Keywords
neurocardiogenic, cardiac complications, cardiac innervation, neurologic injury, stroke, epilepsy, trauma
Cardiac abnormalities are common after acute neurologic injury. Disturbances can range in severity from transient electrocardiographic (ECG) abnormalities to profound myocardial injury and dysfunction. Evidence from animal models and clinical observations indicate that the central nervous system (CNS) is involved in the generation of cardiac arrhythmias and dysfunction even in an otherwise normal myocardium. Neurologic lesions may influence cardiovascular function and affect cardiac prognosis, and—in addition—the presence of cardiac abnormalities may be associated with poor neurologic outcomes. A better understanding of cardiac abnormalities after acute neurologic injury can improve the clinical management of patients and may also have important prognostic implications.
This chapter briefly outlines the cardiac manifestations that follow acute neurologic injury, summarizes the neurophysiology and neuroanatomy of cardiac control, and discusses the clinical implications and diagnostic and treatment recommendations for the most common cardiac complications.
Historical Perspective
Cushing first described hemodynamic changes after acute intracerebral hemorrhage in 1903. The bradycardia and hypertension in response to increased intracranial pressure (ICP), known as the “Cushing reflex,” was later proved in animal models to be mediated by the CNS. Over subsequent decades, clinical observations began to identify the importance of the brain–heart interaction in patients with cerebral lesions. Cardiac abnormalities were described with various CNS diseases including seizures, trauma, ischemic stroke, and intracerebral hemorrhage (ICH), and less commonly with tumors, electroconvulsive therapy, and meningitis. Cardiac pathology with features of subendocardial hemorrhage was described in these neurologic patients without known previous cardiac disease. After World War II, patients with subarachnoid hemorrhage (SAH) were noted to have cardiac myocytolysis similar to that in pheochromocytoma. An emotional- and stress-induced cardiomyopathy was then described in Japan, and subsequently reported in other populations.
Anatomy and Physiology
The anatomy and physiology of the pathways involved in brain–heart interactions have been elucidated in both animal and human studies. The medulla has been described as the principal site of vagal parasympathetic and sympathetic areas involved in cardiac control. In addition, both anatomic and physiologic evidence exists to implicate the hypothalamus in cardiac control. Electrical stimulation experiments suggest a posteriorly located area of cardiovascular sympathetic control and an anterior parasympathetic control region. Beattie and colleagues first described cardiac arrhythmias after hypothalamic stimulation. Arrhythmias from hypothalamic stimulation were subsequently confirmed in other animal models.
Areas of the cerebral cortex with connections to the autonomic nervous system can also elicit cardiac responses. The autonomic–emotional interactions with cardiovascular function have been linked to the central nucleus of the amygdala. Stimulation of the orbitofrontal, cingulate, and temporal lobes may elicit a cardiac response, but with much less frequency than the hypothalamus and brainstem. The central autonomic network has been investigated further with modern neuroimaging. The majority of evidence suggests that the insular cortex has a pivotal role in integrating autonomic responses involved in the regulation of the cardiovascular system and is strongly associated with adverse cardiac events after neurologic injury.
The Insular Cortex
The insular cortex has widespread connectivity with other areas of the brain that are known to be involved in autonomic control. Both experimental and clinical evidence strongly suggest a role for the insula in cardiovascular function. In microstimulation experiments in rats, Oppenheimer and colleagues first identified the insular cortex as a site from which lethal cardiac arrhythmias and myocardial damage could be produced, resembling changes seen in patients after stroke and in association with sudden death in patients with epilepsy.
Animal models of stroke have provided further evidence for involvement of the insula in the autonomic regulation of the heart. In a rat model of cerebral ischemia, insular infarction was associated with increased renal sympathetic nerve activity, prolongation of the QT interval, and elevated norepinephrine levels. Pathologically, cardiac myocytolysis was demonstrated only if the insular cortex was involved. This evidence collectively supports the belief that stroke can alter cardiovascular tone by directly damaging the insular cortex or other interrelated areas and shifting the balance toward a predominance of sympathetic activation.
Although there is considerable evidence suggesting lateralization of cardiac control in animal models, the clinical impact of the laterality of insular strokes in humans has proved to be more complicated. In patients undergoing temporal lobectomy for the control of intractable seizures, stimulation of either insula resulted in alterations of blood pressure and heart rate, but bradycardia and decreased blood pressure occurred with greater frequency from the left anterior insular cortex. Other observations during infusion of amyl barbital during the Wada test are of tachycardia after left carotid infusion and bradycardia with right internal carotid infusion. Collectively, these observations support a greater sympathetic cardiovascular representation on the right and greater parasympathetic regulation on the left.
