Fig. 26.1
Brain perfusion SPECT in a normal dog (horizontal/transverse slices). The colour scale gives information on the intensity of radioactivity registered in different areas: highest uptake is related with the colours at the top of the scale, low uptake with those at the bottom. The areas with highest radioactivity represent neuronal tracer uptake in the cortical and subcortical areas
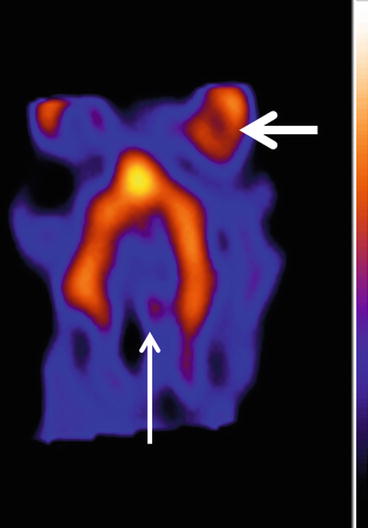
Fig. 26.2
A typical image of the distribution of the 5-HT2A receptor radioligand in the cortical areas (horizontal/transverse slices). Note the lack of activity in the cerebellar area, a region void of 5-HT2A receptors and used as a reference region for semiquantification (arrow). Note also the high physiological periorbital uptake (thick arrow)
Individual perfusion data were automatically fit to a template, and a predefined Volume of Interest (VOI) map was used for semiquantification (Brain Registration and Automated SPECT Semiquantification, Hermes, NUD) (Fig. 26.3). Due to the inferior anatomical information on the radioligand images, individual data were manually matched with the corresponding perfusion data with multimodality software (Hermes, NUD). Regions of interest were defined on the perfusion data and automatically transferred to the radioligand data (Fig. 26.4). The cerebellum was used as reference area in the semiquantification procedure. The obtained binding indices provide an estimation of the receptor density in the different cerebral areas.
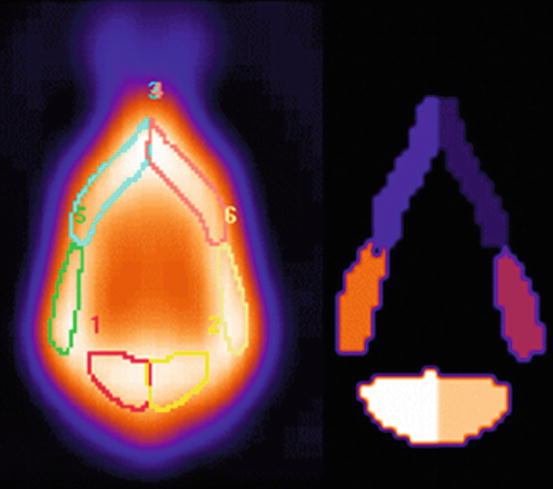
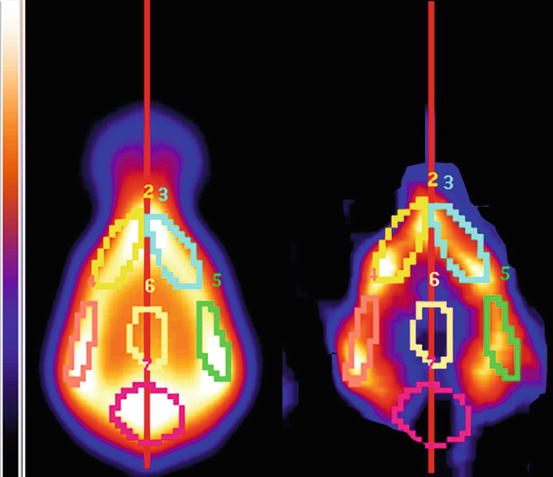
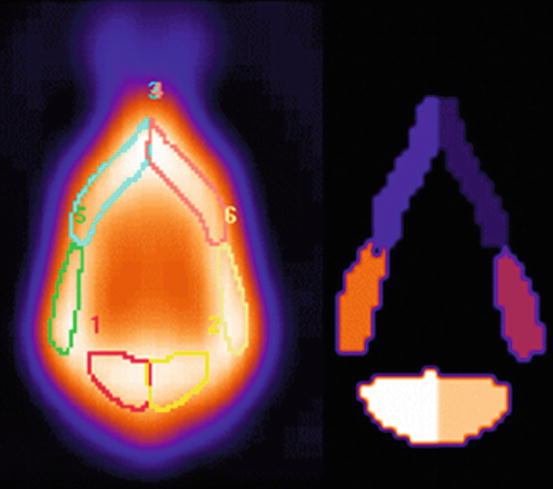
Fig. 26.3
Semiquantification of perfusion data is performed with BRASS software (Hermes, NUD) (normalisation to total brain counts). A template is created from the data of the group of normal dogs (left panel). A manually drawn Volume of Interest (VOI) map is created on this template (right panel) delineating the different cortical and subcortical regions. This template generates the average (with standard deviation) activity in the delineated areas in normal animals. New patient data can be introduced in this system and will be automatically compared with average activity in normal dogs. The regional activity in the patients’ brain and differences with the template will be displayed. 