2577 Brief Rhythmic Discharges, Seizures, and Mimics in Critically Ill Neonates LEARNING OBJECTIVES • To recognize and interpret ictal and interictal activity in neonatal EEG • To become familiar with factors that influence clinical and electrographic aspects of neonatal seizures • To understand the definition and clinical relevance of neonatal status epilepticus • To recognize EEG patterns of uncertain significance as well as patterns mimicking seizures Introduction The majority of neonatal seizures (NS) are symptomatic, and they are often the first sign of neurologic injury in the newborn. Their early recognition provides an opportunity to diagnose and treat, or ameliorate, the underlying disorder. While it remains controversial whether NS confer additional neurologic injury, they can disrupt cardiorespiratory control in already unstable infants. In addition to management of the underlying etiology, treatment with antiseizure medication (ASM) is undertaken in all but rare cases. Establishing the incidence of NS has been complicated by varying methods of seizure diagnosis, (e.g., clinical diagnosis alone vs. electrographic diagnosis), varying modalities used to detect NS electrographically (e.g., amplitude integrated EEG [aEEG] vs. continuous video EEG [cvEEG]), and the impracticality of routinely monitoring all neonates at high risk. General estimates suggest that NS occur in 1% to 8% of patients in the neonatal intensive care unit (NICU) and in 1 to 3.5 per 1,000 live births.1,2 A recent population-based study in Parma, Italy using EEG confirmation for diagnosis of NS yielded an incidence of 2.29/1,000 live births, with 1.10/1,000 in full term and 14.28/1,000 in preterm births. Incidence increased with decreasing gestational age (up to 85.6/1,000 live births <28 weeks gestation).3 In high-risk neonates undergoing EEG monitoring in the NICU, electrographic seizures (ES) are found in 26% to 82% of patients.4 Hypoxic-ischemic encephalopathy (HIE) is the most common cause of NS, followed by arterial ischemic stroke and intracranial hemorrhage.5 Seizures associated with HIE typically start within the first 24 hours of life in infants with clinical evidence of encephalopathy, whereas seizures associated with infectious or metabolic etiologies often occur after an initial period of apparent well-being. In recent cohort studies, males show a higher incidence of seizures than females for unknown reasons.5 Historically, NS have been considered a marker of the severity of the underlying brain injury and have not been thought to have an independent deleterious effect. However, in HIE and other types of acute brain injury, there is the potential for additional seizure-related excitotoxic injury in vulnerable penumbral regions.6,7 Accumulating clinical evidence suggests that NS may independently worsen neurodevelopmental outcome and play a role in later epileptogenesis.8,9 The increased availability of continuous bedside video EEG monitoring in the NICU is now making it possible to accurately characterize the frequency, duration, and topography of NS. This should lead to a better understanding of the relationship of NS to neurodevelopmental outcome. NS are associated with an overall mortality of ~17%, and neonates who do not respond to first-line medications are twice as likely to die as neonates who do respond.5 In addition, survivors of NS have a high rate of neurodevelopmental disability including cerebral palsy (~30%), intellectual disability (~35%), and epilepsy (~15%), with the underlying etiology most predictive of outcome.3 Treatment of NS can be challenging, as up to two-thirds of neonates with seizures do not respond to first-line medications and one-half do not respond to second-line medications.5 Treatment strategies have not changed substantially over decades despite the availability of many new antiseizure medications.10,11 Phenobarbital and phenytoin/fosphenytoin remain standard choices. Newer drugs, such as levetiracetam, have failed to show superiority over the traditional agents. The search for new medications and treatment strategies is an area of active research with the goal of early seizure cessation and improved long-term neurodevelopmental outcomes. The natural history of acute symptomatic seizures, which tend to resolve after a few days, complicates the assessment of the efficacy of new agents as any medication added second line may coincide with the natural decline in seizure frequency. 258Sharp Waves and Brief Rhythmic Discharges Sharp Wave Transients In the newborn, unlike at other stages of development, sporadic sharp wave transients occur as a normal developmental EEG feature during brain maturation. They are most abundant at 32 to 34 weeks, decreasing gradually in frequency through term, at which point they should be rare (≤2–3/hour), of modest amplitude and duration (<75 µV, 100–200 ms), located in the central, temporal, or centro-temporal region, and only seen during quiet sleep.12 When they are more frequent than normal, they are considered a manifestation of encephalopathy and not an indication of a lowered seizure threshold. The American Clinical Neurophysiology Society (ACNS) guideline on EEG terminology in neonates states that sharp wave transients are excessive when there are >11/hour in the term newborn and >13/hour in the preterm newborn.12 There are other features of neonatal sharp wave transients that suggest they are abnormal, and in some cases the argument can be made that they may in fact be epileptiform or suggestive of a structural lesion. These features include high amplitude (>150 mV), positive polarity, focal persistence, occurrence in focal runs, complex morphology with a large after-going slow wave, and presence in continuous portions of the record (e.g., active sleep and waking) (Figure 7.1A–D).12 Positive Sharp Waves Positive sharp wave transients (PSW) located in the central or vertex region (also referred to as positive rolandic sharp waves) in the preterm infant have classically been associated with intraventricular hemorrhage (IVH)13,14 (Figure 7.2A–D). PSW are seen in 30% to 78% of patients with IVH.15,16 An association between PSW and periventricular leukomalacia has also been described.17 Location outside of the rolandic area, particularly in the temporal region, may also offer insight into cerebral injury; Chung and Clancy found an association of positive temporal sharp waves in preterm neonates with cerebral injury.18 Others have suggested that they represent a benign pattern similar to the temporal theta bursts seen in the premature infant.19 Brief Rhythmic Discharges Brief rhythmic discharges (BRDs) may be indicative of a lowered seizure threshold. These are also referred to as B(I)RDs—the “(I)” meant to convey either ictal or interictal occurrence and a degree of controversy as to their significance. In the newborn, they are currently defined as evolving rhythmic discharges of >2 µV amplitude and <10 seconds duration12 (Figure 7.3A–F). The requirement for evolution of the waveform has not always been included in the definition, however it is required as per the ACNS guideline definition. In 1990, Shewmon first characterized BRDs as a possible epileptiform pattern distinct from other physiologic (e.g., temporal theta/alpha bursts, delta “brushes”) or pathologic rhythmic discharges in the neonate.20 This and subsequent studies have indicated a prevalence in neonatal EEG recordings of up to 20% and have described associations with neonatal brain injury (particularly leukomalacia), electrographic seizures, increased mortality, and long-term neurodevelopmental disability.21,22 While they are generally not expected to have a clinical correlate, Oliveira et al. described a clinical correlate (focal clonic movements, focal tonic events, convulsive apnea, or motor automatisms) in ~16% of BRDs.22 Although less well-studied in adults, BRDs were reported to occur in a case-controlled series at a frequency of 2% in critically ill adult patients.23 In the critically ill adult population, BRDs were strongly associated with seizure occurrence and were more likely to occur in the first hour of monitoring than were seizures (75% vs. 25%), leading to a recommendation to continue monitoring in those in whom BRDs were noted early in the recording.23 While the same data do not exist regarding the timing of BRDs in newborn EEGs, the presence of BRDs should be noted and, depending on the clinical circumstances, their association with seizure and brain injury may suggest the advisability of continued monitoring. Neonatal Seizures Historically, clinical NS included events with or without associated electrographic seizure patterns. Now, and for the purposes of this chapter, only those clinical events that are associated with electrographic seizure patterns are considered NS.12 It is nonetheless possible that clinical seizures occur in the absence of detectable scalp EEG activity, as can occur in older children and adults. The clinical events most often associated with electrographic seizures include focal clonic, focal tonic, and myoclonic seizures. Neonates may also have electroclinical spasms.24 Orolingual and ocular movements can be seen at the onset or during seizure evolution.25 Autonomic phenomena, including apnea, tachycardia, hypertension, sialorrhea, and piloerection, often accompany NS or can themselves be the primary clinical manifestations of a seizure. As it is not possible to confidently assess awareness in newborns, seizures cannot be designated as with or without impaired awareness as in older children and adults. It is believed that, due to the immaturity of myelination, dendritic arborization, and synaptic development, the immature brain is unable to support “generalized” seizures;26 therefore, all NS are considered focal. Seemingly generalized clinical patterns are usually, in fact, multifocal with asynchronous bilateral semiology and may represent different seizures occurring simultaneously but 259independently over the left and right hemisphere. Exceptions include spasms and myoclonic seizures that are associated with diffuse voltage attenuation24 or an ictal burst-suppression pattern,27 respectively. The clinical seizure can be subtle, fragmentary, and brief compared to the electrographic seizure or may be lacking entirely (electrographic-only/subclinical). This is particularly true in neonates who have received anti-seizure medication. Electroclinical uncoupling/decoupling, a phenomenon in which treated electroclinical seizures lose their clinical correlate and become solely subclinical, has been well studied in neonates. Scher and colleagues found this to occur in 58% of a cohort of 59 neonates.28 The term electroclinical dissociation has been used interchangeably with uncoupling. We note that in some early studies the term electroclinical dissociation was used to refer to the lack of an EEG correlate of a clinical seizure due to the electrophysiologic characteristics of the seizure generator.29 Infants receiving neuromuscular blockers will also manifest solely EEG-only/subclinical seizures. Similarly, in an encephalopathic infant, all electrographic seizures may be subtle or subclinical (~16% in a recent cohort).5 For these reasons, conventional video EEG is the gold standard for the diagnosis of neonatal seizures.12,30,31 An electrographic NS is defined as a sudden EEG change lasting at least 10 seconds characterized by a repetitive, usually rhythmic, pattern with unequivocal evolution in frequency, voltage, waveform, and/or location (in contrast to older patients where evolution of all parameters is required), with a clear beginning, middle, and end, and a minimum voltage of 2 µV (peak to peak)12 (Figure 7.4A–C). Ictal patterns include rhythmic or semirhythmic spikes and sharp waves (Figure 7.5A–C) and/or sinusoidal waves of alpha, theta, or delta frequency (Figure 7.6A–F). Ictal discharges can be relatively monomorphic or can involve multiple patterns (Figure 7.7A–B). Different patterns may predominate in seizures from different regions in an individual patient.26 Unlike in children and adults, in neonates there is no minimum required frequency of discharges. Recurrent electrographic seizures should be separated by at least 10 seconds to be considered separate seizures. NS typically show less electrographic spread than seizures in children and adults, thought to be due to the immature state of myelination.32 Seizures usually arise from a single focus, but multiple foci discharging asynchronously can also occur (Figures 7.8A–E, 7.9, and 7.10). Location(s) and lateralization of seizure onset, frequency, pattern of spread, and presence or absence of a clinical correlate should be reported. Just as it remains uncertain whether NS confer additional brain injury in settings such as hypoxic-ischemic injury, it is also unclear how best to express seizure “burden.” Previously, and often in clinical practice, counting the number of seizures occurring during the period of monitoring has been the primary means of quantification. However, the duration of seizures (expressed as total duration/unit time), the maximum seizure frequency (maximum number of seizures/hour), proportion of the record in which seizures occur, and/or the temporal and spatial evolution (expressed in channels/seconds) may be more relevant to the potential for seizure-related injury. Each of these measures has been used in studies of NS and their treatment, though not all are practical in the clinical setting. Regardless of the method used, serial evaluation and quantification may be useful in assessing the temporal progression of seizure burden and/or the response to treatment. Effect of Gestational Age Ictal patterns are generally similar in term and preterm newborns, with some notable exceptions. Some authors have described a greater likelihood of sharp elements (e.g., sharp and slow waves, repetitive sharp waves) in the ictal