Observational stroke studies in humans have provided conflicting results without a predominant laterality related to prognosis and outcomes. In an observational study of 62 acute stroke patients, an increased incidence of sudden death occurred among patients with right insular strokes. In addition, right middle cerebral artery strokes were associated with a significantly increased incidence of supraventricular tachyarrhythmias. When anterior left insular lesions were specifically isolated, there was increased cardiac sympathetic and decreased cardiac parasympathetic tone. In contrast, left parietoinsular stroke was associated with an increased incidence of new-onset atrial fibrillation, often considered a parasympathetically derived abnormality. In a prospective study of patients with stroke and transient ischemic attacks (TIAs), left insular strokes were an independent risk factor for adverse cardiac outcome in patients without heart disease. In the Northern Manhattan Study, left parietal lobe infarction was an independent predictor of long-term cardiac death and myocardial infarction. Similarly, in a secondary analysis of the North American Symptomatic Endarterectomy Trial, long-term risk of sudden death was significantly increased in patients with left brain infarction. These observations highlight the complex interactions of the insular cortex and other autonomic centers of the brain.
Ischemic and Hemorrhagic Stroke
Clinical observations in patients with stroke have greatly advanced the understanding of interactions between the brain and heart. Cardiac abnormalities occur in a majority of patients after stroke, and can range from transient ECG findings to serious cardiac events and cardiac death. The most common disturbances include ECG abnormalities, cardiac arrhythmias, and myocardial injury and dysfunction. Distinguishing cardiac abnormalities caused directly by stroke, however, remains difficult because the prevalence of preexisting cardiac disease is high, particularly among patients with ischemic stroke. However, substantial evidence exists supporting the occurrence of cardiac disturbances after stroke even in the absence of significant coronary artery disease (CAD). Increasing evidence suggests that the presence of stroke should be a deemed a risk-factor equivalent contributing to absolute risk estimates for outcomes and prevention of vascular disease. A meta-analysis of patients with acute stroke followed for a mean of 3.5 years revealed an annual risk of myocardial infarction of 2 percent. Cardiac disturbances are the most common cause of death after stroke, accounting for up to 6 percent of unexpected deaths during the first month. Understanding the mechanisms of cardiac disturbances may prevent future cardiac complications and improve survival in these patients.
The Electrocardiogram
ECG abnormalities are common, presenting in the majority of patients with acute stroke. In 1947, Byer and colleagues first described marked QT prolongation with large T and U waves in the ECG of four patients with acute stroke. Subsequently, Burch and colleagues described an ECG pattern after acute stroke consisting of large inverted T waves, prolonged QT intervals, and large septal U waves that has become distinctive of cerebrovascular injury ( Fig. 10-1 ). In the 17 abnormal ECGs reported in their study, the abnormalities were most frequently observed after SAH, followed by ICH and ischemic lesions. These early studies did not control for the incidence of concomitant or preexisting ischemic cardiac disease, but subsequent case-control studies suggested that repolarization abnormalities on ECGs are not present prior to the stroke event. Overall, there was a significantly increased incidence of ST depression, prolongation of the QT interval, T-wave inversion, and ventricular premature beats among stroke patients compared to age- and sex-matched controls. Although patients with known cardiac disease were excluded, limitations of these studies were the lack of detailed cardiac evaluations and comparison to antecedent ECG tracings that may not have completely excluded coexistent heart disease.

QT Prolongation
The most common stroke-related ECG abnormality is QT prolongation, a myocardial repolarization abnormality associated with an increased risk of a characteristic life-threatening cardiac arrhythmia, known as torsade de pointes ( Fig. 10-2 ). Interestingly, congenital forms of long QT may be related to imbalance of sympathetic innervation of the heart. Among acute stroke patients, prolonged QT interval is more frequently observed after hemorrhagic strokes, occurring in 45 to 71 percent of patients with SAH or ICH compared to 38 percent of those with ischemic strokes. Ventricular tachyarrhythmias including sudden death and torsade de pointes are often preceded by QT prolongation in patients with SAH. QT prolongation, accompanied by U waves and T-wave changes, often correlates with elevated systolic blood pressure on admission. Assessment of common causes of these ECG changes, such as hypokalemia, hypomagnesemia, and medication toxicity, is recommended before attributing them to the underlying stroke.