1 Left occipital cortex, 2 Right occipital cortex, 3 Left frontal cortex, 4 Right frontal cortex, 5 Left temporal cortex, 6 Right temporal cortex
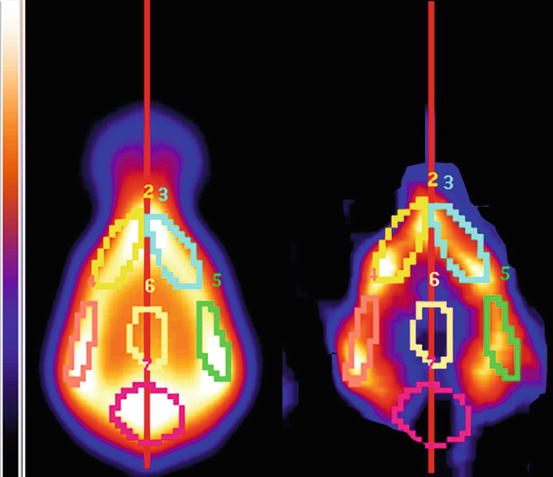
Fig. 26.4
The perfusion images of the same individual are used to define the VOIs on the serotonin-2A receptor radioligand data. Radioligand data are fused with the perfusion data and the VOIs defined on the perfusion data are automatically applied to the radioligand images. The cerebellar area is used as the reference region in the semiquantification procedure. 2 Left frontal cortex, 3 Right frontal cortex, 4 Left temporal cortex, 5 Right frontal cortex, 6 Subcortical area; 7 Cerebellum
Although age difference was statistically not significant, age was taken into account as confounding factor as a previous study showed that, similar to man, age has profound effects on both perfusion and 5-HT2A radioligand binding (Baeken et al. 1998; Meltzer et al. 1998; Peremans et al. 2002a; Rosier et al. 1996; Van Laere et al. 2001) (Fig. 26.5). In impulsive-aggressive dogs a significantly increased binding of the 5-HT2A radioligand was observed in the frontotemporal cortex. No significant perfusion alterations occurred in the different brain regions. There was also no correlation between perfusion and radioligand binding excluding perfusion effects on receptor radioligand binding (Peremans et al. 2003). The statistically higher 5-HT2A binding index in the cortical regions confirms the involvement of the serotonergic system in impulsive-aggressive behaviour. In so far as methodological differences do not preclude the following comparisons, these results are in line with the higher levels of 5-HT2A receptor binding measured with PET in impulsive-aggressive human patients (Rosell et al. 2010; Soloff et al. 2007) and with post-mortem findings in the brain of suicide victims (Arango et al. 1990; Arora and Meltzer 1989; Stanley et al. 1983).