patterns of term infants, whereas preterm infants are more likely to have seizures comprising rhythmic alpha or delta patterns.33,34 While seizure onset is focal in both term and preterm infants, regional onset (more than one adjacent electrode) may be more common in preterm infants.34 Centrotemporal location of seizure onset is most common in term and late preterm infants,33,35 while in preterm infants <32 weeks gestational age, occipital and frontal locations predominate.33,36 Shorter seizure duration has been described in preterm infants in some,37 but not all, studies34 (Figure 7.11A–D). Propagation of seizure is rare at <28 weeks gestational age34 and, if it occurs, is usually to the homologous contralateral region (i.e., central: central). In more mature newborns, propagation can occur to any region, ipsilateral or contralateral. Subclinical seizures occur at all gestational ages; some studies indicate a similar proportion of subclinical seizures at all gestational ages (~65%),38 while others suggest that a higher proportion of seizures are subclinical in preterm as compared to term neonates (75% vs. 51%).33 The occurrence of exclusively subclinical seizures was significantly greater in preterm infants (24%) than in term infants (14%) in the multicenter Neonatal Seizure Registry.5 Effect of Etiology Approximately 70% of NS are acute symptomatic due to hypoxic-ischemic injury, arterial ischemic stroke, or intracranial hemorrhage. In HIE, seizure onset is usually within the first 24 hours of life, often within the first 12 hours, with resolution after the first few days of life.39,40 In infants with severe encephalopathy, seizures are often subclinical. More than half of infants with arterial ischemic stroke present in the neonatal period, often with focal seizures that onset after 12 hours of life. Preterm infants with arterial ischemic stroke are less likely to have seizures but may present, instead, with respiratory difficulties, including apnea.41,42 Subdural hemorrhage and intraventricular hemorrhage (IVH) are the most common forms of intracranial hemorrhage in term and preterm newborns, respectively.43 Small subdural hemorrhages are common and usually benign; however, large hemorrhages can be associated with 260NS, often occurring on the second day of life.43 IVH with or without parenchymal extension is more common in preterm than in term infants and, when grade III and IV, often causes seizures in the first 3 days of life.44 Occurrence of IVH after the immediate postnatal period may also be associated with seizures in neonates;45 this is typically accompanied by other clinical signs of IVH. Seizures occurring after a 48-hour period of well-being should raise concern for infection or inborn errors of metabolism, which occur at about equal frequency in neonates.5 Little is known about the relationship of etiology to ictal clinical and electrographic patterns. A recent review27 suggested that infection (p =.042) and genetic disorders (p < .001) were associated with clonic seizures, metabolic/vitamin-related disorders with myoclonic seizures (p < .001), and vascular disorders with “sequential” seizures (evolving sequence of clinical features, p = .009). Focal discharges were more prevalent in vascular disorders (stroke and hemorrhage, p < .001) and burst suppression in genetic disorders (p < .001) as compared to other etiologies. Future studies should confirm and/or extend these associations. Effect of Therapeutic Hypothermia The reported incidence of seizures in HIE in the pre-versus posthypothermia era is similar, 22% to 64% and 34% to 65%, respectively.40,46,47 However, seizure burden (total duration of seizures/unit time) has been shown to be significantly reduced in hypothermia- as compared to nonhypothermia-treated infants with HIE, with a reduced seizure burden seen specifically in moderate but not severe HIE.9,40 Time of initial seizure onset is variable in hypothermia-treated infants. Although more than 50% of hypothermia-treated infants will have their initial seizure in the first 24 hours of life, a subset of infants will have their first seizure as late as 60 to 90 hours of life, some during or after rewarming.47,48 Seizures may also be shorter and more subtle in hypothermia-treated infants,40 making them more difficult to identify clinically; approximately 45% of hypothermia-treated infants in two small series had exclusively subclinical seizures.46,47 Hypothermia does not prevent status epilepticus, which continues to be documented in approximately 25% of hypothermia-treated infants with seizures.39 Lastly, hypothermia may be one factor, among others, responsible for a possible decrease in the rate of childhood epilepsy in survivors of acute symptomatic NS reported in some recent series.49,50 Neonatal Status Epilepticus The definition of status epilepticus (SE) presents particular difficulties in the newborn infant. The International League Against Epilepsy defines SE in children and adults as a state of prolonged seizures due to failure of normal termination processes or initiation of processes that lead to abnormally prolonged seizures.51 There are two clinically important time points: t1—the time at which a seizure should be considered “prolonged” and treatment should begin; and t2—the time at which irreversible injury may occur. For convulsive SE, t1 is 5 minutes and t2 is 30 minutes. For focal or nonconvulsive status epilepticus, it is less clear when these time points are reached, including in older children and adults. In newborns, where there is controversy as to whether seizures cause additional injury, the determination of these time points is also far from clear. An operational definition of SE as convulsive seizure ≥5 minutes or 2 or more discrete seizures between which there is incomplete recovery of consciousness is also difficult to apply to the neonate in whom convulsive seizures may be infrequent and assessment of consciousness is limited, especially in the presence of neonatal encephalopathy. Recent American Clinical Neurophysiology Society guidelines recommend that neonatal status epilepticus (NSE) be considered present when “the summed duration of (electrographic) seizures comprises ≥50% of an arbitrarily defined 1-hour epoch”12 (Figure 7.12A–F). NSE typically occurs in infants with moderate to severe encephalopathy.38 The frequency of electrographic SE in the NICU ranges from 14% to 43%,52 depending on the population studied and the definition of SE used. Diagnosis rests on EEG documentation, as the majority of seizures in NSE are subclinical. In the past, it was thought that SE occurred less commonly in neonates than in critically ill children. However, with wider use of prolonged video EEG recordings in critically ill newborns, the rates appear to be similar. SE occurs at about the same frequency (14%–15%) in preterm and term infants.38 In critically ill children, electrographic SE, but not electrographic seizures, has been associated