Repolarization Abnormalities
The similarities between ECG changes due to acute myocardial ischemia and infarction and those associated with stroke are most striking with the repolarization abnormalities involving the ST segment, leading many investigators to hypothesize coexisting cardiac disease as the primary cause. ST segment changes (including ST elevations) occur in 22 to 35 percent of patients with ischemic stroke, but interpretation of such findings is complicated by the increased prevalence of cardiac disease in this subgroup of stroke patients. However, new T-wave abnormalities appear in approximately 15 percent of patients with acute stroke, even in the absence of electrolyte disturbances or primary ischemic heart disease. Inverted or flat T waves have also been reported in up to 55 percent of patients with SAH, the stroke subgroup with the lowest prevalence of coexistent cardiac disease. Kono and colleagues performed detailed cardiac assessments on 12 patients with acute SAH and ST elevations on ECG. Although patients were found to have apical wall motion abnormalities on echocardiogram, there was no evidence of coronary artery stenosis or vasospasm on cardiac angiography. These findings, along with the observation that stroke-induced ECG changes are evanescent, resolving over a period of days to months with little residuum, argue against myocardial ischemia or infarction as the only cause of repolarization changes on ECG.
Q Waves and U Waves
New Q waves similar in morphology to those observed in acute myocardial infarction are also common after acute stroke, reported in approximately 10 percent of patients with acute ischemic or hemorrhagic stroke. To complicate matters, Q waves may be transient or proceed through the evolutionary changes seen in myocardial infarction. Further cardiac evaluation may be necessary in patients with Q waves and ST segment alterations, particularly if they are over 65 years of age with coronary risk factors such as diabetes mellitus.
New U waves occur in isolation or with T waves and QT abnormalities in approximately 13 to 15 percent of patients with acute ischemic stroke and SAH. Isolated U waves were equally distributed between ischemic and hemorrhagic strokes, but the combination of U waves and QT prolongation was more common among patients with hemorrhagic strokes. There is no relationship between the presence of U waves and stroke mortality, suggesting that this ECG change should not require any specific treatment or evaluation.
Cardiac Arrhythmias
Nearly every type of cardiac arrhythmia has been reported after acute stroke, including bradycardia, supraventricular tachycardia, atrial flutter, atrial fibrillation, ectopic ventricular beats, multifocal ventricular tachycardias, torsade de pointes, ventricular flutter, and ventricular fibrillation. Most arrhythmias occur within the first week after stroke, occurring in 25 to 40 percent of patients with ischemic stroke or ICH, and 98 percent of patients with SAH. In a prospective study, the incidence of serious arrhythmia was highest in the first 24 hours after hospital admission, and clinically symptomatic in 25 percent of events. Atrial fibrillation was the most common cardiac arrhythmia, accounting for 60 percent of the events. In these studies, which did not control for preexisting cardiac disease, rhythm disturbances may have preceded the stroke event and, at least for atrial fibrillation, may have been causally related to the ischemic stroke. Not surprisingly, since the ECG is a relatively insensitive test for arrhythmia, a higher incidence of ventricular extrasystoles, followed by atrial extrasystoles, supraventricular tachycardia, and atrial fibrillation was found in a prospective study of ischemic stroke patients using cardiac telemetry monitoring. Importantly, the presence of arrhythmias after stroke is significantly associated with increased mortality.
In studies of hemorrhagic strokes, the incidence of ventricular arrhythmias was 10 percent after ICH. Location of hemorrhage appears to correlate with the rhythm disturbances. Yamour and colleagues reported a correlation between the occurrence of brainstem bleeds and atrial fibrillation. Ventricular arrhythmias correlated with temporoparietal location, whereas sinus bradycardia and supraventricular tachycardias were seen more commonly with traumatic frontal lobe hemorrhage.
Patients with SAH have even more profound rhythm disturbances that may be related to the diffuse nature of the injury and the degree of monitoring in the intensive care unit. Stober and colleagues described multifocal ventricular ectopy (54%), asystolic intervals (27%), sinus bradycardia (23%), and atrial fibrillation (4%). Because the frequency and severity of arrhythmias are significantly higher in patients studied within 48 hours of onset of SAH, we recommend continuous cardiac monitoring in an intensive care setting for all patient with acute SAH.
