (amph, 5 mg/kg, 1 day, i.p.) or saline (Veh-sal, Cv-sal, Veh-amph, and Cv-amph). Values are means ± SEM. *p < 0.05 significantly different from the other amphetamine-challenged groups, +p < 0.05 significantly different from Veh-amph, (1-way ANOVA, post hoc Newman-Keuls). Right panel: Photomicrographs ×200 magnifications showing the pattern of p-erk immunoreactive neurons
Recent results from our laboratory have showed an increase in the AT1 receptors protein expression in CPu and NAc, 7 and 21 days after the amphetamine treatment (5 mg/kg, i.p., 1 day), and a decrease in AOGEN RNAm and protein expression in CPu, 21 days after the amphetamine treatment, indicating that amphetamine induced long-lasting changes in brain RAS system [68]. Moreover, in another experiment the functional role of AT1 receptors in the expression of sensitization to amphetamine was studied. The AT1 receptors were blocked (Losartan 8 μg/μL by brain side) in CPu and NAc, 5 min before an amphetamine challenge (0.5 mg/kg, i.p) 21 days after amphetamine (5 mg/kg, i.p) administration. These results showed that the expression of amphetamine-induced sensitization was blunted after the blockade of AT1 receptors in CPu [68].
The experiments provide evidence supporting the brain RAS involvement in behavior sensitization induced by amphetamine, contributing to the knowledge of neurobiological mechanisms involved in the psychostimulant drug effects.
The aforementioned evidence points to a new role of the brain RAS in long-lasting effects induced by psychostimulant drugs.
RAS and Ethanol
Alcohol is another of the major drugs abused today, and alcoholism and alcohol-related disorders are disturbingly prevalent in contemporary society. Despite the ever-increasing contributions to the field of alcohol research, a clinically effective pharmacological treatment for alcohol abuse has yet to be developed [69]. It was extensively studied in animals the relationship between alcohol intake and the RAS [70, 71]. In this sense, it was also found that Ang II induced alcohol consumption throught AT1 receptors activation [71]. In 1988, Spinosa and co-workers assessed the ability of the ACE inhibitors (captopril and enalapril) to change alcohol consumption in laboratory rats. The drugs were found to produce a marked reduction in voluntary alcohol consumption independently of changes in blood pressure and without altering alcohol pharmacokinetics [72]. As was observed with psychostimulant drugs, the mesolimbic DA system had been hypothesized to mediate the reinforcing actions of other drugs such as ethanol. Acute administration of ethanol directly alters the DA neurotransmission. Alcohol-preferring animals exhibited a greater dopaminergic response to acute ethanol administration than alcohol−non-preferring animals [73]. Electro-physiological studies demonstrated that ethanol administration increased the firing rate of VTA DA neurons in vivo [74] and in vitro [75, 76]. Systemic administration of ethanol has been shown to increase extracellular DA levels in the NAc and VTA [77–80]. Additionally, microdialysis studies demonstrated an increase in DA release in the NAc following oral self-administration of ethanol [81–83]. Finally, previous data indicate that microinjections of ethanol matabolite into the posterior VTA increase dopamine release in the NAc shell [84].
Involvement of brain RAS components in drug-induced responses has been investigated by correlating altered RAS function and ethanol consumption. Increased expression of AOGEN was found in microarray studies of brains from different rodent lines selectively bred for high ethanol preference (HAP mice) compared with their respective controls [85]. Supporting this idea, chronic ethanol consumption tended to increase AT1 binding in CPu and NAc in C57BL/6 mice, an ethanol-tolerating strain [10].