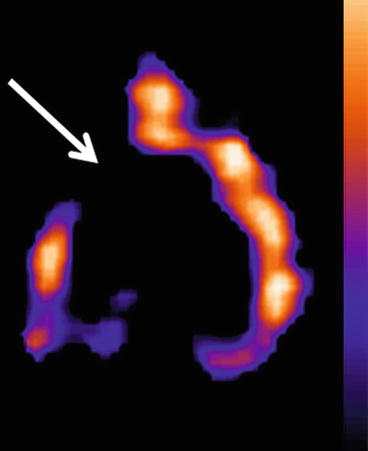
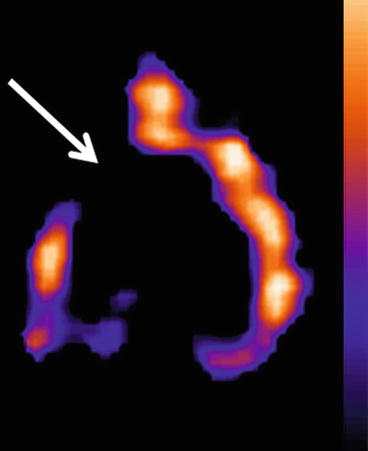
Fig. 26.5
A 5-HT2A receptor SPECT in an aging dog (10 years). Note the decreased (arrow) radioactivity in the frontal cortex compared to the distribution of radioactivity in Fig. 26.2
The increased 5-HT2A receptor binding index could be the consequence of a reduced presynaptic availability of serotonin resulting in reduced synaptic serotonin concentration and therefore leaving more postsynaptic receptors available for radioligand binding or a compensatory up-regulation of the number of postsynaptic 5-HT2A receptors. However, no definite hypothesis can be put forward. First, no microdialysis was performed and therefore no information is present on the concentration of serotonin in the synaptic cleft, and second, it is well known that different neurotransmitter systems interact in general and the noradrenergic and serotonergic system in particular. As an example, up-regulation of 5-HT2A receptors without any modification of 5-HT or 5-HIAA concentrations in the brain can be induced in rats after administration of dexamethasone or exposure to stress (forced swimming) (Katagiri et al. 2001; Takao et al. 1995). The evidence for possible interactions of the noradrenergic and serotonergic systems in canine impulsive aggression was further provided by a post-mortem study measuring β-adrenergic receptor and serotonergic receptor concentrations. A decrease was detected in the β-adrenergic receptors next to an increase in the high-affinity serotonergic receptors in the frontal cortex, hypothalamus and thalamus of aggressive dogs (Badino et al. 2004). In rodents, an interactive role has also been attributed to the dopaminergic system in different research paradigms on impulsivity, albeit the results of these studies are not convergent and further research on the interplay of the serotonergic and dopaminergic system in impulsive behaviour remains mandatory (Dalley and Roiser 2012).
26.2.2 5-HT2A-Receptor Imaging as a Biomarker in Canine Behavioural Research
As previously mentioned in this chapter, aggressive behaviour is considered normal as long as it fits within the canine ethogram. However, distinguishing different types of aggression remains susceptible to subjective behavioural analysis. The challenge also remains to differentiate impulsive aggression from aggression originating from an anxiety disorder. In that respect, functional brain imaging was carried out to evaluate the 5-HT2A receptor as an objective biomarker for differentiating impulsive-aggressive dogs from dogs with anxiety disorders. In the following study, a dual goal was aimed at. First, increasing the knowledge in canine behavioural problems will safeguard the human-dog bond which is under pressure in case of aggressive behaviour. Aggression, but also anxiety leading to aggressive behaviour, results in a low quality of life and expands the previously mentioned welfare issues to a safety issue as well. A second goal was to further investigate the value of behaviourally disordered dogs as a model for human behavioural disorders. Previous SPECT research already pointed out the value of this canine model in diagnostic and therapeutic aspects of impulsive-aggressive, anxiety-disordered and compulsive-disordered dogs (Peremans et al. 2003, 2005; Vermeire et al. 2009a, b, 2010, 2012).
Sixty-six dogs were included and divided in three equally sized groups, i.e. 22 dogs with impulsive-aggressive behaviour (18 M (7 neutered), 4 F (all intact); mean age 2.73 years; range 12–84 m; breeds included are 3 Rottweilers, 3 Belgian shepherds, 2 Berger de Beauce, 2 golden retrievers, 2 Great Danes, 2 Labrador retrievers, 2 Dobermans, 1 Caucasian shepherd, 1 Jack Russell, 1 bull mastiff, 1 pit bull, 1 mongrel and 1 English bulldog), 22 dogs with severe anxiety (18 M (10 neutered), 4 F (2 neutered); mean age 3.67 years; range 14–86 m; breeds included are 4 mongrels, 3 golden retrievers, 3 Border collies, 2 German shepherds, 1 Staffordshire bull terrier, 1 Dutch Decoy Dog, 1 Berger de Beauce, 1 Bernese mountain dog, 1 Bleu de Gascogne, 1 Bordeaux dog, 1 boxer, 1 English bulldog, 1 miniature pinscher and 1 Shar Pei), and 22 normally behaving dogs (14 M (3 neutered), 8 F (all intact); mean age 3.17 years; range 12–84 m; breeds included are 4 German shepherds, 4 mongrels, 3 Border collies, 3 Belgian shepherds, 2 Boerboels, 2 Dutch Decoy Dogs, 1 dachshund, 1 French bulldog, 1 Rottweiler and 1 Labrador retriever). As in the previous study, ECVBM specialists selected the patients and categorised them based on behavioural analysis and a validated canine behavioural questionnaire CBARQ (Hsu and Serpell 2003). Possible neurological or physical illnesses were excluded (Haug 2008; Sherman and Mills 2008).