Cardiac Injury and Dysfunction
Myocardial infarction in the setting of acute stroke is not uncommon, and often represents concomitant CAD in older patients with ischemic stroke and vascular risk factors. However, evidence from autopsy series in both ischemic and hemorrhagic stroke indicates that cardiac dysfunction may occur in the absence of underlying CAD. When myocardial tissue injury is present, suspicion for underlying cardiac disease increases, but pathologic and functional studies of the heart suggest a mechanism distinct from coronary artery–induced ischemia. Subendocardial hemorrhages were initially described in patients dying after acute strokes and seizures. Further studies suggested that these pathologic changes are secondary to excessive sympathetic stimulation. Following intracranial hemorrhage, catecholamines increase in cardiac tissue. Catecholamine-induced subendocardial lesions include scattered foci of swollen myocytes surrounded by infiltrating monocytes, interstitial hemorrhages, and myofibrillar degeneration. Collectively, the characteristic pathologic changes have been called contraction band necrosis, coagulative myocytolysis, or myofibrillar degeneration ( Fig. 10-3 ). The pattern of myofibrillar necrosis localizing near cardiac nerves is identical to other lesions thought to be of sympathetic origin such as catecholamine infusion, “voodoo death,” hypothalamic stimulation, or reperfusion of transiently ischemic cardiac muscle. The Takotsubo or “broken heart” syndrome is a severe cardiomyopathy associated with such major life events or stresses as the death of a loved one or intense fear. There is no evidence of CAD and survivors may recover left ventricular function completely over the following 1 to 2 weeks.

In patients with CAD, myocardial necrosis typically follows a vascular distribution. The timing of the injury is also distinct from the necrosis seen in CAD, which typically occurs in a delayed fashion after progressive ischemia and muscle cell death. Neurogenic myocardial injury can be visible within minutes of onset, with appreciable differences observed on a cellular level. In myocytolysis, mononuclear infiltration predominates, with early calcification and myocardial cells in a hypercontracted state with contraction bands.
Further evidence for a neurogenic mechanism of cardiac injury comes from studies of cardiac function after SAH, which typically affects younger patients without a history of coexistent cardiac disease. Global or regional left ventricular systolic dysfunction on echocardiogram has been described after SAH with an approximate incidence of 10 to 28 percent. The severity of neurologic injury is strongly associated with the presence of left ventricular dysfunction. Similarly, diastolic dysfunction is also common after SAH, is associated with the severity of neurologic injury, and may be the cause of pulmonary edema in these patients. The onset of left ventricular dysfunction occurs early in the course of SAH. In the largest study to date, a regional wall motion abnormality was most likely to be present within the first 2 days. The prevalence then declined during days 3 to 8 after hemorrhage. In this same study, the authors demonstrated complete or partial resolution of left ventricular dysfunction in the majority of patients during their acute hospitalization. Thus, cardiac dysfunction appears to be reversible in most cases and normalizes over time.
There is a well-demonstrated, unique, apical-sparing pattern of regional wall motion abnormality that differentiates SAH patients from those with the typical patterns seen in CAD. A retrospective study of patients with SAH demonstrated reversibility and both global and regional left ventricular dysfunction, most commonly affecting the anterior and anteroseptal walls and not involving the apex. Younger age and anterior aneurysm position were independent predictors of this pattern. This apical-sparing pattern of left ventricular dysfunction argues against an obstruction or vasospasm of coronary arteries and provides indirect evidence of a neurally mediated mechanism of injury.
Experimental and clinical studies have addressed a neurogenic catecholamine-mediated mechanism (“catecholamine hypothesis”) of cardiac dysfunction. In a cohort of patients with SAH who had echocardiograms and nuclear scans of cardiac innervation and perfusion, regions of contractile dysfunction were associated with abnormalities in myocardial sympathetic innervation while cardiac perfusion was normal. Degree of cardiac innervation was measured with a scintigraphic evaluation using [ 123 I]metaiodobenzylguanidine (MIBG), cardiac perfusion was measured using [ 99m Tc]sesta-methoxyisobutylisonitrile ( MIBI), and regions of myocardial dysfunction were determined by echocardiography simultaneously in the patients. Patients with functional cardiac denervation had worse regional wall motion scores (RWMS) and more troponin release than patients without evidence of cardiac denervation ( Table 10-1 ). Figure 10-4 illustrates normal perfusion and global denervation in a patient with SAH whose echocardiogram showed global left ventricular systolic dysfunction. All study subjects had normal perfusion imaging, which excluded significant coronary artery disease and supported a neurogenic mechanism of cardiac injury. In addition, data suggest that genetic polymorphisms of the adrenoceptors are associated with an increased risk of cardiac abnormalities after SAH. These data support the hypothesis that cardiac dysfunction after SAH is a form of neurocardiogenic injury.