The studies performed in animals with genetic modification in several components of the RAS demonstrated that Ang II via AT1 receptor action is a positive modulator of spontaneous ethanol consumption in rodents [86, 87]. Transgenic rats expressing a specific AOGEN antisense RNA in the brain [TGR(ASr-AOGEN)680] present angiotensin generation and drastically reduced and modified levels of AT1 receptors in the CNS. These animals show lower ethanol consumption and altered responses after ethanol intoxication compared with controls. Supporting the idea that angiotensin-mediated DA release plays an essential role in Ang II-triggered regulation of alcohol intake, altered DA concentrations were found in relevant brain areas. Indeed, concentrations of DA as well as its principal metabolite (DOPAC) were found to be strongly reduced in a region covering the VTA of TGR(ASrAOGEN)680 rats [88]. It is interesting to note that mice lacking the D2 receptor gene were less sensitive to alcohol-induced ataxia than their wild type littermates while they ingested lower amounts of alcohol in free choice experiments [89]. It is believed that Ang II stimulates DA release in NAc and CPu [9], and angiotensin receptors are expressed in brain areas such as the NAc, where dopaminergic transmission has been strongly implicated in alcohol self-administration and sensitivity [10, 46]. Evidence has shown an increase in the voluntary consumption of alcohol in the transgenic mice expressing a rat angiotensinogen transgene (TGM123). These animals have elevated levels of Ang II resulting from additional expression of the AOGEN transgene. Consumption was significantly reduced by administration of fluphenazine, a DA receptor antagonist. Thus, increased alcohol intake in mice over-expressing angiotensin may relate to an interaction of Ang II with dopaminergic systems. Furthermore, the knockout mice lacking the AOGEN gene (TLM), drank even less alcohol than the controls. Furthermore, it was found that the ACE inhibitor Spirapril, known to cross the blood–brain barrier and consequently lower the Ang II levels in brain and blood circulation, suppressed alcohol intake in the TGM123 mice [87]. Ang II ICV infusion in the ethanol-tolerating mice, C57BL/6J, stimulated the intake of 4 % ethanol solution and caused a transient increase in the intake of 10 % ethanol solution. The increase in ethanol solution intake that occurred did not increase progressively over the 3 days of Ang II treatment, as is usually observed when water is available [90], probably indicating a response to hedonic stimulus of alcohol more than to homeostatic deregulation by Ang II infusion.
The stimulation of brain RAS by food depletion also modifies alcohol consumption. Animals deprived of ad libitum food access had higher ethanol consumption than controls during treatment, and the behavior was reversed when free access to rat food was restored [91].
Controversial results have been reported on ICV infusion of Ang II in rats. An increased alcohol intake has been described by Fitts [92], but most experiments indicate no functional effects for this type of Ang II administration [90, 91, 93]. It is important to highlight that these experiments are based on invasive techniques with unpredictable effects on drinking behavior. Functional stimulation of Ang II receptors in the vicinity of the lateral ventricle does not exert an influence on alcohol consumption. Furthermore, as alcohol consumption does not decrease at the expense of a robust increase in water consumption, it is possible to assume dissociation between the effects of Ang II on alcohol and water intake [93].
Experiments have been conducted with pharmacologic modification of RAS activity. The blockade of the receptors with different doses of the Ang II antagonist SarThr-Ang II does not modify alcohol consumption. Interestingly, ACE inhibitors reduce alcohol intake in a dose-dependent manner in genetically selected alcohol-preferring and –non-preferring rats, as well as in Wistar rats [94–96]. This may indicate that ACE inhibitors are not acting through peripherally based Ang II-related processes to reduce alcohol consumption. Because peripheral administration of ACE inhibitors can elevate central RAS activity, the present findings indicate that if the reduction in alcohol intake produced by ACE inhibition is mediated through the RAS, the locus of this effect is likely to be at a central site not accessible to a peripherally administered Ang II antagonist [95].
These results clearly support the hypothesis that the central RAS, through AT1 receptors, are involved in the control of alcohol intake behaviour, modulating the DA system.
Conclusion
It is widely known that the high incidence in health costs and in patient welfare and its environmental deterioration are a result of drug abuse and alcohol-related diseases worldwide. In this context it is very important to study new targets in order to provide new pharmacological tools for the treatment of these pathologies. A group of drugs that interfere with the RAS, widely used in clinical practice for hypertension treatment and cardio protection, have particular advantages because they are nearly free of severe side effects. Therefore, the results presented in this chapter regarding the key role of the brain RAS in the neuroadaptative response to drugs of choice for abuse show a new therapeutic application for AT1 blockers. More research is needed to reveal the effectivity of long-term treatment of available ACE inhibitors or AT1 blockers that could be used in the treatment of drug abuse disorders.