with unfavorable outcome and greater risk of later epilepsy.53 In newborns, the association of NSE with poor outcome is also clearer than for NS. For example, NSE is associated with postneonatal epilepsy, with approximately 33% to 83% of neonates with SE later developing epilepsy.54,55 In a recent multicenter, prospective cohort of neonates with seizures,5 mortality was higher in patients with NSE than those without (26% vs. 15%). In the same study, mortality was higher in preterm than in term infants with SE (32% vs. 15%);5 these findings are similar to the results of a study by Pavlidis et al. showing 53% vs. 18% mortality in preterm versus term infants with SE.55 A single center study by Kharoshankaya et al. examined seizure burden and outcome in newborns with HIE. In this study, the presence of seizures alone was not associated with a poor outcome; however, the odds of a poor outcome increased ninefold for neonates with a total seizure burden >40 minutes (p = .001) and eightfold for neonates with a maximum 1 hour seizure burden >13 minutes (p = .003).56 Rhythmic and Periodic Patterns of Uncertain Significance The definitions of rhythmic/periodic patterns that are applied to the critical care EEG in children and adults can be applied to the neonate. We note that this is not explicitly stated in the critical care EEG terminology; however, 261the definitions of rhythmic and periodic patterns applied to the critical care EEG of children and adults are referenced in the ACNS guideline for standardized EEG terminology and description of continuous EEG monitoring in neonates.12,57 Caution should be taken in cases where a rhythmic pattern is identified in the neonate as slow, evolving patterns are accepted as seizure in the neonatal age group. Also, some normal features of the neonatal EEG (anterior dysrhythmia, monomorphic occipital delta) are both rhythmic and nonevolving. Rhythmic/periodic patterns that do not meet the criteria for seizure are rare in neonates, occurring in only 5% of neonatal EEG recordings58 (Figure 7.13A–B). In these cases, the patterns do not evolve in any of the domains required to meet the definition of a seizure. Periodic discharges with uniform morphology occurring at regular intervals are found most commonly in neonates with stroke, but also in neonates with herpes simplex virus encephalitis and hypoxic ischemic encephalopathy.58,59 Periodic discharges tend to occur in the parasagittal region in preterm neonates and in the temporal region in term neonates. Periodic discharges often occur in neonatal EEGs also containing seizures and are likely to outlast electrographic seizures in infants treated with antiseizure medication.58 Artifacts That Mimic Seizure As in older children, it is important to distinguish artifacts from electrocerebral activity (Figures 7.14 and 7.15). Artifacts due to respiratory movements and pulse and cardioballistic artifacts should be relatively constant and time-locked to the polygraphic monitoring of respiration and heart rate. Difficulty may arise when head or body movements, changes in position, or variations in pulse volume cause the artifact to fluctuate and mimic the evolution seen in ictal patterns. Video recording, when available, can be helpful in clarifying responsible clinical events. Single electrode artifacts with unusual (e.g., squared off) morphology are usually related to electrode impedance (Figures 7.16 and 7.17). The absence of a field, the lack of evolution, and an unusual persistence and duration are often helpful in distinguishing these patterns as artifact. Artifacts with a completely invariant interval may be due to an intravenous drip, especially when the intravenous line is located in a scalp vein. In the ICU environment, many different electronic monitoring and treatment devices may generate vibration or other movements that can be transmitted to the EEG wires/electrodes. A bedside assessment by an experienced technologist who can check and repair impedances and assess the impact of changing the head or EEG wire position or a visit by the EEG reader to the bedside may be most helpful in identifying the source of an unresolved artifact. Conclusion Seizures are common in the neonatal intensive care unit, and the majority of neonatal seizures are subclinical. Proper identification of seizures is important as it is suggested that neonates with high seizure burden and NSE have poorer developmental outcome and higher rates of postneonatal epilepsy. A strong understanding of the electrographic principles of NS and how they differ from important EEG background patterns and seizure mimics are necessary for proper interpretation of neonatal EEG. This chapter includes access to several videos with real time EEG that illustrate concepts and patterns. Please follow the following url and click onto the show chapter supplementary tab to access the videos: http://connect.springerpub.com/content/book/978-0-8261-4867-4/chapter/ch07 Video 7.1 (Neonatal subclinical seizure) Video 7.2 (Neonatal focal clonic seizure) Video 7.3 (Rocking artifact) KEY POINTS • In the newborn, sporadic sharp wave transients occur as a normal developmental EEG feature during brain maturation; in contrast, brief rhythmic discharges (BRDs) may be indicative of a lowered seizure threshold. • An electrographic neonatal seizure is defined as a sudden EEG change lasting at least 10 seconds characterized by a repetitive, usually rhythmic, pattern with unequivocal evolution in frequency, voltage, waveform, and/or location, with a clear beginning, middle, and end, and a minimum voltage of 2 µV (peak to peak). • Approximately 70% of neonatal seizures are acute symptomatic due to hypoxic-ischemic injury, arterial ischemic stroke, or intracranial hemorrhage. • The incidence of seizures in hypoxic-ischemic encephalopathy in the pre- as compared to posttherapeutic hypothermia era is similar; however, seizure burden has been shown to be significantly reduced by therapeutic hypothermia. • ACNS guidelines recommend that neonatal status epilepticus be considered present when “the summed duration of (electrographic) seizures comprises ≥50% of an arbitrarily defined 1-hour epoch.” 