(H:M)>1.57 ( n =19) | (H:M)<1.57 ( n =18) | P | |
---|---|---|---|
LVEF<50% | 32% | 61% | 0.072 * |
Mean LVEF | 53±15 | 51±16 | 0.61 † |
RWMS>1.0 | 47% | 83% | 0.038 ‡ |
Mean RWMS | 1.3±0.5 | 1.5±0.5 | 0.071 † |
cTi>1.0 | 16% | 50% | 0.038 ‡ |
Mean cTi | 2.9±10.2 | 5.3±11.8 | 0.15 † |

Plasma Markers
In addition to pathologic data and measurements of cardiac function, elevations in cardiac enzymes provide evidence of myocardial injury after stroke. Creatine kinase (CK) and specifically the cardiac isoenzyme CK-MB are released from damaged myocardium. Elevated serum levels occur in 10 to 45 percent of stroke patients, and there is a good correlation between elevation in CK-MB and stroke-induced ECG changes or cardiac arrhythmias. Unlike acute myocardial infarction, a stroke-induced increase in serum CK-MB levels occurs more slowly and peaks at a much lower value on around day 4 after stroke.
Cardiac troponin I is a specific and more sensitive marker of myocardial damage. Elevations in troponin I have been described in 20 to 25 percent of patients with SAH. The degree of neurologic injury and female gender are strong predictors of myocardial necrosis after SAH ( Table 10-2 ). Acute troponin I elevation after SAH is associated with ECG abnormalities, left ventricular dysfunction, pulmonary edema, hypotension, and delayed cerebral ischemia from vasospasm. In a meta-analysis of measurements of cardiac complications after SAH, markers