Age correction was performed for previously mentioned reasons. The comparison of the 5-HT2A receptor binding revealed significant changes in both impulsive-aggressive and anxious behaving dogs in the frontal, temporal and occipital cortices (both hemispheres). Those changes were in opposing directions, with increased 5-HT2A receptor binding for the impulsive-aggressive dogs and decreased 5-HT2A receptor binding for the anxiety-disordered dogs, compared to the normal group. Brain perfusion changes were again excluded as the cause of the altered serotonin 2A receptor binding indices as no correlations were found between perfusion and radioligand data. The receiver operating characteristic (ROC) analysis of the data determined that the cut-off values for the frontal cortex had the highest statistical power to differentiate the separate groups (Fig. 26.6), a not surprising observation as the (pre)frontal cortex has been extensively identified as the key regulating region in behaviour (Fuster 1997). This region also contains the highest density of 5-HT2A receptors of the brain in the human and canine species (Busatto et al. 1997; Peremans et al. 2002b), strengthening the role of the frontal cortex in behaviour. It is essential to realise that the proposed cut-off value is not rigid and a higher or lower cut-off value can be chosen depending on whether priority is given to risk assessment or to animal welfare issues. In case of risk assessment, one would prefer a high sensitivity (to avoid impulsive-aggressive animals ending in families); however, this would be at the expense of the specificity (more nonaggressive animals being labelled as impulsive aggressive). In case of animal welfare, one would prioritise a lower sensitivity with a higher specificity to avoid unnecessary euthanasia (Vermeire et al. 2011).
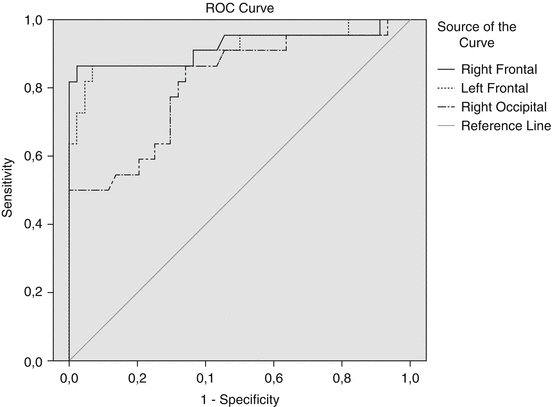
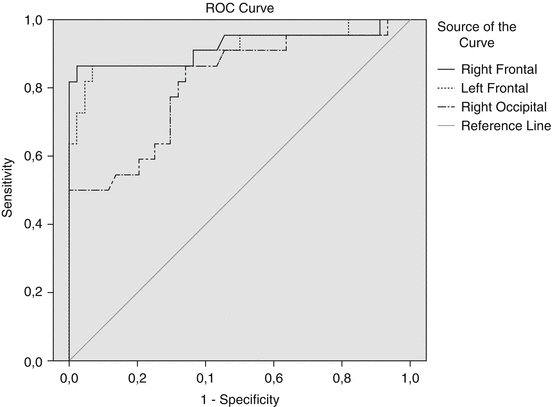
Fig. 26.6
Receiver operating curves (ROC) are shown for the group of impulsive-aggressive dogs. The sensitivity (true-positives) is plotted against (1-specificity) (the false-positives) across a range of cut-off values. The reference curve represents the characteristics of a test that is unable to differentiate the two groups. The curve of the ideal test would go straight up to a sensitivity of 1 (left top corner) before moving to the right (right top corner). Binding indices of the right and left frontal cortex as well as the right occipital region are shown as examples of two valuable curves (frontal) and a less useful curve (occipital). The curves of the other brain regions are not shown because of their low sensitivity and specificity
Variations in the 5-HT2A receptor gene have been suggested to play a role in anxiety and impulsive traits in man and could in theory affect radioligand binding due to conformational or functional alterations (Bjork et al. 2002; Giegling et al. 2006; Nomura et al. 2006; Unschuld et al. 2007). However, no variants in the HTR2A gene were detected in aggressive golden retrievers, making a genetic modification as the cause of alterations found in the impulsive-aggressive dogs less likely (van den Bergh et al. 2008). No comparative studies have been performed in the anxiety-disordered dog up till now.