References
1.
Allen AM, Zhuo J, Mendelsohn FA. Localization and function of angiotensin AT1 receptors. Am J Hypertens. 2000;13(1 Pt 2):31S–8.PubMed
2.
Lenkei Z, Palkovits M, Corvol P, Llorens-Cortes C. Expression of angiotensin type-1 (AT1) and type-2 (AT2) receptor mRNAs in the adult rat brain: a functional neuroanatomical review. Front Neuroendocrinol. 1997;18(4):383–439.PubMed
3.
Bader M, Ganten D. It’s renin in the brain: transgenic animals elucidate the brain renin angiotensin system. Circ Res. 2002;90(1):8–10.PubMed
5.
Phillips MI. Functions of angiotensin in the central nervous system. Annu Rev Physiol. 1987;49:413–35.PubMed
7.
Ferguson AV, Bains JS. Actions of angiotensin in the subfornical organ and area postrema: implications for long term control of autonomic output. Clin Exp Pharmacol Physiol. 1997;24(1):96–101.PubMed
8.
Ferguson AV, Washburn DL, Latchford KJ. Hormonal and neurotransmitter roles for angiotensin in the regulation of central autonomic function. Exp Biol Med (Maywood). 2001;226(2):85–96.
9.
Brown DC, Steward LJ, Ge J, Barnes NM. Ability of angiotensin II to modulate striatal dopamine release via the AT1 receptor in vitro and in vivo. Br J Pharmacol. 1996;118(2):414–20.PubMedCentralPubMed
10.
Daubert DL, Meadows GG, Wang JH, Sanchez PJ, Speth RC. Changes in angiotensin II receptors in dopamine-rich regions of the mouse brain with age and ethanol consumption. Brain Res. 1999;816(1):8–16.PubMed
11.
Wright JW, Harding JW. Brain angiotensin receptor subtypes in the control of physiological and behavioral responses. Neurosci Biobehav Rev. 1994;18(1):21–53.PubMed
12.
Stornetta RL, Hawelu-Johnson CL, Guyenet PG, Lynch KR. Astrocytes synthesize angiotensinogen in brain. Science. 1988;242(4884):1444–6.PubMed
13.
Ganong WF. Origin of the angiotensin II secreted by cells. Proc Soc Exp Biol Med. 1994;205(3):213–9.PubMed
14.
Chai SY, Mendelsohn FA, Paxinos G. Angiotensin converting enzyme in rat brain visualized by quantitative in vitro autoradiography. Neuroscience. 1987;20(2):615–27.PubMed
15.
Johnston CI. Biochemistry and pharmacology of the renin-angiotensin system. Drugs. 1990;39 Suppl 1:21–31.PubMed
16.
Lippoldt A, Paul M, Fuxe K, Ganten D. The brain renin-angiotensin system: molecular mechanisms of cell to cell interactions. Clin Exp Hypertens. 1995;17(1–2):251–66.PubMed
17.