262Figures FIGURE 7.1. Abnormal sharp wave transients. (A) Bipolar montage. The EEG of a 1-day-old term female who presented with a suspected seizure. The black box highlights focal persistence of sharp waves in the left mid-temporal (T7) region during a continuous portion of the recording. The sharp waves persisted throughout the record, becoming periodic at times. The patient went on to develop left temporal seizures. Settings: LFF 1 Hz, HFF 70 Hz, sensitivity 10 µV/mm, time base ~15 seconds/page. (B) Bipolar montage. The EEG of a 7-day-old female, born at 38 weeks gestation, who presented with hyperbilirubinemia and apneic spells. The red arrows highlight persistent sharp waves in the left posterior temporal/occipital (P7/O1) region. Settings: LFF 1 Hz, HFF 70 Hz, sensitivity 10 µV/mm, time base ~15 seconds/page. (C) Average referential montage. The same EEG as in (B) shown in an average referential montage, highlighting the high voltage of the left posterior temporal/occipital (P7/O1) sharp waves (~130 µV, marked by the red and blue target symbol). Settings: LFF 1 Hz, HFF 70 Hz, sensitivity 10 µV/mm, time base ~15 seconds/page. (D) Bipolar montage. The EEG of a 2-day-old female, born at 38 weeks gestation, being treated with therapeutic hypothermia for hypoxic-ischemic encephalopathy. The black box highlights persistent runs of abnormal sharp waves in the right frontal (F4) region. There are also persistent single sharp waves in the left central (C3) region (red arrows) and periodic discharges in the right anterior temporal (F8) region (*). Settings: LFF 1 Hz, HFF 70 Hz, sensitivity 5 µV/mm, time base ~15 seconds/page. Source: (A–D) Images courtesy of Arnold Sansevere. FIGURE 7.2. Positive sharp waves. (A) Bipolar montage. The EEG of a 2-day-old male, born at 35 weeks gestation, with intraventricular hemorrhage and episodes of apnea and bradycardia. The * denotes a positive anterior temporal (F7) sharp wave. Settings: LFF 1 Hz, HFF 70 Hz, sensitivity 10 µV/mm, time base ~15 seconds/page. (B) Average referential montage. The same EEG as in (A) shown in an average referential montage, highlighting the positive polarity of the F7 sharp wave (red arrow). Settings: LFF 1 Hz, HFF 70 Hz, sensitivity 10 µV/mm, time base ~15 seconds/page. (C) Bipolar montage. The * denotes a positive mid-temporal (T7) sharp wave. Settings: LFF 1 Hz, HFF 70 Hz, sensitivity 10 µV/mm, time base ~15 seconds/page. (D) Average referential montage. The same EEG as in (C) is shown in an average referential montage, highlighting the positive polarity of the T7 sharp wave (red arrow). Settings: LFF 1 Hz, HFF 70 Hz, sensitivity 10 µV/mm, time base ~15 seconds/page. Source: (A–D) Images courtesy of Arnold Sansevere. FIGURE 7.3. Brief rhythmic discharges. (A) Bipolar montage. The EEG of a 20-day-old female, born at 40 weeks gestation, who presented with apnea, somnolence, and a suspected metabolic disorder. A brief rhythmic discharge (BRD) starts in the left parasagittal (C3/P3) region (red arrow) and lasts for 5 seconds. Note the spread anteriorly to the left frontal (F3) region and to the posterior midline (Pz) (blue arrows). Settings: LFF 1 Hz, HFF 70 Hz, sensitivity 7 µV/mm, time base ~15 seconds/page. (B) Bipolar montage. EEG from the same patient as in (A) showing an electrographic seizure in the same left parasagittal region less than 1 hour later. Red arrows denote the beginning and end of the seizure. Note surface positivity at C3, with one of the recurring surface positive discharges denoted by the *. Settings: LFF 1 Hz, HFF 70 Hz, sensitivity 7 µV/mm, time base ~15 seconds/page. (C) Bipolar montage. The EEG of a 7-day-old female, born at 38 weeks gestation, who presented with hyperbilirubinemia and apneic spells. A BRD starts as low-amplitude fast activity over the left occipital (O1) region (red arrow) and then spreads anteriorly to the left parasagittal (P3) and posterior temporal (P7; blue arrow) regions before increasing in amplitude (black arrow). Settings: LFF 1 Hz, HFF 70 Hz, sensitivity 15 µV/mm, time base ~15 seconds/page. (D) Bipolar montage. The EEG of a 1-day old, born at 40 weeks gestation, undergoing therapeutic hypothermia for hypoxic ischemic encephalopathy. A BRD starts with a sharply contoured waveform over the right central (C4) region (red arrow) and spreads to the midline (Cz) (blue arrow) before slowing in frequency (black arrow). This pattern lasts for approximately 8 seconds. Settings: LFF 1 Hz, HFF 70 Hz, sensitivity 5 µV/mm, time base ~15 seconds/page. (E) Average referential montage. The same EEG as in (D) shown in an average referential montage, highlighting the field to the right parietal (P4) region and to the central midline (Cz) (*). Settings: LFF 1 Hz, HFF 70 Hz, Notch 60 Hz, sensitivity 5 µV/mm, time base ~15 seconds/page. (F) Neonatal montage. The EEG of a 1-day-old term female treated for neonatal seizures while undergoing therapeutic hypothermia for hypoxic ischemic encephalopathy. A BRD begins with fast activity in the left temporal (T3) region (red arrows), which increases in amplitude (blue line) and slows in frequency (black line) over 3 seconds. Settings: LFF 1 Hz, HFF 70, sensitivity 5 µV/mm, ~20 seconds/page. Source: (A–E) Images courtesy of Arnold Sansevere; (F) image courtesy of Tammy Tsuchida. FIGURE 7.4. Beginning, middle, and end of left frontal midline seizure. The EEG of a 1-day-old male, born at 37 weeks gestation, who presented with apnea, right arm tonic posturing, and rightward eye deviation. MRI revealed a left frontal hemorrhagic infarct. (A) Bipolar montage. The seizure begins with surface positive midline (Cz) discharges (red arrow). Settings: LFF 1 Hz, HFF 70 Hz, sensitivity 3 µV/mm, time base ~15 seconds/page. (B) Bipolar montage. Prominent surface positive midline discharges continue (*). Note the field to the left frontal (Fp1, F3) region (black arrow), concordant with the clinical semiology and left-sided hemorrhagic infarct. Settings: LFF 1 Hz, HFF 70 Hz, sensitivity 3 µV/mm, time base ~15 seconds/page. The EEG of a 1-day-old male, born at 37 weeks gestation, who presented with apnea, right arm tonic posturing, and rightward eye deviation. MRI revealed a left frontal hemorrhagic infarct. (C) Bipolar montage. The seizure ends; the last recognizable waveform >2 µV is denoted by the red arrow. Settings: LFF 1 Hz, HFF 70 Hz, sensitivity 3 µV/mm, time base ~15 seconds/page. FIGURE 7.5. A seizure demonstrating evolution of waveform, field, and frequency. The EEG of a 1-day old, born at 39 weeks gestation, who presented with a right upper extremity clonic seizure. (A) Bipolar montage. The seizure begins with ~2 to 3 Hz sharp waves (red arrow) evolving over the left central region (C3). Settings: LFF 1 Hz, HFF 70 Hz, sensitivity 10 µV/mm, time base ~15 seconds/page. (B) Bipolar montage. As the seizure continues to evolve, low-amplitude spikes appear in the left central (C3) region (red arrow) and spread to the midline (Cz) (blue arrow). Fast spikes embedded in delta (or theta) waves were described as a “mitten pattern” by Gibbs and Gibbs in 1963. Settings: LFF 1 Hz, HFF 70 Hz, sensitivity 10 µV/mm, time base ~15 seconds/page. (C) Bipolar montage. With further evolution, prominent left centroparietal (C3, P3) and midline (Cz, Pz) spikes are seen (red arrows). Settings: LFF 1 Hz, HFF 70 Hz, sensitivity 10 µV/mm, time base ~15 seconds/page. FIGURE 7.6. Other neonatal seizure patterns. (A) Bipolar montage. The EEG of a 4-month old, born at 22 and 5/7 weeks gestation, who presented with episodes concerning for seizure. A seizure starts with sinusoidal alpha and theta activity over the left temporal (T7) and right frontopolar (Fp2) regions (red arrows) and evolves to theta/delta activity with embedded spikes (blue arrow). Settings: LFF 1 Hz, HFF 70 Hz, sensitivity 7 µV/mm, time base ~15 seconds/page. (B) Bipolar montage. EEG from the same patient as in (A) showing another seizure manifest as 7 to 8 Hz sinusoidal activity over the right frontopolar (Fp2) and right temporal (F8, T8, P8) regions (red arrows) with spread to the midline frontal (Fz) and left posterior temporal (P7) regions (blue arrows). Settings: LFF 1 Hz, HFF 70 Hz, sensitivity 5 µV/mm, time base ~15 seconds/page. (C) Bipolar montage. The EEG of a 1-day old, born at 38 weeks gestation with poor respiratory effort and hypotonia undergoing therapeutic hypothermia. A seizure starts with a sharp wave over the left central (C3) region (red arrow). This is followed by rhythmic delta activity that spreads to the central and posterior midline (Cz, Pz) (black arrow) and to the left parietal (P3) region (blue arrow). Settings: LFF 1 Hz, HFF 70 Hz, sensitivity 7 µV/mm, time base ~15 seconds/page. (D) Linked ears referential montage. The same seizure as in (C) is shown on a linked ears referential montage, highlighting the field involving C3, Cz, Pz, P3 (*). Settings: LFF 1 Hz, HFF 70 Hz, sensitivity 7 µV/mm, time base ~15 seconds/page. (E) Bipolar montage. EEG from the same patient as in (C–D) showing a seizure manifest as sharply contoured 1 to 2 Hz delta activity beginning over the right central (C4) region (red arrow) and spreading to the central midline (Cz) (blue arrow). Settings: LFF 1 Hz, HFF 70 Hz, sensitivity 7 µV/mm, time base 15 seconds/page. (F) Linked ears referential montage. The same seizure as in (E) is shown on a linked ears referential montage, highlighting the field involving C4, Cz (*). Settings: LFF 1 Hz, HFF 70 Hz, sensitivity 7 µV/mm, time base 15 seconds/page. Source: (A–F) Images courtesy of Arnold Sansevere. FIGURE 7.7. A seizure manifesting diverse patterns during its evolution. The EEG of a 5-day-old male, born at 40 weeks gestation, who presented with recurrent apnea and hyperbilirubinemia. (A) Bipolar montage. The seizure begins with sharp waves over the right posterior temporal (P8) region (red arrows) and spreads to the bioccipital regions (O1, O2) (blue arrows). Settings: LFF 1 Hz, HFF 70 Hz, sensitivity 10 µV/mm, time base ~15 seconds/page. (B) Bipolar montage. As the seizure evolves, bursts of alpha frequency activity are seen over the right temporal (T8, P8) and bioccipital regions (O1, O2) (red arrows). Settings: LFF 1 Hz, HFF 70 Hz, sensitivity 10 µV/mm, time base ~15 seconds/page. FIGURE 7.8. A seizure demonstrating spread to the central region. The EEG of a 1-day-old female, born at 38 weeks gestation with a prenatally-diagnosed vein of Galen malformation, who presented with respiratory distress. (A) Bipolar montage. Right frontal (F4) seizure onset (red arrow) with 1 to 2 Hz discharges and spread, 5 to 6 seconds later, to the left central (C3) region (blue arrow). Settings: LFF 1 Hz, HFF 70 Hz, 3 µV/mm, time base ~15 seconds/page. (B) Bipolar montage. The seizure continues independently over the left hemisphere as sharp waves maximal over the left central (C3) region with a field to the left parietal (P3) region (blue arrow). The right hemisphere seizure continues as right frontal (F4) sharp waves that evolve in frequency (red arrow). Settings: LFF 1 Hz, HFF 70 Hz, 3 µV/mm, time base ~15 seconds/page. (C) Bipolar montage. The seizure ends on the left (blue arrow) and continues on the right (red arrow). Settings: LFF 1 Hz, HFF 70 Hz, 3 µV/mm, time base ~15 seconds/page. (D) Bipolar montage. The seizure continues over the right frontal (F4) and central (C4) and midline (Cz) regions (red arrow). Settings: LFF 1 Hz, HFF 70 Hz, 3 µV/mm, time base ~15 seconds/page. (E) Bipolar montage. The seizure ends on the right (red arrow). Note that the background is discontinuous and the seizure is low in voltage (sensitivity at 3 µV/mm). Settings: LFF 1 Hz, HFF 70 Hz, 3 µV/mm, time base ~30 seconds/page. FIGURE 7.9. Independent bitemporal seizures. Bipolar montage. The EEG of a 1-day-old female, born at 39 weeks gestation with pregnancy and delivery complicated by in utero drug exposure and prolonged partial hypoxic ischemic injury, who presented with apnea, lip smacking, and refractory electroclinical seizures. An ongoing left temporal (F7, T7, P7) seizure and independent onset of a right temporal (F8, T8, P8) seizure (red arrow) are shown. Settings: LFF 1 Hz, HFF 70 Hz, sensitivity 3 µV/mm, time base ~15 seconds/page. FIGURE 7.10. A seizure demonstrating evolution at high voltage. Bipolar montage. The EEG of a 6-day-old male, born at term, who presented with dysmorphic features, hypotonia, and apnea. He was diagnosed with a peroxisomal disorder. An electrographic seizure is seen evolving in the left posterior quadrant (P3/P7/O1) (red arrow) to very high voltage (*) (>500 µV at O1/P3; note sensitivity of 15 µV/mm). Settings: LFF 1 Hz, HFF 70 Hz, sensitivity 15 µV/mm, time base ~15 seconds/page. FIGURE 7.11. Prolonged focal seizure in preterm infant. The EEG of a 6-day old, born at 30 weeks gestation, with a tracheoesophageal fistula and intraventricular and left temporal parenchymal hemorrhages. (A) Bipolar montage. Seizure onset is seen over the left parietal/posterior temporal region (P3/P7) as sharp waves (red arrow) with evolution to T7 (blue arrow). Settings: LFF 1 Hz, HFF 70 Hz, sensitivity 20 µV/mm, time base ~30 seconds/page. (B) Bipolar montage. One minute after onset, seizure activity is ongoing over the left mid-temporal (T7) region (blue arrow). Settings: LFF 1 Hz, HFF 70 Hz, sensitivity 20 µV/mm, time base ~30 seconds/page. (C) Bipolar montage. As the seizure continues 2.5 minutes after onset, there is evolution of wave form morphology (blue arrow) and frequency (red arrow). Settings: LFF 1 Hz, HFF 70 Hz, sensitivity 20 µV/mm, time base ~30 seconds/page. (D) Bipolar montage. The seizure ends after 12 minutes after continued evolution in frequency (blue arrow). Settings: LFF 1 Hz, HFF 70 Hz, sensitivity 20 µV/mm, time base ~30 seconds/page FIGURE 7.12. Neonatal status epilepticus. The EEG of a 1-day old, born at 38 weeks gestation, with perinatal asphyxia treated with therapeutic hypothermia. (A) Bipolar montage. Onset. Seizure onsets at the midline (Cz) (red arrow) as positive spikes with a frequency of 1 to 1.5 Hz (*). Settings: LFF 1 Hz, HFF 70 Hz, sensitivity 10 µV/mm, time base ~15 seconds/page. (B) Bipolar montage. Evolution. The seizure evolves in voltage, frequency (to 2 Hz), and location with spread to the left centroparietal (C3/P3) region (red arrow). Settings: LFF 1 Hz, HFF 70 Hz, sensitivity 10 µV/mm, time base ~15 seconds/page. (C) Bipolar montage. Evolution. The seizure continues to evolve in voltage, morphology, and location with spread to the left anterior temporal (F7) and left frontal (F3) regions (red arrows). Settings: LFF 1 Hz, HFF 70 Hz, sensitivity 10 µV/mm, time base ~15 seconds/page. (D) Bipolar montage. Resolving. The seizure begins to slow in frequency to 1 Hz. Settings: LFF 1 Hz, HFF 