A correct differentiation of the underlying 5-HT2A receptor imbalance is important in view of psychopharmacological and behavioural treatment choice (Moresco et al. 2007; Peremans et al. 2008; Vermeire et al. 2010; Zanardi et al. 2001). Hereby, the 5-HT2A receptor neuroimaging and semiquantification is a more objective means to differentiate behaviour according to its biological underpinnings, in addition to the more subjective behavioural analysis.
26.3 Selective Serotonin Reuptake Inhibitors in the Treatment of Impulsive-Aggressive Behaviour in Dogs
SSRIs are currently used to manage impulse control disorders and/or aggression in man and dogs (Dodman et al. 1996; Fuller 1996). In a pilot SPECT study the effect of a single dose of citalopram on the canine serotonin reuptake transporters (SERT) was evaluated with the radioligand [123I]-labelled 2 h-carboxymethoxy-3 h-(4-iodophenyl)tropane (123I β-CIT), a nonselective competitive antagonist of norepinephrine, dopamine and serotonin transporters (Peremans et al. 2006) (Fig. 26.7a, b). A decreased binding was observed compared to the blank scan, providing proof that the SSRI, citalopram, binds to the canine serotonin reuptake transporters.
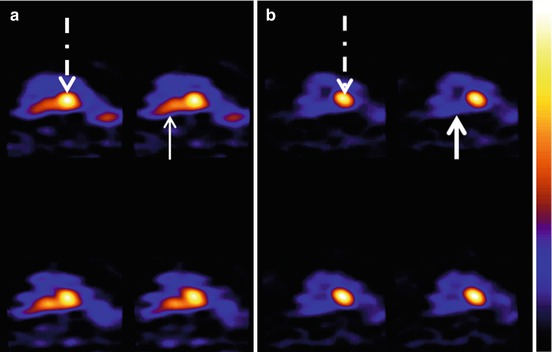
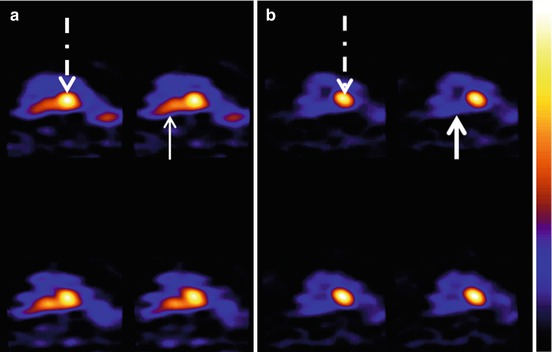
Fig. 26.7
SERT and DAT imaging with 123I-β-CIT in a normal dog before (a) and after (b) administration of the SSRI Cipramil. Note the decreased activity in the area of the raphe nuclei where SERT are abundantly present (thin arrow). After blockade of the SERT by Cipramil, radiopharmaceutical uptake decreases substantially in this same area (thick arrow). The interrupted arrow points to the uptake of the radioligand in the basal ganglia, an area rich in dopamine transporters
Nine impulsive-aggressive dogs were then treated daily with SSRIs (citalopram, 1 mg/kg) and were re-examined at 6 weeks of treatment (no behaviour therapy was allowed in this period). Both perfusion and 5-HT2A receptor radioligand binding were evaluated, and behavioural changes were assessed before and after treatment.