for cardiac damage and dysfunction were associated with increased mortality, delayed cerebral ischemia, and poor functional outcome. Among SAH survivors, there is an increased long-term mortality associated with SAH-related cardiac injury, providing more evidence for the role of cardiac injury in adverse outcomes.
Predictors | Univariate | Multivariate | ||||
---|---|---|---|---|---|---|
OR | P | 95% CI | OR | P | 95% CI | |
Age (per 10 year increase) | 1.16 | 0.262 | 0.90–1.49 | 1.54 | 0.112 | 0.90–2.62 |
Female (vs. male) | 2.98 | 0.019 | 1.20–7.43 | 34.96 | 0.009 | 2.47–495.01 |
Body surface area (per 0.2 m 2 increase) | 1.03 | 0.847 | 0.78–1.36 | 2.20 | 0.025 | 1.11–4.39 |
Hunt and Hess score>2 (vs. 1–2) | 8.47 | <0.001 | 3.43–20.92 | 6.62 | 0.026 | 1.25–34.82 |
Systolic blood pressure (per 20 mmHg increase) | 0.78 | 0.019 | 0.64–0.96 | 0.52 | 0.007 | 0.32–0.83 |
Heart rate (per 10 bpm increase) | 1.26 | 0.001 | 1.10–1.43 | 1.61 | 0.005 | 1.16–2.25 |
Phenylephrine dose (per 50 μg/min increase) | 1.17 | <0.001 | 1.07–1.28 | 1.47 | 0.010 | 1.10–1.98 |
LVMI (per 20 g/m 2 increase) | 1.23 | 0.083 | 0.97–1.55 | 1.74 | 0.032 | 1.05–2.89 |
SAH to cTi * (per 1 day increase) | 0.86 | 0.001 | 0.78–0.94 | 0.70 | 0.008 | 0.54–0.91 |
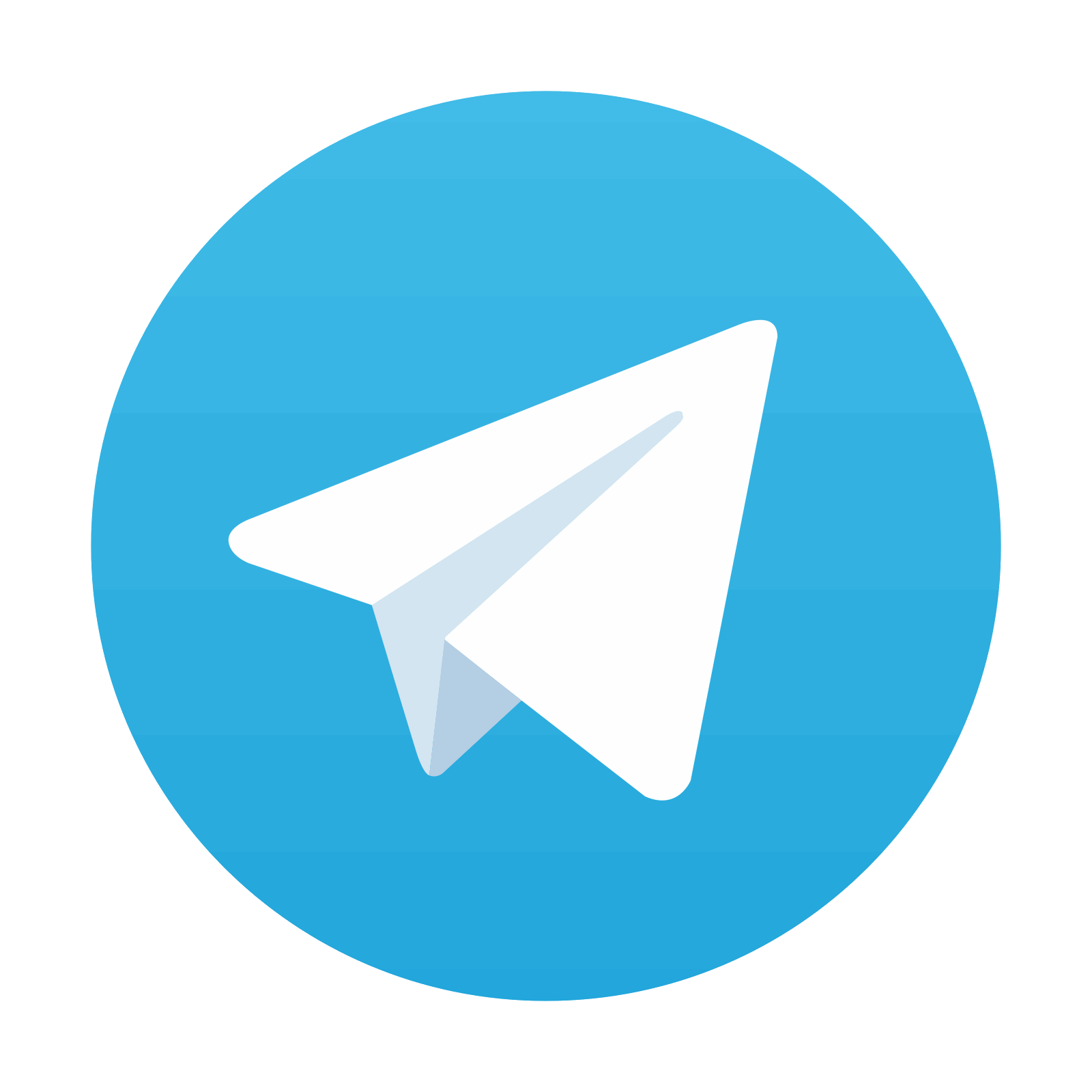
Stay updated, free articles. Join our Telegram channel

Full access? Get Clinical Tree
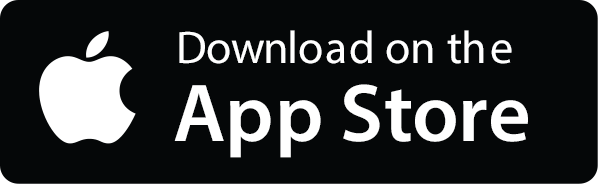
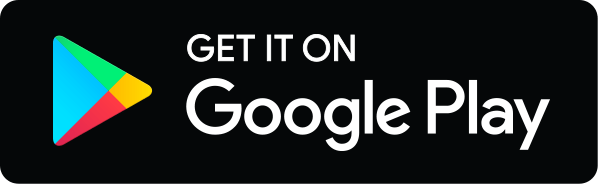