Saavedra JM. Brain angiotensin II: new developments, unanswered questions and therapeutic opportunities. Cell Mol Neurobiol. 2005;25(3–4):485–512.PubMed
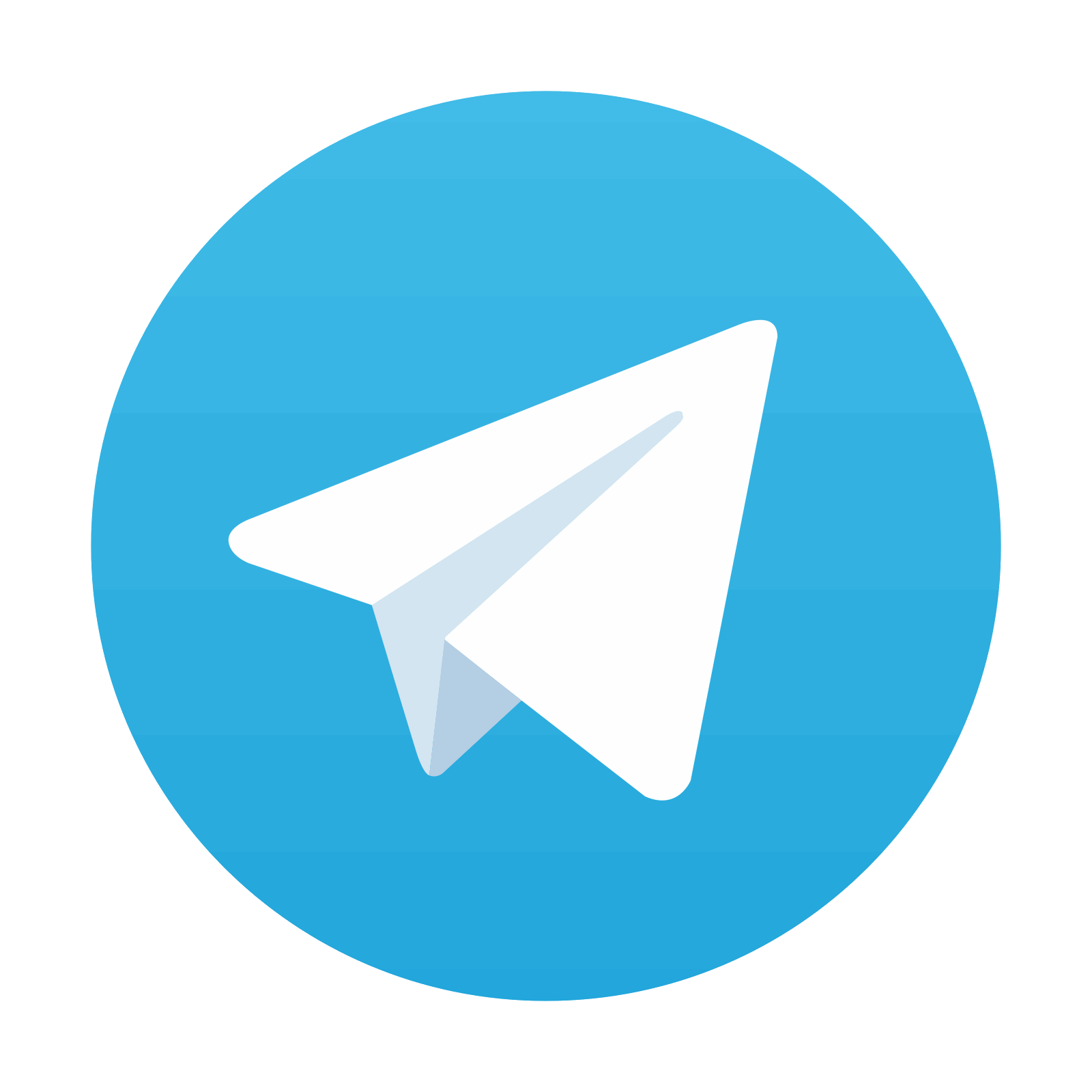
Stay updated, free articles. Join our Telegram channel

Full access? Get Clinical Tree
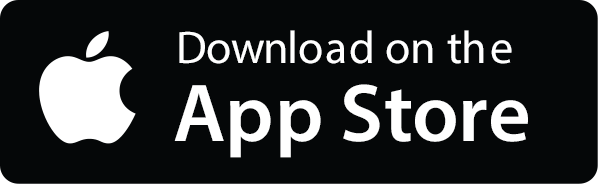
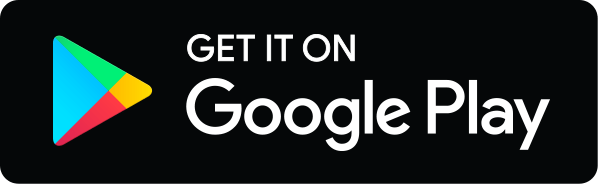