After treatment, a significant decreased binding of the 5-HT2A radioligand was evident in the frontotemporal and to a lesser extent in the occipital area without alterations in regional perfusion (Peremans et al. 2005). These findings show also resemblance with the results of a 5-HT2A receptor PET study in depressed patients, where decreased binding of 18F-setoperone was found in the cortex of young patients with depression, treated for 6 weeks with paroxetine (Meyer et al. 2001). An increased synaptic serotonin concentration due to chronic blockade of the SERT seems the most logical explication for the observed decreased radioligand binding, the decrease being invoked by either compensatory downregulation of the receptors or competition of endogenous serotonin with the radioligand. The extent of clinical behavioural recovery paralleled the extent of reduction of 5-HT2A binding index. The possibility has been raised that even though downregulation of the receptors may not be a therapeutic effect in itself, the decreased numbers of 5-HT2A receptor may reduce the agonistic impact on these receptors (Meyer et al. 2001). More, it is well known in human depression that a lag period exists between initiation of SSRI treatment and the onset of their antidepressive effect. Initial agonistic activation of the 5-HT2A receptor by blocking the SERT has been pointed at as one of the culprits for this phenomenon. In this regard, augmentation with a 5-HT2A-receptor antagonist has been proposed as a potential alternative treatment strategy (Nemeroff 2005).
This study represents a nice example of the potency of functional imaging to evaluate receptor alterations after drug interventions in the canine brain and the therapeutic impact of psychopharmaca.
26.4 Confounding Factors in Canine Functional Brain Imaging Studies
26.4.1 Anaesthesia
In contrast to human studies, anaesthesia is generally required to perform animal imaging studies, although some recent studies report on investigations in the awake animal, employing fixing devices (Hassoun et al. 2003; Kakiuchi et al. 2000; Tokunaga et al. 2009). Sedatives and anaesthetics in general affect respiratory and cardiovascular dynamics and as such can influence peripheric tracer kinetics (Kersemans et al. 2006). In case of neuroimaging, interaction of the anaesthetics with the cerebral neurotransmission and metabolism may be an additional important confounder. Furthermore, through its influence on blood pressure and its effect on the ventilation, it has an impact on arterial carbon dioxide tensions and arterial oxygen tensions as well. All the aforementioned parameters, as well as the direct effect of anaesthetics on the cerebral blood vessels, can affect the (regional) cerebral blood flow (Waelbers et al. 2010). Due to those, sometimes opposing effects, the ultimate result of anaesthesia on brain imaging studies is difficult to predict.
Although the influence of anaesthetics on brain imaging studies should never be neglected, it can sometimes be avoided. When performing SPECT brain perfusion studies, for instance, retention tracers can be used. After intravenous injection, these tracers are trapped in the cerebral neurons with a distribution pattern resembling the regional cerebral blood flow at the moment of the injection. A very interesting feature of these tracers is that their distribution is not altered by anaesthetic-induced brain perfusion changes occurring after tracer administration. Like in humans, the duration of this fixed distribution is long enough in dogs and cats as well, to allow induction of anaesthesia followed by the acquisition (Waelbers et al. 2012, 2012a). This creates the possibility to visualise the brain perfusion without interference of the anaesthetics. In some situations, however, sedation or anaesthesia is required prior to tracer injection. This leads inevitably to global and regional perfusion alterations that have to be taken into account (Waelbers et al. 2011, 2012b).
Tracers that need to be injected simultaneous with the start of the acquisition and neuroreceptor radioligands, requiring acquisition during an equilibrium state, are more sensitive to anaesthetic-induced alterations. When tracers are used in animals under anaesthesia, blood flow changes are only one confounder (Lee et al. 2012). Other factors to consider are possible interactions of anaesthetics with the release and/or reuptake of neurotransmitters and direct actions of the anaesthetics on the neuroreceptors (Adriaens et al. 2012; Hassoun et al. 2003; Waelbers 2012b).
In conclusion, it can be stated that the influence of anaesthesia on functional brain imaging should not be omitted and that careful selection of sedatives, anaesthetic agents and anaesthetic techniques is required. Furthermore fixed anaesthetic protocols (agents and dosages) should be used throughout all studies.