70 Hz, sensitivity 10 µV/mm, time base ~15 seconds/page. (E) Bipolar montage. Resolving. There is a decrease in voltage. Settings: LFF 1 Hz, HFF 70 Hz, sensitivity 10 µV/mm, time base ~15 seconds/page. (F) Bipolar montage. Abrupt end. The seizure ends abruptly (red arrow). The seizure lasted 35 minutes and was associated with right arm clonic movements at the start of the seizure that spread to the right face and leg. Settings: LFF 1 Hz, HFF 70 Hz, sensitivity 10 µV/mm, time base ~15 seconds/page. Source: (A–F) Images courtesy of Arnold Sansevere. FIGURE 7.13. Periodic patterns. (A) Bipolar montage. Lateralized periodic discharges. The EEG of a 5-day old, born at 40 weeks gestation, who presented with recurrent apnea and hyperbilirubinemia. Periodic discharges are seen over the right frontal (F4) region (red arrows). Settings: LFF 1 Hz, HFF 70 Hz, sensitivity 10 µV/mm, time base ~15 seconds/page. (B) Bipolar montage. Bilateral independent lateralized periodic discharges. The EEG of a neonate, born at 35 weeks gestation, with hyperbilirubinemia and abnormal movements. The EEG shows bilateral independent periodic discharges. The bilateral independence of the discharges is highlighted by the red lines. The fixed interval between discharges is highlighted by the*. This pattern did not evolve. Settings: LFF 1 Hz, HFF 70 Hz, sensitivity 7 µV/mm, time base ~15 seconds/page. Source: (A–B) Courtesy of Arnold Sansevere. FIGURE 7.14. “Jittering” artifact. Bipolar montage. The EEG of a 1-day old, born at term, with hypoxic ischemic encephalopathy treated with therapeutic hypothermia. No clinical or electrographic seizures were seen. Apparently evolving wave forms are seen over the right central (C4) region (red arrow); these wax and wane with oscillatory jittering of the left arm. The infant is lying on his right side. Settings: LFF 1 Hz, HFF 70 Hz, sensitivity 7 µV/mm, time base ~15 seconds/page. FIGURE 7.15. Patting artifact. Bipolar montage. The EEG of a neonate born at 37 weeks gestation with pregnancy and delivery complicated by intrauterine growth restriction and emergency Cesarean section for poor fetal biophysical profile, who presented with episodes concerning for seizure. BRD-like patting artifact is seen maximally over the right occipital (O2) (red arrows) and right central (C4) regions. Settings: LFF 1 Hz, HFF 70 Hz, sensitivity 7 µV/mm, time base ~15 seconds/page. FIGURE 7.16. Electrode “pop” artifact. Bipolar montage. The EEG of a 2-day old, born at 40 weeks gestation, who presented with right arm jerking. Electrode “pops” are seen over the left central (C3) and left posterior temporal (P7) (red arrows) regions, and an “evolving” run of pops is seen over the left anterior temporal (F7) region (blue arrow). Settings: LFF 1 Hz, HFF 70 Hz, sensitivity 5 µV/mm, time base ~15 seconds/page. FIGURE 7.17. EKG and electrode “pop” artifact. Bipolar montage. A term neonate with a prenatally-diagnosed vein of Galen malformation, who presented with respiratory distress. Electrographic seizures were identified on video-EEG monitoring. Multiple artifacts were also noted, including electrode artifact over the left frontopolar (Fp1) region with the appearance of mild evolution (red arrow) and EKG artifact in the right temporal (F8, T8, P8) leads (black arrows). The black dotted line shows that the EKG is in perfect sync with the “discharges,” confirming EKG artifact. Note the depressed background activity. Settings: LFF 1 Hz, HFF 70 Hz, 3 µV/mm, time base ~15 seconds/page. 288References 1.Glass HC, Pham TN, Danielsen B, Towner D, Glidden D, Wu YW. Antenatal and intrapartum risk factors for seizures in term newborns: A population-based study, California 1998–2002. J Pediatr. 2009;154:24–28. 2.Saliba RM, Annegers JF, Waller DK, Tyson JE, Mizrahi EM. Incidence of neonatal seizures in Harris County, Texas, 1992–1994. Am J Epidemiol. 1999;150:763–769. 3.Pisani F, Facini C, Bianchi E, Giussani G, Piccolo B, Beghi E. Incidence of neonatal seizures, perinatal risk factors for epilepsy and mortality after neonatal seizures in the province of Parma, Italy. Epilepsia. 2018;59:1764–1773. 4.Pinchefsky EF, Hahn CD. Outcomes following electrographic seizures and electrographic status epilepticus in the pediatric and neonatal ICUs. Curr Opin Neurol. 2017;30:156–164. 5.Glass HC, Shellhaas RA, Wusthoff CJ, et al. Contemporary profile of seizures in neonates: A prospective cohort study. J Pediatr. 2016;174:98–103. 6.Mitra S, Bale G, Mathieson S, et al. Changes in cerebral oxidative metabolism during neonatal seizures following hypoxic-ischemic brain injury. Front Pediatr. 2016;4:83. 7.Younkin, DP, Delivoria-Papadopoulos M, Maris J, Donlon E, Clancy R, Chance B. Cerebral metabolic effects of neonatal seizures measured with in vivo 31P NMR spectroscopy. Ann Neurol. 1986;20:513–519. 8.Glass HC, Glidden D, Jeremy RJ, Barkovich AJ, Ferriero DM, Miller SP. Clinical neonatal seizures are independently associated with outcome in infants at risk for hypoxic-ischemic brain injury. J Pediatr. 2009;155:318–323. 9.Srinivasakumar P, Zempel J, Wallendorf M, Lawrence R, Inder T, Mathur A. Therapeutic hypothermia in neonatal hypoxic ischemic encephalopathy: Electrographic seizures and magnetic resonance imaging evidence of injury. J Pediatr. 2013;163:465–470. 10.Painter MJ, Scher MS, Stein AD, et al. Phenobarbital compared with phenytoin for the treatment of neonatal seizures. New Engl J Med. 1999;34:485–489. 11.Sankar R, Painter MJ. Neonatal seizures: After all these years we still love what doesn’t work. Neurology. 2005;64:776–777. 12.Tsuchida TN, Wusthoff CJ, Shellhaas RA, et al. American Clinical Neurophysiology Society standardized EEG terminology and categorization for the description of continuous EEG monitoring in neonates: Report of the American Clinical Neurophysiology Society Critical Care Monitoring Committee. J Clin Neurophysiol. 2013;30:161–173. 13.Clancy RR, Tharp BR, Enzman D. EEG in premature infants with intraventricular hemorrhage. Neurology. 1984;34:583–590. 14.Cukier F, Andre M, Monod N, Dreyfus-Brisac C. Contribution of EEG to the diagnosis of intraventricular hemorrhages in the premature infant. Rev Electroencephalogr Neurophysiol Clin. 1972;2:318–322. 15.Blume WT, Dreyfus-Brisac C. Positive rolandic sharp waves in neonatal EEG: Types and significance. Electroencephalogr Clin Neurophysiol. 1982;53:277–282. 16.Clancy RR, Tharp BR. Positive rolandic sharp waves in the electroencephalograms of premature neonates with intraventricular hemorrhage. Electroencephalogr Clin Neurophysiol. 1984;57:395–404. 17.Marret S, Parain D, Samson-Dollfus D, Jeannot E, Fessard C. Positive rolandic sharp waves and periventricular leukomalacia in the newborn. Neuropediatrics. 1986;17:199–202. 18.Chung HJ, Clancy RR. Significance of positive temporal sharp waves in the neonatal electroencephalogram. Electroencephalogr Clin Neurophysiol. 1991; 79: 256–263. 