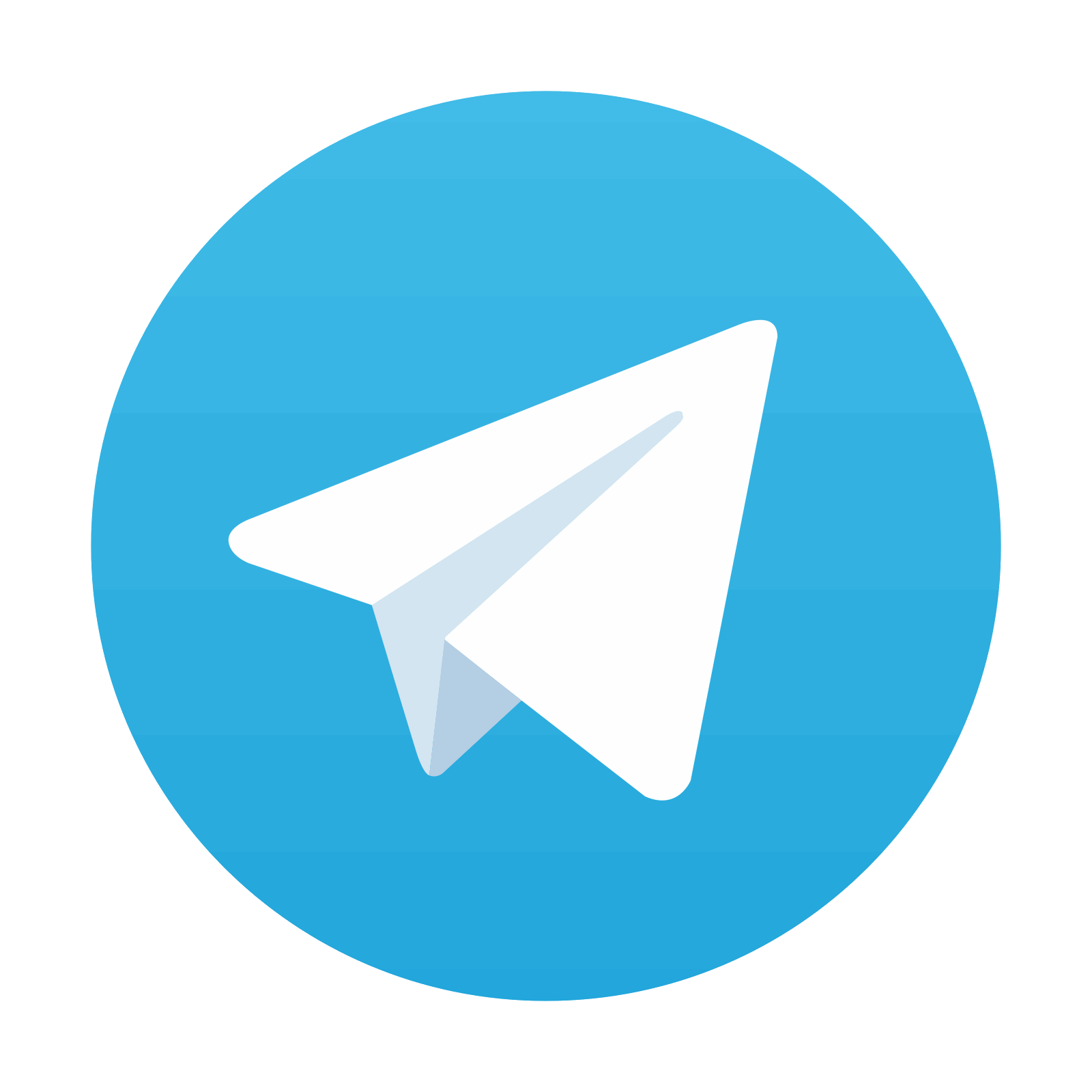
Stay updated, free articles. Join our Telegram channel

Full access? Get Clinical Tree
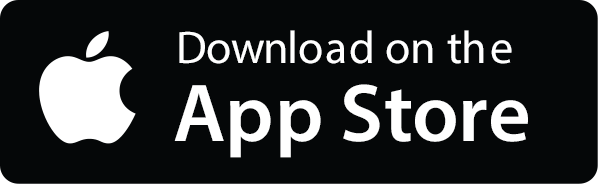
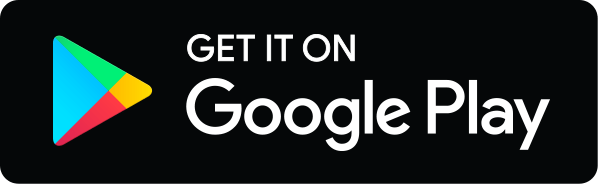