19.Scher MS, Bova JM, Dokianakis SG, Steppe DA. Physiological significance of sharp wave transients on EEG recordings of healthy pre-term and full-term neonates. Electroencephalogr Clin Neurophysiol. 1994;90:179–185. 20.Shewmon DA. What is a neonatal seizure? Problems in definition and quantification for investigative and clinical purposes. J Clin Neurophysiol. 1990;7:315–368. 21.Nagarajan L, Palumbo L, Ghosh S. Brief electroencephalography rhythmic discharges (BERDs) in the neonate with seizures: Their significance and prognostic implications. J Child Neurol. 2011;26:1529–1533. 22.Oliveira AJ, Nunes ML, Haertel LM, Reis FM, da Costa JC. Duration of rhythmic EEG patterns in neonates: New evidence for clinical and prognostic significance of brief rhythmic discharges. Clin Neurophysiol. 2000;111:1646–1653. 23.Yoo JY, Rampal N, Petroff OA, Hirsch LJ, Gaspard N. Brief potentially ictal rhythmic discharges in critically ill adults. JAMA Neurol. 2014;71:454–462. 24.Mizrahi EM, Kellaway P. Characterization and classification of neonatal seizures. Neurology. 1987;37:1837–1844. 25.Nagarajan L, Palumbo L, Ghosh S. Classification of clinical semiology in epileptic seizures in neonates. Eur J Paediatr Neurol. 2012;16:118–125. 26.Watanabe K. Neurophysiological aspects of neonatal seizures. Brain Dev. 2014; 36: 363–371. 27.Nunes ML, Yozawitz EG, Zuberi S, et al. Neonatal seizures: Is there a relationship between ictal electroclinical features and etiology? A critical appraisal based on a systematic literature review. Epilepsia Open. 2019;4:10–29. 28.Scher MS, Alvin J, Gaus L, Minnigh B, Painter MJ. Uncoupling of EEG-clinical neonatal seizures after antiepileptic drug use. Pediatr Neurol. 2003;28:277–280. 29.Weiner SP, Painter MJ, Geva D, Guthrie RD, Scher MS. Neonatal seizures: Electroclincal dissociation. Pediatr Neurol. 1991;7:363–368. 30.Murray DM, Boylan GB, Ali I, Ryan CA, Murphy BP, Connolly S. Defining the gap between electrographic seizure burden, clinical expression and staff recognition of neonatal seizures. Arch Dis Child Fetal Neonatal Ed. 2008;93:F187–F191. 31.Shellhaas RA, Chang T, Tsuchida T, et al. The American Clinical Neurophysiology Society’s guideline on continuous electroencephalography monitoring in neonates. J Clin Neurophysiol. 2011;28:611–617. 32.Bye AM, Flanagan D. Spatial and temporal characteristics of neonatal seizures. Epilepsia. 1995;36:1009–1016. 33.Janackova S, Boyd S, Yozawitz E, et al. Electroencephalographic characteristics of epileptic seizures in preterm neonates. Clin Neurophysiol. 2016;127:2721–2727. 34.Patrizi S, Holmes GL, Orzalesi M, Allemand F. Neonatal seizures: Characteristics of EEG ictal activity in preterm and fullterm infants. Brain Dev. 2003;25:427–437. 35.Shellhaas RA, Clancy RR. Characterization of neonatal seizures by conventional EEG and single-channel EEG. Clin Neurophysiol. 2007;118:2156–2161. 36.Lloyd RO, O’Toole JM, Pavlidis E, Filan PM, Boylan GB. Electrographic seizures during the early postnatal period in preterm infants. J Pediatr. 2017;187:18–25. 37.Scher MS, Hamid MY, Steppe DA, Beggarly ME, Painter MJ. Ictal and interictal electrographic seizure durations in preterm and term neonates. Epilepsia. 1993; 34:284–288. 38.Glass HC, Shellhaas RA, Tsuchida TN, et al. Seizures in preterm neonates: A multicenter observational cohort study. Pediatr Neurol. 2017;72:19–24. 39.Boylan GB, Kharoshankaya L, Wusthoff CJ. Seizures and hypothermia: Importance of electroencephalographic monitoring and considerations for treatment. Semin Fetal Neonatal Med. 2015;20:103–108. 40.Low E, Boylan GB, Mathieson SR, et al. Cooling and seizure burden in term neonates: An observational study. Arch Dis Child Fetal Neonatal Ed. 2012;97:F267–F272. 41.Golomb MR, Garg BP, Edwards-Brown M, Williams LS. Very early arterial ischemic stroke in premature infants. Pediatr Neurol. 2008;38:329–334. 42.Lehman LL, Rivkin MJ. Perinatal arterial ischemic stroke: Presentation, risk factors, evaluation, and outcome. Pediatr Neurol. 2014;51:760–768. 43.Shah NA, Wusthoff CJ. Intracranial hemorrhage in the neonate. Neonatal Netw. 2016;35:67–71. 44.Vesoulis ZA, Inder TE, Woodward LJ, Buse B, Vavasseur C, Mathur AM. Early electrographic seizures, brain injury, and neurodevelopmental risk in the very preterm infant. Pediatr Res. 2014;75:564–569. 45.Al Yazidi G, Boudes E, Tan X, Saint-Martin C, Shevell M, Wintermark P. Intraventricular hemorrhage in asphyxiated newborns treated with hypothermia: A look into incidence, timing and risk factors. BMC Pediatr. 2015;15:106. 46.Nash KB, Bonifacio SL, Glass HC, et al. Video-EEG monitoring in newborns with hypoxic-ischemic encephalopathy treated with hypothermia. Neurology. 2011;76:556–562. 47.Wusthoff CJ, Dlugos DJ, Gutierrez-Colina A, et al. Electrographic seizures during therapeutic hypothermia for neonatal hypoxic-ischemic encephalopathy. J Child Neurol. 2011;26:724–728. 48.Glass HC, Wusthoff CJ, Shellhaas RA, et al. Risk factors for EEG seizures in neonates treated with hypothermia: A multicenter cohort study. Neurology. 2014; 82:1239–1244. 49.Glass HC, Numis AL, Gano D, Bali V, Rogers EE. Outcomes after acute symptomatic seizures in children admitted to a neonatal neurocritical care service. Pediatr Neurol. 2018;84:39–45. 50.Liu X, Jary S, Cowan F, Thoresen M. Reduced infancy and childhood epilepsy following hypothermia-treated neonatal encephalopathy. Epilepsia. 2017;58:1902–1911. 51.Trinka E, Cock H, Hesdorffer D, et al. A definition and classification of status epilepticus—Report of the ILAE Task Force on Classification of Status Epilepticus. Epilepsia. 2015;56:1515–1523. 52.Abend NS, Wusthoff CJ. Neonatal seizures and status epilepticus. J Clin Neurophysiol. 2012;29:441–448. 53.Wagenman KL, Blake TP, Sanchez SM, et al. Electrographic status epilepticus and long-term outcome in critically ill children. Neurology. 2014;82:396–404. 54.Glass HC, Hong KJ, Rogers EE, et al. Risk factors for epilepsy in children with neonatal encephalopathy. Pediatr Res. 2011;70:535–540. 55.Pavlidis E, Spagnoli C, Pelosi A, Mazzotta S, Pisani F. Neonatal status epilepticus: Differences between preterm and term newborns. Eur J Paediatr Neurol. 2015; 19:314–319. 56.Kharoshankaya L, Stevenson NJ, Livingstone V, et al. Seizure burden and neurodevelopmental outcome in neonates with hypoxic–ischemic encephalopathy. Dev Med Child Neurol. 2016;58:1242–1248. 57.Hirsch LJ, LaRoche SM, Gaspard N, et al. American Clinical Neurophysiology Society’s Standardized Critical Care EEG Terminology: 2012 version. J Clin Neurophysiol. 2013;30:1–27. 58.Scher MS, Beggarly M. Clinical significance of focal periodic discharges in neonates. J Child Neurol. 1989;4:175–185. 59.Mikati MA, Feraru E, Krishnamoorthy K, Lombroso CT. Neonatal herpes simplex meningoencephalitis: EEG investigations and clinical correlates. Neurology. 1990;40:1433–1437.
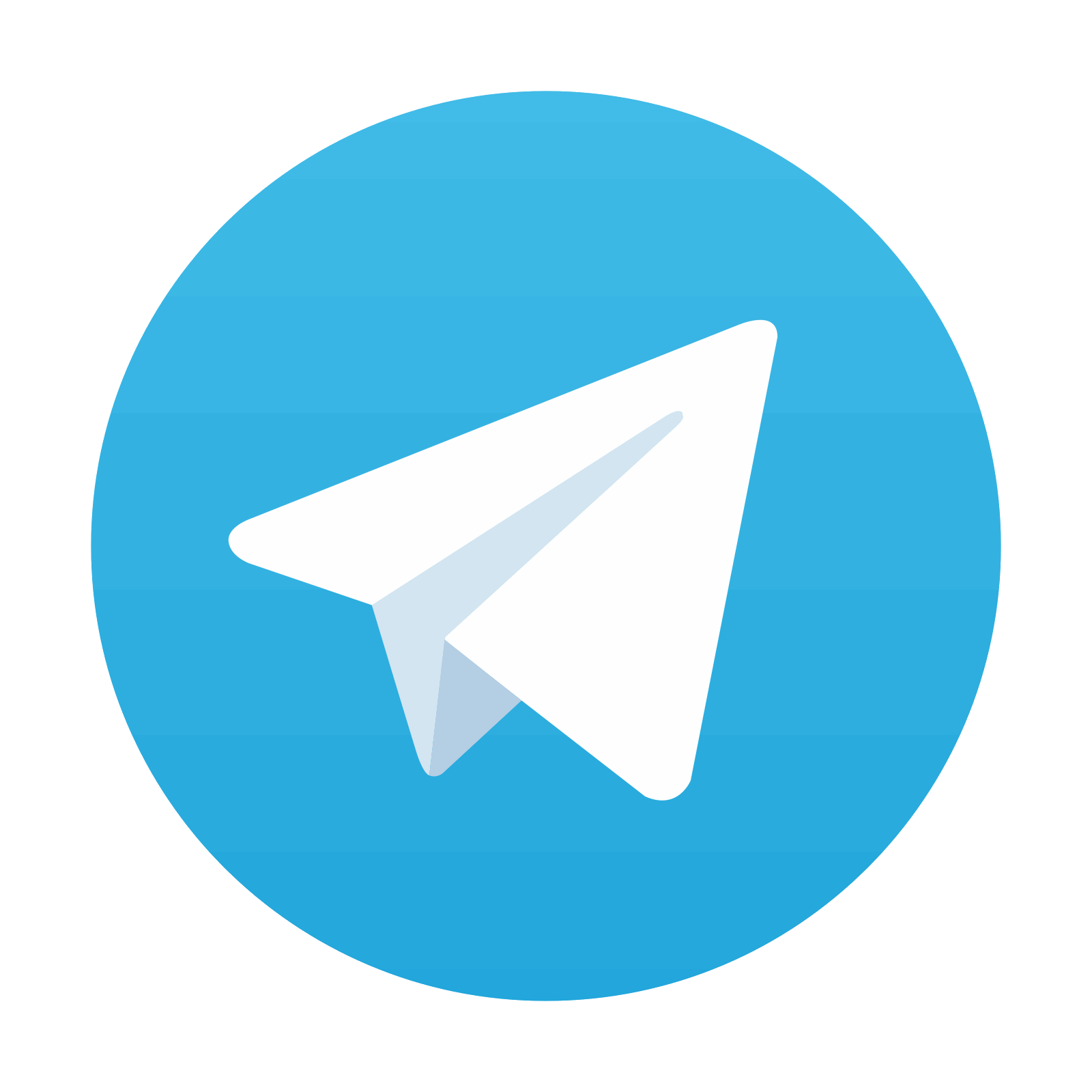
Stay updated, free articles. Join our Telegram channel

Full access? Get Clinical Tree
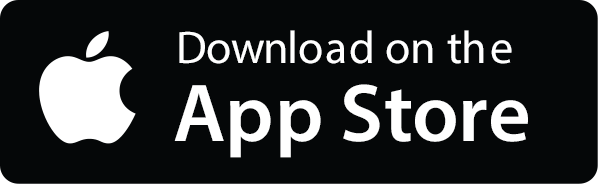
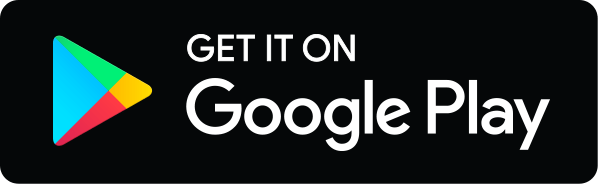